A Molecular Clone and Culture Inventory of the Root
Fungal Community Associated with Eastern Hemlock in
Great Smoky Mountains National Park
Richard Baird, C. Elizabeth Stokes, Alicia Wood-Jones, Clarence Watson, Mark Alexander, Glenn Taylor, Kristine Johnson, Paul Threadgill, and Susan Diehl
Southeastern Naturalist, Volume 13, Special Issue 6 (2014): 219–237
Full-text pdf (Accessible only to subscribers.To subscribe click here.)
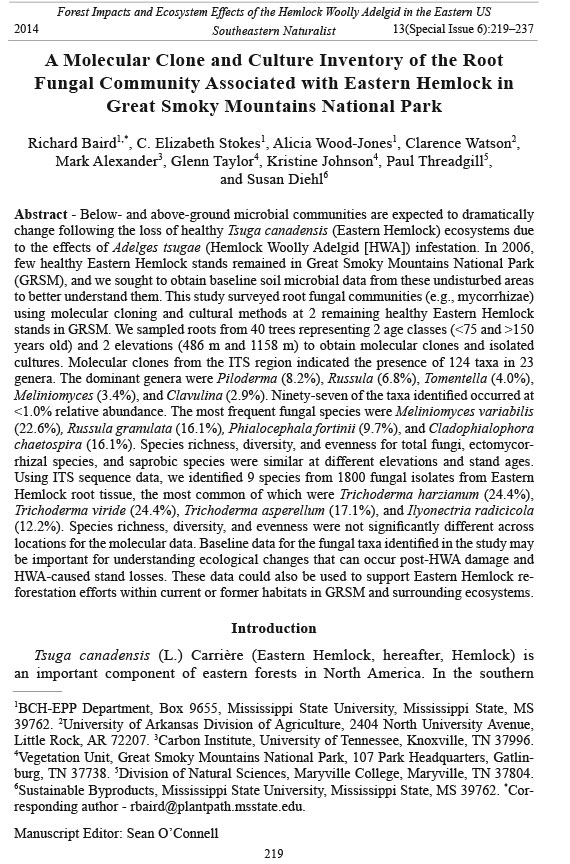
219
A Molecular Clone and Culture Inventory of the Root
Fungal Community Associated with Eastern Hemlock in
Great Smoky Mountains National Park
Richard Baird1,*, C. Elizabeth Stokes1, Alicia Wood-Jones1, Clarence Watson2,
Mark Alexander3, Glenn Taylor4, Kristine Johnson4, Paul Threadgill5,
and Susan Diehl6
Abstract - Below- and above-ground microbial communities are expected to dramatically
change following the loss of healthy Tsuga canadensis (Eastern Hemlock) ecosystems due
to the effects of Adelges tsugae (Hemlock Woolly Adelgid [HWA]) infestation. In 2006,
few healthy Eastern Hemlock stands remained in Great Smoky Mountains National Park
(GRSM), and we sought to obtain baseline soil microbial data from these undisturbed areas
to better understand them. This study surveyed root fungal communities (e.g., mycorrhizae)
using molecular cloning and cultural methods at 2 remaining healthy Eastern Hemlock
stands in GRSM. We sampled roots from 40 trees representing 2 age classes (<75 and >150
years old) and 2 elevations (486 m and 1158 m) to obtain molecular clones and isolated
cultures. Molecular clones from the ITS region indicated the presence of 124 taxa in 23
genera. The dominant genera were Piloderma (8.2%), Russula (6.8%), Tomentella (4.0%),
Meliniomyces (3.4%), and Clavulina (2.9%). Ninety-seven of the taxa identified occurred at
<1.0% relative abundance. The most frequent fungal species were Meliniomyces variabilis
(22.6%), Russula granulata (16.1%), Phialocephala fortinii (9.7%), and Cladophialophora
chaetospira (16.1%). Species richness, diversity, and evenness for total fungi, ectomycorrhizal
species, and saprobic species were similar at different elevations and stand ages.
Using ITS sequence data, we identified 9 species from 1800 fungal isolates from Eastern
Hemlock root tissue, the most common of which were Trichoderma harzianum (24.4%),
Trichoderma viride (24.4%), Trichoderma asperellum (17.1%), and Ilyonectria radicicola
(12.2%). Species richness, diversity, and evenness were not significantly different across
locations for the molecular data. Baseline data for the fungal taxa identified in the study may
be important for understanding ecological changes that can occur post-HWA damage and
HWA-caused stand losses. These data could also be used to support Eastern Hemlock reforestation
efforts within current or former habitats in GRSM and surrounding ecosystems.
