Access Journal Content
Open access browsing of table of contents and abstract pages. Full text pdfs available for download for subscribers.
Current Issue: Vol. 30 (3)
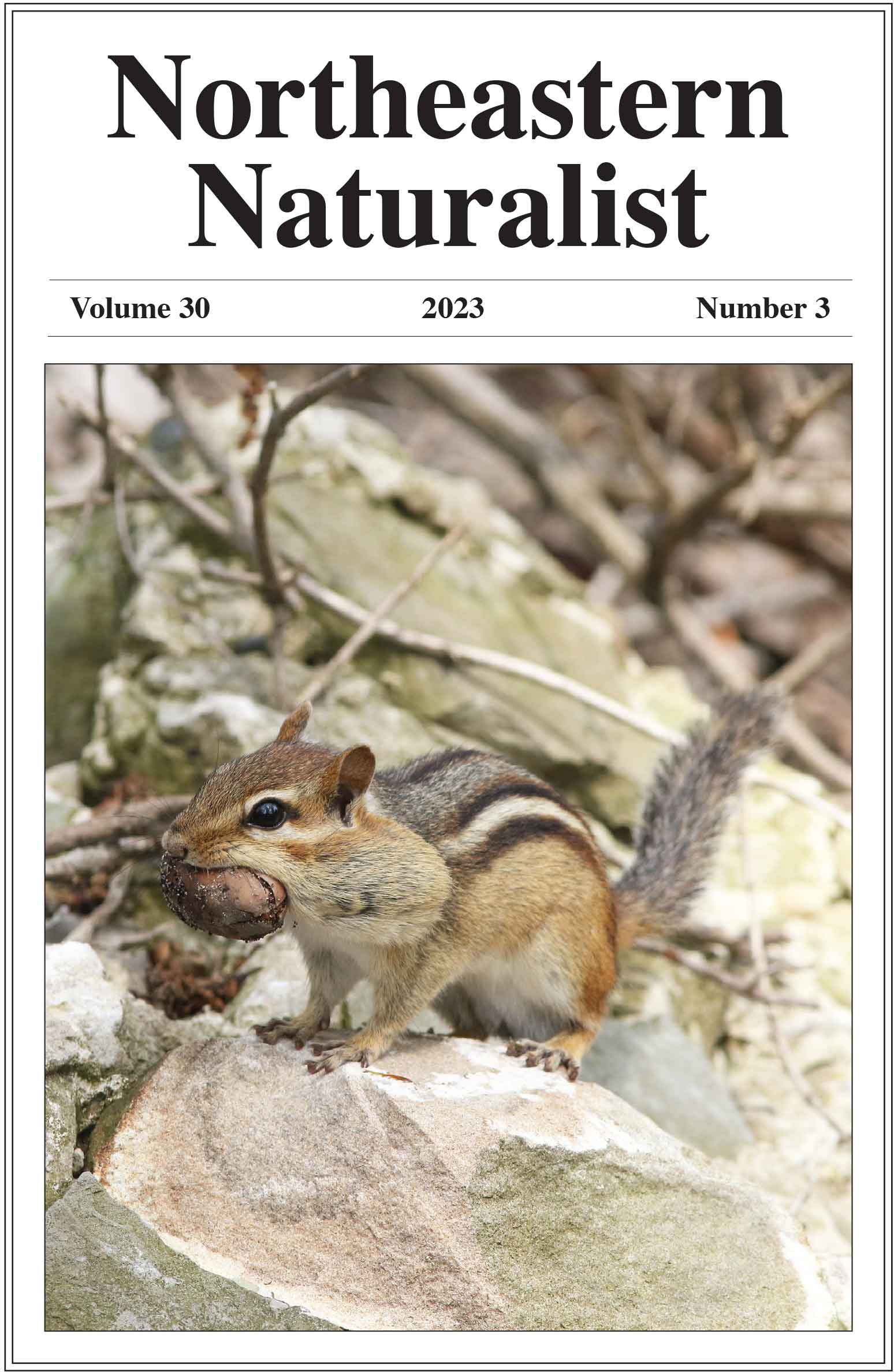
Check out NENA's latest Monograph:
Monograph 22
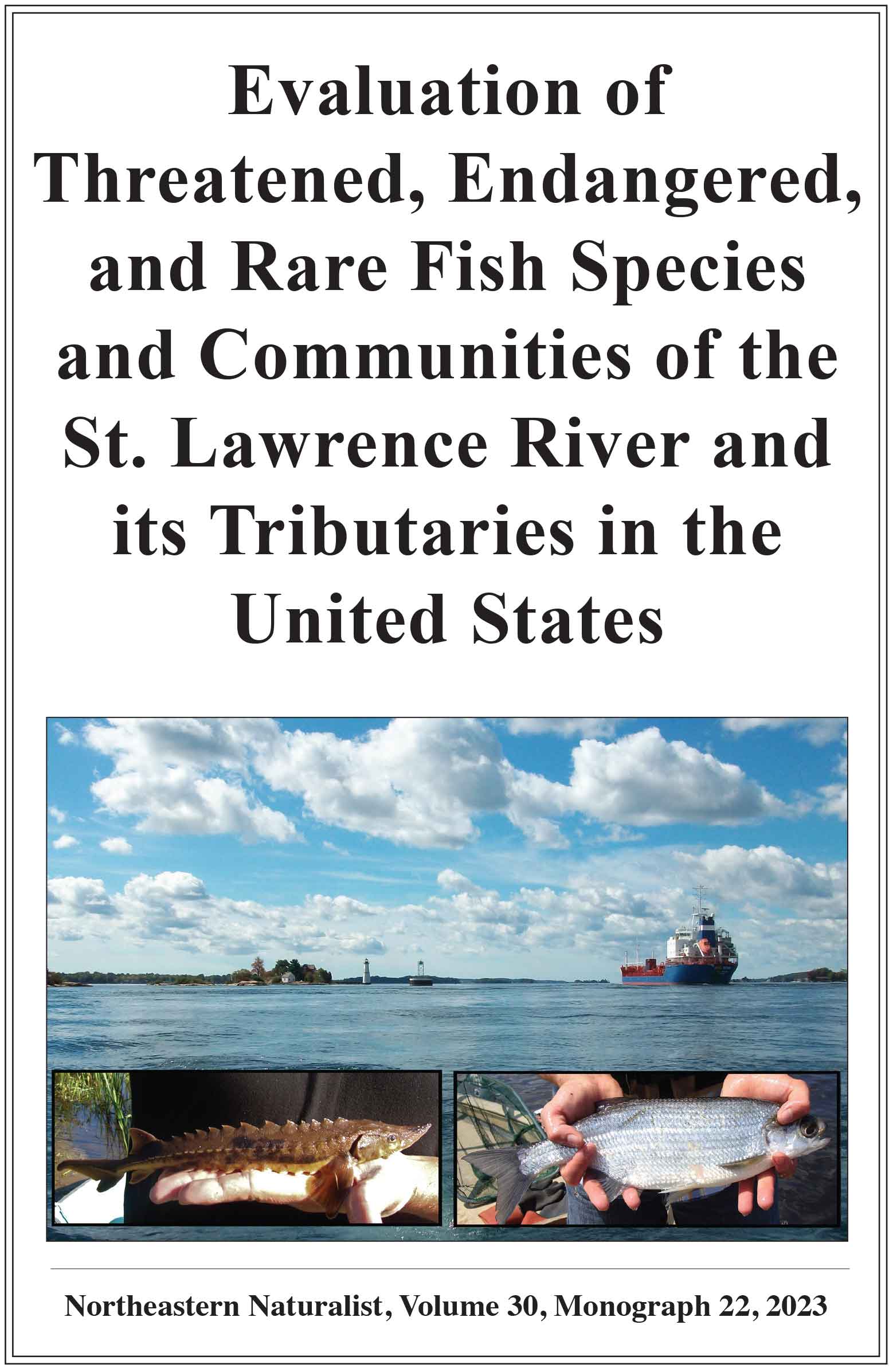
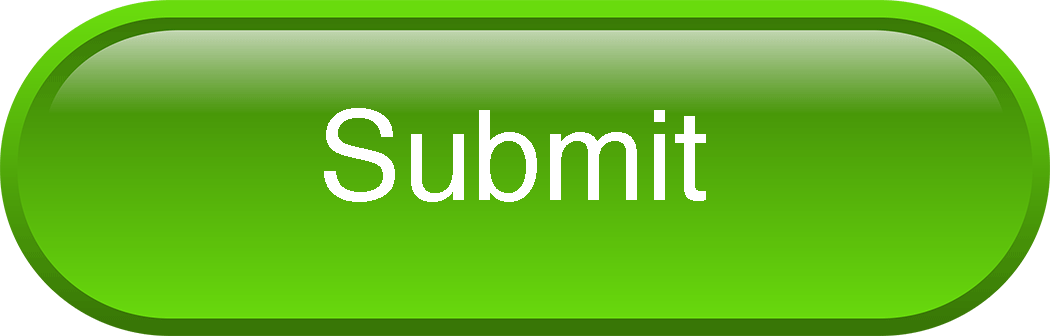
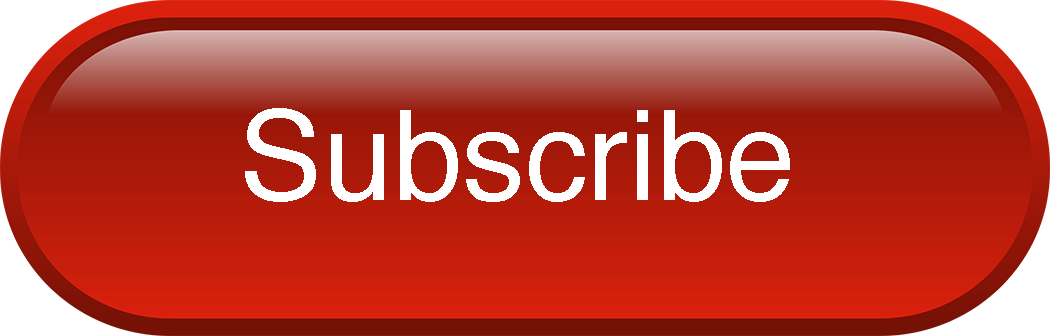
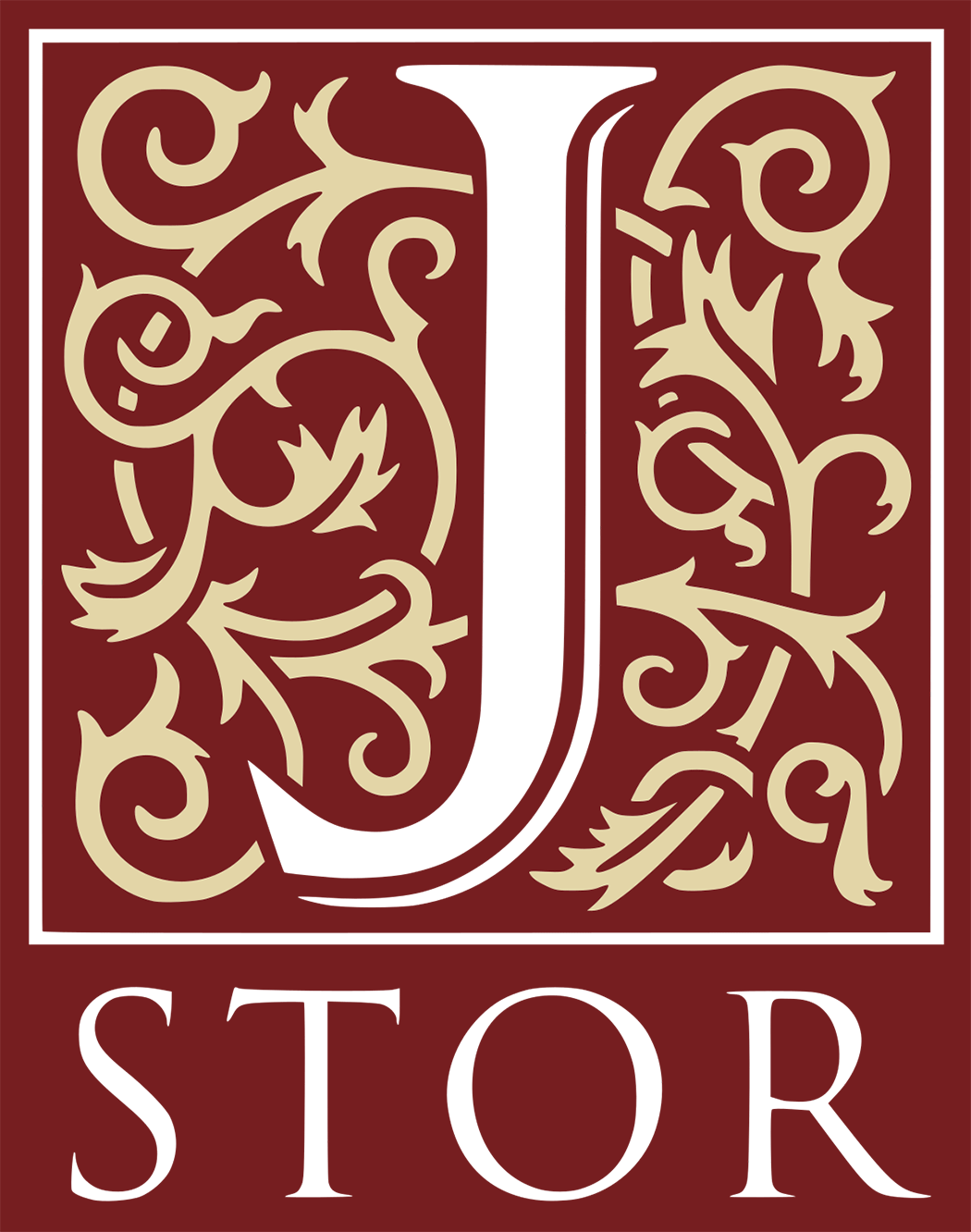


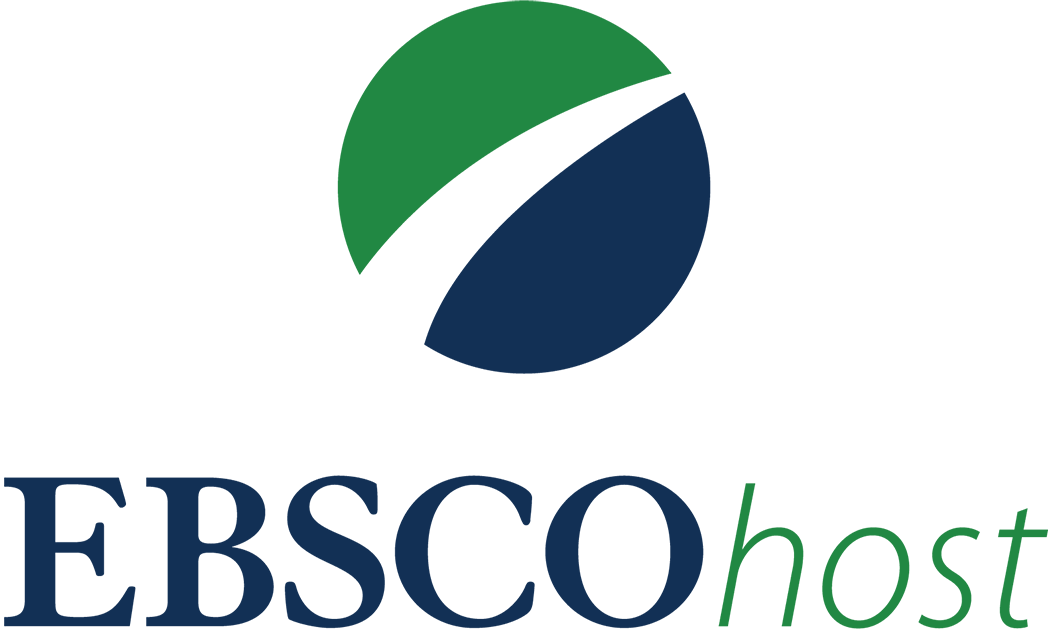

2007 NORTHEASTERN NATURALIST 14(3):461–470
Top-down Effect of Fish Predation in Virginia
Headwater Streams
Elise Wach1 and Randolph M. Chambers2,*
Abstract - We completed laboratory-feeding studies that demonstrated preference of
the headwater stream fishes Gambusia holbrooki (Mosquitofish) and Clinostomus
funduloides (Rosyside Dace) for smaller Gammarus pseudolimnaeus (amphipods)
over larger ones. We also submerged oak leaf-litter bags in sections of streams with
and without these fish predators. After three weeks, the mean number ± s.e. of
amphipods per litter bag in streams with fish was significantly lower (289 ± 50 vs.
978 ± 122) and the average size of amphipods was significantly larger (13.9 ± 0.1 mg
vs. 8.1 ± 0.1 mg), relative to streams without fish. The quantity and quality of leaf
litter, however, were not significantly different. Top-down predation may have
altered the population structure of stream-dwelling amphipods, but did not change
leaf decomposition over the time of the study.
Introduction
Headwater stream ecosystems are predominantly heterotrophic (Fisher
and Likens 1972), where food-web dynamics and maintenance of community
structure are dependent upon the input of organic matter—primarily as
leaf litter—from adjacent terrestrial environments (Cummins et al. 1972,
Short and Maslin 1977, Wallace et al. 1997, Webster et al. 1995). In these
detritus-based streams, factors that control the decomposition of
allochthonous organic matter determine the transfer of energy and nutrients
to higher trophic levels (Creed and Reed 2004, Hall et al. 2000, Wallace et
al. 1999). For example, the action of invertebrate “shredder” organisms that
break down leaf litter into smaller physical pieces is dependent on litter
quality (Motomori et al. 2001) and can account for 25% of the loss of leaf
mass (Cuffney et al. 1990, Newman 1990, Peterson and Cummins 1974).
Shredder feces and small leaf fragments are rich substrates for microbes and
constitute a high-quality food source for filter-feeding organisms that remove
these particles from the water column (Cummins et al. 1972). Shredder
biomass, in turn, is food for higher trophic levels such as fish (Dahl and
Greenberg 1996, Wipfli 2005).
In addition to the bottom-up controls of food-web dynamics, top-down
regulation in freshwater streams has been demonstrated by several studies
(Gibson et al. 2004, Malmqvist 2002, Peckarsky and Dodson 1980, Walde
and Davies 1984). Fish predation can reduce stream invertebrate volume up
to 90%, relative to sections of stream without fish (Gilliam et al. 1989).
1Biology Department, University of Southern California, Los Angeles, CA. 2Biology
Department and Keck Environmental Lab, College of William and Mary,
Williamsburg, VA . *Corresponding author - rmcham@wm.edu.
462 Northeastern Naturalist Vol. 14, No. 3
Some fish species exhibit size preferences for smaller invertebrates
(Schlosser and Ebel 1989) or larger invertebrates (Gilliam et al. 1989),
whereas other species are thought to maintain a mixed-size diet of invertebrates,
regardless of relative or absolute abundance of different prey sizes
(Bence and Murdoch 1986). Because fish predation can alter the distribution
of shredders in streams, the influence of fish predation could extend down
two trophic levels and change the rate of leaf-litter breakdown (Konishi et al.
2001, Obendorfer et al. 1984, Ruetz et al. 2002).