Introduction
Tsuga canadensis (L.) Carrière (Eastern Hemlock, hereafter, Hemlock) is
an important component of eastern forests in North America. In the southern
1BCH-EPP Department, Box 9655, Mississippi State University, Mississippi State, MS
39762. 2University of Arkansas Division of Agriculture, 2404 North University Avenue,
Little Rock, AR 72207. 3Carbon Institute, University of Tennessee, Knoxville, TN 37996.
4Vegetation Unit, Great Smoky Mountains National Park, 107 Park Headquarters, Gatlinburg,
TN 37738. 5Division of Natural Sciences, Maryville College, Maryville, TN 37804.
6Sustainable Byproducts, Mississippi State University, Mississippi State, MS 39762. *Corresponding
author - rbaird@plantpath.msstate.edu.
Manuscript Editor: Sean O’Connell
Forest Impacts and Ecosystem Effects of the Hemlock Woolly Adelgid in the Eastern US
2014 Southeastern Naturalist 13(Special Issue 6):219–237
Southeastern Naturalist
R. Baird, et al.
2014
220
Vol. 13, Special Issue 6
Appalachians, Hemlock occurs most frequently at 610–1520 m elevation and is often
restricted to north and east slopes, coves, or cool, moist valleys (USDA 1965).
Hemlock is usually a medium-sized tree 18–22 m high and 1–2 m in diameter at
maturity. The first record of Adelges tsugae Annand (Hemlock Woolly Adelgid
[HWA]) in Great Smoky Mountains National Park (GRSM) is from 1996 (Johnson
et al. 2008). HWA had previously caused extensive loss and damage to thousands
of hectares of Hemlock north of the park. All Hemlock age- and size-classes are
susceptible to HWA infestation, but Hemlock mortality usually occurs 2–12 years
after the initial infestation (Mayer et al. 2002, McClure et al. 2001, Orwig 2002).
To offset the losses in the GRSM, managers treat hundreds of Hemlock trees
with the broad spectrum insecticide imidicloprid. The effects of this chemical on
below-ground arthropod and other invertebrate or arthropod faunal (Collembolan)
communities remain largely unknown (Reynolds 2008). Furthermore, the chemical
is a neonicotinoid insecticide with a half-life of 24–124 days, resulting in N residues.
Altered faunal communities and N-input could influence fungal community
baseline data as could the presence of vegetation associated with Hemlocks. An
additional literature review of impacts of those factors was presented in Baird et al.
(2014 [this issue]).
Little is known about the character and function of Hemlock-associated fungi,
and no comprehensive research is available on the assemblages of soilborne fungi
associated with stands of healthy mature Hemlock. A survey of fungal taxa was conducted
in mature stands of Tsuga heterophylla (Raf.) Sarg. (Western Hemlock) in
the Pacific Northwest (Wright et al. 2009), but few formal investigations are available
showing baseline data regarding root fungal communities in the southern US.
Walker et al. (2005, 2008) and Baird et al. (2013), provided reviews of the literature
concerning fungal communities and forest ecosystems in the southern Appalachian
Mountains, but these were not specific to Hemlock ecosystems. Without baseline
data on species diversity from healthy, pre-HWA-infested Hemlock, comparisons
of any soil microbial changes that occur during and after HWA infestations are
difficult, if not impossible. Baseline data on a healthy ecosystem is necessary for
evaluating the health of the resulting ecosystem (Dyke 2003).
The purpose of this study was to determine species composition, richness, and
diversity of soilborne saprobic, pathogenic and ectomycorrhizal fungi associated
with healthy Hemlock stand roots. Specific objectives included collection of
baseline data of fungal populations in GRSM using traditional cultural isolation
methods and molecular techniques. The results of this study were also included in
the All Taxa Biodiversity Inventory (ATBI) of GRSM in order to further the effort
to document all life in this national park (Sharkey 2001).
Materials and Methods
Study sites and general data collection
We established plots in May 2006 at 2 locations within the GRSM where mature
Hemlock stands had high vigor and crown-health ratings (Schomaker et al. 2007):
Copeland Creek (487 m elevation) and Gabes Mountain Trail (1158 m elevation).
Southeastern Naturalist
221
R. Baird, et al.
2014 Vol. 13, Special Issue 6
At each location, we set up a 48.8 x 48.8-m plot and subdivided it into four 24.4 x
24.4-m subplots. We recorded the plot corners as Universal Transverse Mercator
(UTM) coordinates using a Garmin III Plus global positioning system (GPS; Garmin
International Inc., Olathe, KS). We mapped the location of each Hemlock tree
within the subplots, measured its diameter at breast height (dbh), and determined
tree heights using a Suunto® clinometer (http://www.suunto.com). To allow us to
calculate estimates of spatial correlations between fungal occurrences and associated
vegetation, we inventoried and characterized all other tree species and woody
vegetation within the plots.