The objective of the present study was to determine the potential influence
of fish predation on food-web dynamics in three headwater streams on
the southeastern Virginia coastal plain. In all streams, the most abundant
macroinvertebrate was the leaf-shredding amphipod Gammarus
pseudolimnaeus Bousfield. We determined experimentally the abundance
and sizes of amphipods occupying leaf litter in sections of streams with and
without fish, for comparison with laboratory determinations of fish-feeding
preferences on different sizes of amphipods. We also compared changes in
leaf-litter biomass and quality, to determine whether top-down controls of
shredder populations might extend to the base of the food web.
Methods
Study site
This study was conducted in three headwater streams leading into Lake
Matoaka in Williamsburg, VA: Strawberry Creek, Pogonia Creek, and Berkeley
Creek (Fig. 1). Each of the stream sub-watersheds is dominated by
second-growth hardwood forest > 100 years old (Table 1). Stream water
quality was determined on a single date in June 2005 from spot sampling and
analysis for dissolved nutrients using standard analytical methods (Parsons
et al. 1984).
Two sites per stream were chosen for the study based on the presence
or absence of fish in downstream and upstream locations, respectively
(Fig. 1). In both locations, stream depth was < 10 cm, and the water was
clear. Besides the presence of fish, downstream locations in closer proximity
to Lake Matoaka were characterized qualitatively by slightly
broader stream channels, finer sediment in the streambed, and slower
moving water.
The primary source of organic matter in these streams is leaf litter from
the surrounding forest, and instream algal production is very low (Mahon
Table 1. Sub-watershed area, forest coverage, and water quality in the Lake Matoaka drainage
basin in southeastern Virginia. SRP = soluble reactive phosphorus. BD = below detection.
Water quality (g/L)
Sub-watershed Area (km2) % forest SRP NO2
-+NO3
- NH4
+
Strawberry Creek 0.61 72 39 421 53
Pogonia Creek 0.33 96 BD 76 36
Berkeley Creek 0.71 84 BD 560 94
2007 E. Wach and R.M. Chambers 463
1997). The most abundant stream invertebrate by far is the amphipod
Gammarus pseudolimnaeus, a leaf shredder (Zehmer et al. 2002) that numerically
comprises >95% of individuals from all species (G.M. Capelli,
College of Williams and Mary, Williamsburg,VA, pers. comm.). From
sweep-net sampling, Gambusia holbrooki Girard (Mosquitofish) and
Clinostomus funduloides Girard (Rosyside Dace) were the most abundant
fish in the streams, with fewer Lepomis spp. (Sunfish) and Semotilus
atromaculatus Mitchill) (Creek Chub) (Saerom Park, Williams
College,Williamstown, MA, pers. comm.). The upstream absence of fish
was indicated by extensive, unsuccessful seining and sweep netting throughout
the stream channel and along undercut creekbanks.
Fish predation on amphipods
Rosyside dace and mosquitofish were collected during the second week
of June 2005 and held in aquaria at the laboratory. Amphipods were
collected from Strawberry Creek and held in a separate aquarium. For fishpredation
experiments, a single fish was measured for total length to the
nearest mm and placed into a 600-ml beaker filled with stream water and
containing 10 live amphipods that had been blotted dry and measured individually
for mass to the nearest 0.1 mg (wet weight). Weighing amphipods
after blotting with absorbent paper is a repeatable measure with <0.05%
error (Zehmer et al. 2002). In each beaker, we provided the fish a range of
amphipod sizes, from as small as 0.4 mg to as large as 19.5 mg. After 24
Figure 1. Site map of
the Lake Matoaka watershed
on the Virginia
coastal plain
(76.72ºW, 37.27ºN)
showing the location of
sub-watershed boundaries
(solid lines),
headwater streams
(dashed lines), and
mesh bags (crosshatched
circles) in areas
with and without
fish (downstream and
upstream, respectively).
464 Northeastern Naturalist Vol. 14, No. 3
hours, the fish was removed and any remaining amphipods were blotted dry
and weighed to determine which ones had been consumed by the fish. A total
of 30 Rosyside Dace and 22 Mosquitofish were tested. Three separate
beakers containing 10 amphipods and no fish lost no amphipods over the 24
hours, demonstrating that amphipod loss was likely due to consumption by
fish. The average weights of live amphipods eaten and uneaten by Rosyside
Dace and Mosquitofish were compared using t-tests.
Amphipod abundance and size in streams
Nylon bait bags (0.25” mesh size) were filled with 50 grams of air-dried,
mixed leaf litter of Quercus alba L.(white oak), Q. rubra L. (red oak) and Q.
michauxii Nutt. (swamp chestnut oak) collected from the forest floor in the
Lake Matoaka watershed. In each of the three streams, three bags were
tethered and submerged in a line across the stream channel in both upstream
and downstream locations. Twice per week, all bags were lifted off the
sandy streambed and flipped over to keep bags from being buried. In the
laboratory, three additional control bags were placed in deionized water, and
the water was changed every week.
After three weeks, all bags from each upstream and downstream location
and the three control bags were harvested. Each bag was shaken for a total of
one minute in three separate buckets of water containing a mesh-screen
bottom to remove sand and isolate amphipods. All amphipods from the
rinses were blotted dry and weighed as a group, then a 10-cm3 sub-sample
was weighed, and all amphipods in the sub-sample were counted to calculate
average amphipod weight. The sub-sample measurements of number and
weight were used with group weight to calculate the total number of amphipods
per bag. Amphipod weight and abundance between upstream and
downstream locations were compared using t-tests.
Litter weight and CNP analysis
After rinsing to remove amphipods and sand, the harvested bags of litter
were then dried in an oven at 80 ºC and weighed. Elemental analysis for total
carbon (C) and nitrogen (N) was completed on milled litter using a Perkin-
Elmer 2400 Analyzer. Total phosphorus (P) was determined using an ashing/
acid-extraction method after Chambers and Fourqurean (1991). Upstream
and downstream litter weights were compared using a t-test. ANOVA with
post-hoc comparisons was used to test the differences in average C, N, and P
content among control, upstream, and downstream litter.
Results
Fish predation on amphipods
For both Rosyside Dace and Mosquitofish, smaller amphipods were
consumed relative to larger ones (t-tests, P < 0.01, Fig. 2). Twenty-two
Mosquitofish, ranging in size from 20 to 45 mm, consumed a total of 178
amphipods weighing an average ± s.e. of 4.9 ± 0.3 mg. The 70 uneaten
2007 E. Wach and R.M. Chambers 465
amphipods weighed an average of 9.6 ± 0.6 mg. Similarly, for 28 trials with
Rosyside Dace, ranging in size from 44 to 52 mm, the fish consumed 224
amphipods weighing an average of 5.8 ± 0.3 mg. The 62 uneaten amphipods
weighed an average of 11.4 ± 0.4 mg.
Amphipod abundance and size in streams
Averaged across all three streams, more than three times as many amphipods
were recovered from nine litter bags collected upstream in the absence
of fish than downstream in the presence of fish: an average ± s.e. of 978 ±
122 amphipods upstream versus 289 ± 50 amphipods downstream (P <
0.001). The pattern of greater average numbers of amphipods was observed
for all three streams individually (Fig. 3). Further, the average weight of
amphipods collected from nine upstream litter bags in the absence of fish
was significantly smaller than for amphipods collected from downstream
litter bags in the presence of fish (N = 9, average ± s.e. of 8.1 ± 0.1 mg
upstream versus 13.9 ± 0.1 mg downstream, P < 0.01). Litter bags from
downstream locations in the presence of fish contained fewer, larger amphipods
relative to upstream locations, which contained more abundant, smaller
amphipods. The difference in amphipod size paralleled the pattern of fish
predation on amphipods, for which smaller amphipods were consumed preferentially
to larger amphipods (Fig. 2).
Figure 2. Mean weight (+ standard error) of amphipods eaten and uneaten by G.
holbrooki (Mosquitofish) and C. funduloides (Rosyside Dace), and of amphipods
collected in field litter bags from upstream (fish absent) and downstream (fish
present) locations.
466 Northeastern Naturalist Vol. 14, No. 3
Litter weight and CNP analysis
After three weeks of submergence in streams, dry-litter weight was not
significantly different between upstream and downstream locations
(N = 9, average ± s.e. of 47.4 ± 1.2 g upstream versus 46.2 ± 2.1 g
downstream, t-test P > 0.05). Some of the litter bags weighed more than the
initial 50 g, which we attributed to fine sand that could not be fully washed
out of the bags. Litter that had been submerged for three weeks in deionized
water in the lab had, on average, 6% higher carbon and 25% lower nitrogen
and phosphorus content, relative to litter submerged in streams (Fig. 4).
Despite these significant differences between control and field litter
(ANOVA, P < 0.05), there were no significant post-hoc differences in
elemental content between upstream and downstream locations.
Discussion
Results of the current study demonstrate the impact of fish on trophic
structure in headwater streams on the coastal plain of Virginia. Both amphipod
size (Fig. 2) and number (Fig. 3) were shown to be altered by fish
predation, revealing a top-down influence of fish predation on the dominant
invertebrate food source in the streams (Zehmer et al. 2002). The downstream
presence of fish influences energy processing and resource utilization in
headwater stream communities (Baxter et al. 2004, Vannote et al. 1980).
Although many studies have shown effects of fish predation on prey
populations in streams (Gibson et al. 2004, Malmqvist 2002, Peckarsky et al.
Figure 3. Mean number (+ standard error) of amphipods per litter bag in upstream
and downstream locations from all three streams, after three weeks (N = 9).
2007 E. Wach and R.M. Chambers 467
Figure 4. Posthoc
comparison
of elemental
content of
litter bags
from upstream
and downstream
locations
relative
to laboratory
c o n t r o l s
(mean + standard
error).
Asterisks denote
values
significantly
different from
controls (P less than
0.05). No
comparisons
between upstream
and
downstream
samples were
significantly
different.