We recorded tree-crown health-indicator data for all Hemlock trees within each
plot at both locations to verify that we had selected healthy trees for root sampling
(Baird et al. 2014 [this issue]). Crown-condition classification included vigor
class, uncompact live-crown ratio, crown light exposure, crown position, crown
density, crown dieback, and foliage transparency (Schomaker et al. 2007). These
indicators of crown health were based on Hemlock stands rated at vigor 1 rating or
>35% crown ratio, which indicated high vigor or healthy sites. We found that our
putatively healthy study sites had ≈10–30% foliage infestation, as assessed by tree
climbers at the beginning of the study; however, needles remained intact and the
crowns were rated as healthy just prior to initiation of the st udy.
At both locations, we sampled microbial populations 2–4 September, ≈2 weeks
after an imidacloprid application. We collected rhizosphere samples from within a
1.0-m zone near the bases of 20 trees at each site. For each sample, we collected
a 120-cm length of secondary root with feeder roots and soil attached. We made our
collections by carefully removing the litter layer and gently removing soil from the
0–10-cm soil horizon (A–B horizons) to follow root attachment to each Hemlock
tree. We placed the samples in 3.8-liter Ziploc® plastic bags, stored them in a cooler
at 10 °C, and subsequently transferred them to refrigerators for storage at 4 °C. We
processed root samples within 48 h of collection. We divided the 120-cm root and
attached soil samples into 2 equal sections of 60 cm, and randomly selected one section
for isolation or molecular identification of fungi. We used a scalpel to dissect
the 60-cm root sample from each replicate tree into 1-cm segments.
For the cultural isolations, we placed the 1-cm root segments in a 2.0-mm No.
10-mesh sieve and washed them under gently running tap water for 10 min to remove
soil and adhering plant debris (Dighton and Harrison 1983, Goodman et al.
1996). We randomly selected 10 secondary root segments and 10 feeder root segments
per tree for isolation of fungi, with 5 secondary and 5 feeder roots placed on
Petri plates for each of two growth media. To isolate common root and associated
ectomycorrhizal taxa, we plated root segments onto a Petri dish (100 x 15 mm,
Fisherbrand™, Fisher Scientific, Pittsburgh, PA) containing either 12.5 g malt extract
agar (MEA) (Difco® Bacto® malt agar, Detroit, MI) amended with 8 mg/liter of
streptomycin sulfate and 50 mg/liter of chlortetracycline (Pearson and Read 1973),
or onto Petri dishes containing modified Melin-Norkrans agar (MMN) (Fortin and
Piché 1979). We incubated inoculated plates in the dark at 20–25 °C and examined
them at regular intervals for 4 days (Lilleskov et al. 2002, Pearson and Read 1973).
Southeastern Naturalist
R. Baird, et al.
2014
222
Vol. 13, Special Issue 6
We subcultured all fungi onto fresh MEA or MMN nutrient agar in Petri plates
(60 x 15 mm, Fisherbrand™) at ambient temperature and lighting. We aseptically
transferred the isolates to 1.2-ml autoclaved cryogenic vials (Corning, Acton, MA)
with 800 μl of a glycerol solution (15% glycerol + 85% sterile distilled water) and
placed the isolates in cryogenic storage at -80 °C for later identification. We stored
an additional set of fungal cultures in test tubes with 100 ml sterile distilled water
(five 3-mm3 agar plugs/tube).
To prepare fungal isolates for initial morphological groupings, we removed two
3-mm3 mycelial plugs for each isolate from storage at -80 °C and plated them on
the general all-purpose growth medium potato dextrose agar (PDA, Difco®, Detroit,
MI) (Watling 1987, Villarroel et al. 2004). We incubated isolates in the dark at
20–25 °C for 7 days, and subcultured the resulting colonies on fresh PDA, MMN,
and MEA as described above. Species identification of the fungal isolates from pure
culture followed methods by Barnett and Hunter (1987), Ellis (1971), Roy et al.
(2001), Sutton (1980), and Watling (1987). We further determined representative
isolates of similar morphological groups using ITS sequence data and methods as
described below.
We excised the remaining 60-cm root sample from each tree into 1-cm-long
segments and stored them for up to 48 h in 2X CTAB prior to DNA extraction
(Landeweert et al. 2003). The roots were not rinsed prior to DNA extraction. For
these DNA extractions, we randomly chose 5 mg of the root segments per replicate
tree and ground the sample with liquid nitrogen using a mortar and pestle. Rootassociated
fungal genomic DNA was extracted using a DNeasy® Plant Mini Kit
(Qiagen, Valencia, CA). We repeated the elution process once. All genomic DNA
was stored at -20 ºC until further processing.
We used genomic DNA from root-tissue samples for taxon identification. We
used internal transcribed spacer region primers ITS1F and ITS4 for PCR, molecular
cloning, and sequencing to identify species (Mata et al. 2007,White et al.
1990). We identified unique clones by restriction fragment length polymorphism
(RFLP) patterns of the ITS region using endonucleases Alu1 and Hinf1 (Bruns et
al. 2002). We isolated for sequencing a total of 50 clones per sample from each
replicate sample (Kennedy and Clipson 2003). Amplicons were purified with
a Qiagen QIAquick kit. Fungal sequencing was conducted at the laboratory of
MWG Biotech, Inc., Huntsville, AL. We visually inspected electropherogram
output for sequencing errors using CodonCode Aligner version 3.5.6. The contigs
of the sequences were constructed using the SeqMan application of the Lasergene
version 7.0 software package (DNASTAR, Inc., Madison, WI). We used
MegAlign (DNASTAR) to align nucleotide sequences (Challen et al. 2003). All
sequence data were compared using the sequence library from GenBank BLAST
searches to determine identities (Altschul et al. 1997). We considered an ITS fungal
sequence that matched with a sequence in GenBank to be the same species
when 80% coverage or higher showed a minimum of 97% homology (Hughes et
al. 2009). Following completion of the research, we deposited all sequences in
GenBank (Altschul et al. 1997).
Southeastern Naturalist
223
R. Baird, et al.
2014 Vol. 13, Special Issue 6
We also assessed relationships among fungal diversity values and between fungal
diversity and plant and tree distribution patterns in the experimental plots. This
experiment was a completely randomized design. We used analysis of variance
(ANOVA) for all data, we used Fisher’s protected least significant difference test
(P < 0.05) to compare means, and we used the Pearson correlation procedure to
analyze the data.
Identification and enumeration of fungal species associated with confirmed
Hemlock tree roots at both sites allowed us to calculate species richness and diversity
indices for each functional group. Biodiversity indices (Table 1) calculated
for fungi included species richness (n), Shannon-Weaver species diversity index
(H'), Shannon species evenness index (E), and coefficient of community (CC)
(Dyke 2003, Price 1997, Stephenson 1989, Stephenson et al. 2004). The Shannon
diversity index is highly sensitive to species evenness and the presence of rare
species. It is also the most widely used measurement of biodiversity that allows
for comparison of results with previous studies (Magurran 2004). We calculated
relative frequencies of fungal occurrence. Where appropriate, we further analyzed
data using one-way ANOVA and the general linear models procedure (Proc GLM)
of Statistical Analyses System Software (SAS Institute Inc. 1999).
Results
We evaluated woody plants at both sites to determine the possible associations
with the fungi present at the sites. We documented a total of 37 woody species at
the Copeland Creek and Gabes Mountain plots (Table 1). The most common species
were Acer pensylvanicum (Striped Maple) with an overall abundance of 10.8%,
Pyrularia pubera (Buffalo Nut; 10.5%), Ilex opaca (American Holly; 8.2%),
Calycanthus floridus (Carolina Sweetshrub; 4.8%), and Rhododendron maximum
(Great Rhododendron; 4.6%). Species abundance, richness, and diversity were
significantly higher at the Copeland Creek site (39.3 vs. 22.0, 10.3 vs. 5.6, and 1.8
vs. 1.4, respectively, than at Gabes Mountain (Table 2). Coefficient of community
values for woody vegetation comparisons between sites indicated that 52% of
species occurred at both sites. Richness, diversity, and evenness from frequency
data and those from abundance data were significantly correlated (Table 3). These
findings suggest that there were differences in plant species richness and diversity
between locations. Evenness data for plant species abundance was not equal, and
varied within and by location. Associated woody vegetation was not significantly
correlated with ectomycorrhizal, saprobic, or total fungal sequence data.
A total of 124 unique fungal taxa representing 23 genera were identified by the
analysis of 1492 unambiguous and non-chimeric sequences from Hemlock-root-associated
fungi (Appendix A). Percent identity to closest sequence matches utilizing
the GenBank database ranged between 87% and 100%. However all taxa with a
specific epithet listed were above 97%. The dominant 5 genera, which accounted
for 25% of the sequenced clones, included Piloderma (8.2%), Russula (6.8%), Tomentella
(4.0%), Meliniomyces (3.4%), and Clavulina (2.9%). A total of 97 fungal
taxa, including unknown fungal clone species, occurred at less than 1.0% relative
Southeastern Naturalist
R. Baird, et al.
2014
224
Vol. 13, Special Issue 6
abundance. Of the total fungal taxa identified, 75 were collected from Copeland
Creek and 73 from Gabes Mountain, including saprobic and symbiotic species.