468 Northeastern Naturalist Vol. 14, No. 3
2005, Zhao et al. 2006), fewer studies have demonstrated how alteration of
prey density can impact lower trophic levels (Konishi et al. 2001,
Obendorfer et al. 1984, Ruetz et al. 2002). In the current study, we saw no
difference in leaf-litter quantity or quality in sections of streams with and
without fish (Fig. 4), nor among streams with different water-quality characteristics
(Table 1). In the absence of large populations of other invertebrate
detritivores, our data suggest that the shredding of leaves by fewer, larger
amphipods in the presence of fish is similar to the action by more abundant
but smaller amphipods in the absence of fish.
Leaf breakdown by macroinvertebrates, however, can vary due to many
factors including shredder density (Chaffin et al. 2005), shredder size
(Cummins et al. 1972), leaf type (Lecerf et al. 2005), and water-borne chemical
cues (DeLange et al. 2005). Also, leaf breakdown can be driven largely by
physical action, although the large numbers of G. pseudolimnaeus and the
ability of this species to digest cellulose (Chamier 1991) suggest the strong
influence of biological activity. A longer-term study of litter changes might
detect changes in breakdown rate as a function of amphipod number and size.
Finally, downstream drift can reduce the upstream amphipod population
size, with amphipods using litter both as a source of food and as potential
refuge from predators downstream (Friberg and Jacobsen 1994, Hoffmann
2005). Stream invertebrate populations must offset losses to downstream drift
through both upstream migration and local reproduction (Kopp et al. 2001,
Williams and Williams 1993). The observed pattern of greater amphipod
number and smaller size upstream is consistent with these general life-history
characteristics of drift organisms. Thus, both top-down regulation by fish and
bottom-up control by leaf litter appear to influence G. pseudolimnaeus populations
in Virginia headwater streams.
Acknowledgments
This research using live vertebrate animals was completed under IACUC project
#0418 at the College of William and Mary. Thanks to Timothy Russell and Sarah
Gruber for laboratory assistance. The first author was an undergraduate REU student
funded by NSF-EAR grant #0243751—Interdisciplinary Watershed Studies at the
College of William and Mary.
Literature Cited
Baxter, C.V., K.D. Fausch, M. Murakami, and P.L. Chapman. 2004. Fish invasion
restructures stream and forest food webs by interrupting reciprocal prey subsidies.
Ecology 85:2656–2663.
Bence, J.R., and W.W. Murdoch. 1986. Prey-size selection by the Mosquitofish:
Relation to optimal-diet theory. Ecology 67:324–336.
Chaffin, J.L., H.M. Valett, J.R. Webster, and M.E. Schreiber. 2005. Influence of
elevated arsenic on leaf breakdown in an Appalachian headwater stream. Journal of
the North American Benthological Society 24:553–568.
Chambers, R.M., and J.W. Fourqurean. 1991. Alternative criteria for assessing nutrient
limitation of a wetland macrophyte (Peltandra virginica (L.) Kunth). Aquatic
Botany 40:305–320.
2007 E. Wach and R.M. Chambers 469
Chamier, A.C. 1991. Cellulose digestion and metabolism in the freshwater amphipod
Gammarus pseudolimneus Bousfield. Freshwater Biology 25:33–40.
Cuffney, T.F., J.B. Wallace, and G.J. Lugthart. 1990. Experimental evidence quantifying
the role of benthic invertebrates in organic-matter dynamics of headwater
streams. Freshwater Biology 23:281–299.
Cummins, K.W., R.C. Peterson, F.O. Howard, J.C. Wuycheck, and V. Holdt. 1972.
The utilization of leaf litter by stream detritivores. Ecology 54:336–345.
Creed, R.P., and J.M. Reed. 2004. Ecosystem engineering by crayfish in a headwater
stream community. Journal of the North American Benthological Society
23:224–236.
Dahl, J., and L. Greenberg. 1996. Impact on stream benthic prey by benthic- vs. driftfeeding
predators: A meta-analysis. Oikos 77:177–181.
De Lange, H.J., M. Lurling, B. Van den Borne, and E.T.H.M. Peeters. 2005. Attraction
of the amphipod Gammarus pulex to water-borne cues of food.
Hydrobiologia 544:19–25.
Fisher, S.G., and G.E. Likens. 1972. Stream ecosystem: Organic energy budget.
Bioscience 22:33–35.
Friberg, N., and D. Jacobsen. 1994. Feeding plasticity of 2 detritivore-shredders.
Freshwater Biology 32:133–142.
Gibson, C.A., R.E. Ratajczak, and G.D. Grossman. 2004. Patch-based predation in a
southern Appalachian stream. Oikos 106:158–166.
Gilliam, J.F., D.F. Fraser, and A.M. Sabat. 1989. Strong effects of foraging minnows
on a stream benthic invertebrate community. Ecology 70:445–452
Hall, R.O., J.W. Wallace, and S.L. Eggert. 2000. Organic-matter flow in stream food
webs with reduced detrital resource base. Ecology 81:3445–2463.