Fungal taxa with the highest total percent frequency of occurrence per tree
(n = 40) included a reoccurring uncultured Helotiales clone 1 (25.7%), Meliniomyces
variabilis (22.6%), Basidiomycete sp. (19.2), Russula granulata (16.1%),
Table 1. Percent relative abundance of woody plants associated with Eastern Hemlock at two locations
in Great Smoky Mountains National Park. Abundance values are based on relative mean percent
occurrence of woody plant species from the two sites, Copeland Creek (487m) and Gabes Mountain
(1158m), ÷ 37 unique species. Overall abundance indicates mean percent of each taxon from both
sites ÷ 37.
Abundance by site
Overall Copeland Gabes
Woody Plant Taxa abundance Creek Mountain
Acer pensylvanicum L. (Striped Maple) 10.8 6.5 22.8
Acer rubrum L. (Red Maple) 4.5 4.6 4.4
Acer saccharinum Marsh. (Sugar Maple) 1.3 <1.0 3.9
Aristolochia macrophylla Lam. (Dutchman’s Pipe) <1.0 0.0 <1.0
Betula alleghaniensis Britt. (Yellow Birch) 1.4 <1.0 2.6
Betula lenta L. (Sweet Birch) <1.0 <1.0 0.0
Bignonia capreolata L. (Cross Vine) <1.0 <1.0 0.0
Calycanthus floridus L. (Carolina Sweetshrub) 4.8 6.0 1.3
Carpinus caroliniana Walter (Ironwood) <1.0 <1.0 0.0
Carya glabra (Mill.) Sweet (Pignut Hickory) 1.5 2.1 0.0
Clethra acuminata Mich. (Mountain Sweet Pepperbush) 1.3 1.4 <1.0
Cornus alternifolia L. (Alternate-leaved Dogwood) <1.0 <1.0 0.0
Cornus florida L. (Flowering Dogwood) <1.0 <1.0 0.0
Euonymus americanus L. (Strawberry Bush) 1.7 2.4 0.0
Fagus grandifolia Ehrh. (American Beech) 2.3 1.7 3.9
Halesia carolina L. (Carolina Silverbell) 3.7 0.0 14.0
Hamamelis virginiana L. (American Witch Hazel) <1.0 <1.0 0.0
Ilex opaca Aiton (American Holly) 8.2 11.2 0.0
Kalmia latifolia L. (Mountain Laurel) <1.0 <1.0 0.0
Leucothoe fontanesiana (Steud.) Sleumer (Highland Dog Hobble) 1.0 1.1 <1.0
Liriodendron tulipifera L. (Tulip Poplar) 1.3 1.4 <1.0
Magnolia acuminata (L.) L. (Cucumber Tree) <1.0 <1.0 0.0
Magnolia fraseri Walter (Fraser Magnolia) 1.3 1.3 1.3
Nyssa sylvatica Marsh. (Black Gum) 1.5 2.1 0.0
Oxydendrum arboreum (L.) DC. (Sourwood) 2.2 3.0 0.0
Pinus strobus L. (White Pine) <1.0 <1.0 0.0
Pyrularia pubera Michx. (Buffalo Nut) 10.5 14.2 0.0
Quercus alba L. (White Oak) <1.0 <1.0 0.0
Quercus rubra L. (Northern Red Oak) 1.6 1.3 2.6
Rhododendron maximum L. (Great Rhododendron) 4.6 1.1 14.5
Sassafras albidum (Nutt.) Nees (Sassafras) <1.0 <1.0 0.0
Toxicodendron radicans (L.) Kuntze (Poison Ivy) <1.0 <1.0 0.0
Tsuga canadensis (L.) Carrière (Eastern Hemlock) 26.0 26.5 24.6
Vaccinium spp. (blueberry) 2.2 3.0 0.0
Viburnum acerifolium L. (Maple-leaf Viburnum) 1.3 1.7 0.0
Viburnum spp. (viburnum) <1.0 0.0 <1.0
Vitis spp. (grape) <1.0 <1.0 0.0
Southeastern Naturalist
225
R. Baird, et al.
2014 Vol. 13, Special Issue 6
Piloderma sp. 3 (16.1%), and uncultured Venturaceae (12.2%) (Appendix A).