Hoffmann, A. 2005. Dynamics of fine particulate organic matter (FPOM) and
macroinvertebrates in natural and artificial leaf packs. Hydrobiologia 549:167–178.
Konishi, M., S. Nakano, and T. Iwata. 2001. Trophic cascading effects of predatory fish
on leaf-litter processing in a Japanese stream. Ecological Research 16:415–422.
Kopp, M., J.M. Jeschke, and W. Gabriel. 2001. Exact compensation of stream drift as
an evolutionarily stable strategy. Oikos 92:522–530.
Lecerf, A., M. Dobson, C.K. Dang, and E. Chauvet. 2005. Riparian plant species loss
alters trophic dynamics in detritus-based stream ecosystems. Oecologia
146:432–442.
Mahon, S. 1997. Distribution and ecology of freshwater amphipoda in the Lake
Matoaka/College Woods area, Williamsburg, Virginia. M.A. Thesis. The College
of William and Mary, Williamsburg, VA. 39 pp.
Malmqvist, B. 2002. Aquatic invertebrates in riverine landscapes. Freshwater Biology
47:679–694.
Motomori, K., H. Mitsuhashi, and S. Nakano. 2001. Influence of leaf-litter quality on
the colonization and consumption of stream invertebrate shredders. Ecological
Research 16:173–182.
Newman, R.M. 1990. Effects of shredding-amphipod density on watercress Nasturtium
officinale breakdown. Holarctic Ecology 13:293–299.
Obendorfer, R.Y., J.V. McArthur, J.R. Barnes, and J. Dixon. 1984. The effect of
invertebrate predators on leaf-litter processing in an alpine stream. Ecology
65:1325–1331.
Peckarsky, B.L., and S.I. Dodson. 1980. Do stonefly predators influence benthic
distributions in streams? Ecology 61:1275–1282.
Peckarsky, B.L., J.M. Hughes, P.B. Mather, M. Hillyer, and A.C. Encalada. 2005.
Are populations of mayflies living in adjacent fish and fishless streams genetically
differentiated? Freshwater Biology 50:42–51.
470 Northeastern Naturalist Vol. 14, No. 3
Parsons, T.R., Y. Maita, and C.M. Lalli. 1984. A Manual of Chemical and Biological
Methods for Seawater Analysis. Pergammon Press, New York, NY.
Peterson, R.C., and K.W. Cummins. 1974. Leaf processing in a woodland stream
ecosystem. Freshwater Biology 4:343–368.
Ruetz, C.R., R.M. Newman, and B. Vondracek. 2002. Top-down control in a
detritus-based food web: Fish, shredders, and leaf breakdown. Oecologia
132:307–315.
Schlosser, I.J., and K.K. Ebel. 1989. Effects of flow regime and cyprinid predation
on a headwater stream. Ecological Monographs 59:41–57.
Short, R.A., and P.E. Maslin. 1977. Processing of leaf litter by a stream detritivore:
Effect on nutrient availability to collectors. Ecology 58:935–938.
Vannote, R.L., G.W. Minshall, K.W. Cummings, J.R. Sedell, and C.E. Cushing.
1980. The river-continuum concept. Canadian Journal of Fisheries and Aquatic
Sciences 37:130–137.
Walde, S.J., and R.W. Davies. 1984. Invertebrate predation and lotic prey communities:
Evaluation of in situ enclosure/exclosure experiments. Ecology 65:1206–1213.
Wallace J.B., T.F. Cuffney, S.L. Eggert, and M.R. Whiles. 1997. Stream organic
matter inputs, storage, and export for Sattelite Branch at Coweeta Hydrologic
Laboratory, North Carolina, USA. Journal of the North American Benthological
Society 16:67–74.
Wallace, J.B., S.L. Eggert, J.L. Meyer, and J.R. Webster. 1999. Effects of resource
limitation on a detrital-based ecosystem. Ecological Monographs 69:409–442.
Webster, J.R., J.B. Wallace, and E.F. Benfield. 1995. Streams and rivers of eastern
United States. Pp. 117–187, In C.E. Cushing, K. Cummins, and G.W.
Minshall (Eds.). River and Stream Ecosystems. Elsevier Press, Amsterdam,
The Netherlands.
Williams, D.D., and N.E. Williams. 1993. The upstream–downstream movement
paradox of lotic invertebrates: Quantitative evidence from a Welsh mountain
stream. Freshwater Biology 30:199–218.
Wipfli, M.S. 2005. Trophic linkages between headwater forests and downstream fish
habitats: Implications for forest and fish management. Landscape and Urban
Planning 72:205–213.
Zehmer, J.K., S.A. Mahon, and G.M. Capelli. 2002. Calcium as a limiting factor in
the distribution of the amphipod Gammarus pseudolimnaeus. American Midland
Naturalist 148:350–362.
Zhao, X.X., M.G. Fox, and D.C. Lasenby. 2006. Effect of prey density, prey mobility,
and habitat structure on size selection and consumption of amphipods by a
benthic-feeding fish. Archiv Für Hydrobiologie 165:269–288.