The ectomycorrhizal fungus Russula atropurpurea had a low overall frequency
at 6.5%, but it was abundant when present. Meliniomyces variabilis, which is a
member of the Pezizomycotina and has strong phylogenetic affinities to ericoid
mycorrhizal species Rhizoscyphus ericae, had low abundance at <1.0% but higher
frequency than any other taxon (22.6%). The majority of taxa (98) occurred in
only 1 of the 40 replicate root samples. For fungal frequency data, species richness,
diversity, and evenness for total, ectomycorrhizal, and saprobic fungi were
similar across sites (data not shown). Hymenoscyphus monotropae associated with
Monotropa uniflora L. (Indian Pipe) was cloned at a low frequency (Brant 1988).
This fungal species is a member of the Leotiomycetes, and a high percentage of
clones were identified to this class level, which suggests that a large number of
these mycorrhizal fungi are present.
All measured fungal community parameters were positively and significantly
correlated. Ectomycorrhizal, saprobic, and total fungi generally corresponded
in richness, diversity, and evenness across sites. For example, higher values for
richness corresponded with higher levels of diversity and evenness regardless of
taxonomy or ecological role of fungi (Table 4). There were no significant trends for
each study site based on fungal species richness, diversity, or evenness.
The cultures contained over 1800 fungal isolates obtained from Hemlock root
tissue at the two sites. Isolates were separated into 43 unique groups based on
Table 3. Correlations among predictor variables for Hemlock-associated woody vegetation at 2 locations
in Great Smoky Mountains National Park. Associated woody vegetation was inventoried in
sixty-four 6 x 6-m subplots per site (Copeland Creek and Gabes Mountain). Abundance = number of
individual woody plants, richness = number of plant species, diversity = Shannon diversity index,
evenness = Shannon evenness index, and * = Pearson correlation procedure R-values with significant
relationships (P ≤ 0.05).
Associated woody vegetation
Richness Diversity Evenness
Abundance 0.802 * 0.606 * 0.752 *
Richness 0.901 * 0.533 *
Diversity 0.662 *
Table 2. Species abundance, richness, and diversity of woody vegetation associated with Eastern
Hemlock by study site in Great Smoky Mountains National Park. Copeland Creek is a second-growth
mature hemlock forest (487 m elevation); Gabes Mountain is a virgin old-growth forest (1158 m elevation).
Abundance = mean number of individuals; richness = mean number of individual species;
and diversity = Shannon diversity index. Means followed by the same letter in the same column are
not significantly different using Fisher’s LSD test (P > 0.05).
Associated woody vegetation
Site Abundance Richness Diversity
Copeland Creek 39.3 a 10.3 a 1.8 a
Gabes Mountain 22.0 b 5.6 b 1.4 b
LSD (P < 0.05) 6.5 2.2 0.3
Southeastern Naturalist
R. Baird, et al.
2014
226
Vol. 13, Special Issue 6
morphology. We identified 9 unique fungal taxa representing 5 genera using unambiguous
and non-chimeric fungal isolate sequences from the representative groups
(Table 5). Trichoderma was the dominant genus, accounting for 42.0% of isolates.
Four taxa were found in common to both study sites: Pestalotiopsis macuiformans,
Trichoderma asperellum, Trichoderma harzianum, and Hypocrea koningii. Fungal
taxa with the highest frequency of occurrence were Trichoderma harzianum
Table 5. Mean percent frequency and relative abundance of culturable fungi associated with roots of
Eastern Hemlock in Great Smoky Mountains National Park. Abundance values are based on mean
percent relative abundance of fungal taxa from two sites (Copeland Creek (CC; 487 m) and Gabes
Mountain (GM; 1158 m); total number of isolates per species ÷ 800 isolates (= 2 sites × 20 root pieces/
tree × 20 trees) × 100. The National Center of Biotechnology Institute (NCBI) accession numbers
listed are based on ≥97% sequence homology over >80% coverage of fungal taxa in the GenBank database.
Freq. indicates mean percent occurrence of fungal species per root sample, each collected from
a single Eastern Hemlock tree: mean percent ÷ 40 (= 2 sites × 20 trees) × 100. Overall abundance values
are based on mean percent relative abundance of total fungal taxa identified from Eastern Hemlock
root samples (= total mean percent relative abundance from all sites and treatments ÷ 124 unique taxa).
Abundance
by site
NCBI Overall
Fungal taxon Accession Freq. CC GM abund.
Trichoderma harzianum Rifai/ Hypocrea lixii Pat. KF856954 55.6 54.5 14.3 24.4
Trichoderma sp. KF856955 16.7 0.0 10.7 7.3
Ilyonnectria radicicola (Gerlach & L. Nilsson) P. KF856956 27.8 0.0 14.3 12.2
Chaverri & Salgado (= Neonectria radicicola (Gerlach
& L. Nilsson) Mantiri & Samuels)
Pestalotiopsis maculiformans (Guba & Zeller) KF856957 11.1 9.1 3.6 4.9
Steyaert
Rhizopus arrhizus A. Fisch. (= Rhizopus oryzae KF856958 5.6 0.0 3.6 2.4
Went & Prins. Geerl.)
Trichoderma asperellum Samuels, Lieckf. & Nirenberg KF856959 38.9 9.1 7.9 17.1
Trichoderma hamatum (Bonord.) Bainier KF856960 11.1 18.2 0.0 4.9
Hypocrea koningii Lieckf., Samuels & W. Gams KF856961 5.6 9.1 32.1 2.4
Trichoderma viride Pers. KF856962 50.0 0.0 32.1 24.4
Table 4. Correlations among predictor variables for fungi associated with Eastern Hemlock, determined
by molecular methods at 2 locations in Great Smoky Mountains National Park. Richness =
number of fungal taxa, diversity = Shannon diversity index, Evenness = Shannon evenness index, and
* = Pearson correlation procedure R-values with significant relationships (P ≤ 0.05).
Diversity Evenness
Total fungi predictor
Richness 0.835 * -0.232
Diversity 0.249
Ectomycorrhizae predictor
Richness 0.918 * 0.811 *
Diversity 0.924 *
Saprobe predictor
Richness 0.963 * 0.820 *
Diversity 0.919 *
Southeastern Naturalist
227
R. Baird, et al.
2014 Vol. 13, Special Issue 6
(55.6%), Trichoderma viride (50.0%), Trichoderma asperellum (38.9%), and
Ilyonectria radicicola (27.8%), but many taxa varied in occurrence between sites.
Results for abundance, richness, diversity, or evenness of culturable fungi indicated
no other significant or consistent trends. All fungal and plant data were submitted
to the ATBI database for the GRSM.
Discussion
This research represents the first major survey of fungi associated with healthy
Hemlock stands in GRSM using molecular sequence data. In particular, we attempted
to identify important fungal symbionts before the few remaining healthy
habitats in GRSM were destroyed by HWA. Similar surveys have been conducted
in regenerating Hemlock forests at the northern extreme of the range (McLenon-
Porter 2008), on Quercus spp. (oak) seedlings in the southern Appalachians (Walker
et al. 2005), and in other forest types (Burke et al. 2009, DeBellis et al. 2006,
Dickie et al. 2009). These and other surveys, while conducted across a variety of
host species and forest types, provide some basis for comparison and ecological
interpretation of the results of the current study.
The GRSM is an ideal location to study fungal diversity due to the history of
long-term management and protection of its old-growth Hemlock forests prior to
losses from HWA infestation. At the onset of this study, a few sites in the GRSM
had low HWA-infestation levels (10–30%), thus, the Copeland Creek and Gabes
Mountain sites provided an opportunity to collect pre-disturbance, fungal baseline
data; at <5% observed canopy loss, only minor damage to the trees at these study
sites was evident.
The Copeland Creek location was composed of ≈75y-old mature second-growth
Hemlock forest with intermittent mixed hardwoods (T. Ramely et al., GRSM,
unpubl. data). Additionally, a component of large Pinus strobus (White Pine) at
Copeland Creek were killed by Dendroctonus frontalis Zimmerman (Southern Pine
Bark Beetle) infestation within 5 y before the study, resulting in diverse woody
plant regeneration in the understory and abundant coarse woody debris on the forest
floor. The Gabes Mountain site had >150 y-old Hemlocks and was classified as
a high-elevation virgin Hemlock forest with minor components of large Halesia
carolina (Carolina Silverbell) and Tilia americana L. (American Basswood) and a
sparse understory dominated by Striped Maple and Great Rhododendron. In addition,
this site was included in a large tract of old-growth Hemlock being managed as
a long-term ecological and genetic conservation stand by the National Park Service
(Johnson et al. 2008; T. Remaley, pers. comm. 2006).
At least one-third of the fungal taxa identified during the root study were previously
reported to be mycorrhizal mutualists. In a previous 3-year survey that
evaluated the occurrence of fleshy fungi in Hemlock forests (Baird et al. 2014 [this
issue]), the most abundant taxa were species of Lactarius and Russula that were
also present in over 70% (29 of 40 samples) of root-tissue samples in the current
study. Several tomentelloid taxa such as Tomentella sublilacina and corticoid taxa
(Corticiaceae) were also frequent, occurring in 26% of samples (11 of 40 samples).
Southeastern Naturalist
R. Baird, et al.
2014
228
Vol. 13, Special Issue 6
Such high richness of the family Russulaceae and tomentelloid/thelephoroid types
is typical of 16 previous studies in coniferous forests (Horton and Bruns 2001), as
well as other more recent research (Porter et al. 2008, Walker et al. 2005, Wright et
al. 2009). However, our results must be viewed with some caution due to an overall
low sampling effort. Other research using traditional surveying and modern pyrosequencing
techniques confirmed that over 1000 fungal taxa consisting primarily
of ascomycetous and basidiomycetous ectomycorrhizae were identified within a
post-glacial willow ecosystem (Jumpponen 2007, Jumpponen et al. 2010). Those
totals are almost 5 times the number of taxa that occurred in this study from GRSM;
however, those papers reported that many unknown species were encountered and
may have been PCR artifacts or undetermined chimeric sequences. These potential
pitfalls are a conern with any molecular diversity study, but they are potentially
outweighed by the inclusion of fastidious and non-culturable species that would be
missed using conventional methods (Hoshino and Matsumoto 2007).
The majority of mycorrhizal taxa identified during this study were ectomycorrhizal
fungi, including members of the Helotiales, such as Phialophora and
Lachnum species. Phialophora americana is capable of a variety of interactions
with host plants, including dematiaceous hyphomycete endophytes (Jumpponen
and Trappe 1998), ectomycorrhizae and ectendomycorrhizae (Wilcox and Wang
1987), and ericoid mycorrhizae (Monreal et al. 1999, Weiss et al. 2004). The role or
associations of Lachnum spp. in forest ecosystems remains uncertain (Walker et al.
2005). A number of recent studies have found ericoid mycorrhizal fungi colonizing
the roots of tree species in the family Pinaceae and other nonericoid plant species
(Hambleton and Sigler 2005, Vohnik et al. 2007). Ericoid-like fungi were identified
from Hemlock-root sequences in this study. Meliniomyces, believed to form ericoid
mycorrhizae, was identified in 6 root samples and was high in abundance and frequency
in our plots. Whether Hemlock roots act as alternate or primary hosts for
these fungi in the absence of ericaceous plants is uncertain (Burke et al. 2009).
Numerous fungal taxa collected during this study had been previously documented
as associated with Western Hemlock roots (Kropp and Trappe 1982,
Wright et al. 2009). Similarly to findings in a previous study by Wright et al.
(2009), root-sequence data from our study sites (Baird et al. 2014 [this issue])
detected Lactarius pseudomucidus, Phialocephala fortinii, Piloderma fallax,
and Tomentella sublilacina that were not observed above ground. In addition,
sequence data from the current study indicated the presence Piloderma croceum,
Russula fragilis, and Russula cascadensis at our sites. These species are documented
ectomycorrhizal partners with Western Hemlock, and our findings suggest
that they may have wider distributions and might also associate with other tree
species, including Eastern Hemlock.
Following GenBank searches, we listed a total of 38 unique sequences as
unknown fungal clones. We labeled the sequences in this manner due to either
inconsistent identifications or clade support expressed as inconsistent library identifications
and possible undetermined chimeric insertions (K. Hughes, University of
Tennessee, Knoxville, TN, pers. comm.). Unknown fungal clone sequences always
Southeastern Naturalist
229
R. Baird, et al.
2014 Vol. 13, Special Issue 6
had low similarity and coverage values, making any attempt to provide names
for those taxa unsupported. In many cases, the GenBank database showed strong
similarity and coverage with multiple sequences identified as an uncultured fungus
clone. Future comparisons of the current data with the GenBank database could
provide more accurate names for these fungi. Walker et al. (2005, 2008) reported
that many clone sequences could not be identified, but that re-examination of the
data from those studies using updated GenBank information might provide a better
understanding of the fungal populations in those ecosystems.
Although cultural isolation revealed limited diversity, we detected a number of
taxa that were not obtained in root-tissue sequences. Saprobic species identified exclusively
from cultural isolations included Pestalotiopsis maculiformans, Rhizopus
arrhizus, and 6 species of Trichoderma (teleomorph = Hypocrea), a genus known
to have important ecological roles in soil. Trichoderma species are considered important
cellulose-degrading microorganisms that can limit or inhibit the growth of
other microorganisms (Harman 2000). Some species are known to be antagonistic
or biological control agents of bacteria and other fungi (Chaverri and Samuels
2004). Trichoderma was dominant in isolates obtained from root tissue during this
study and accounted for 80% of all isolates. This study is in agreement with others
in showing high saprobic fungal diversity, including those in the commonly isolated
Ascomycota and Zygomycota (Lynch and Thorn 2006, Porter et al. 2008).
We did not observe many of the taxa commonly associated with Hemlock in
other studies of younger regenerating stands (e.g., Cenococcum species) (Wright et
al. 2009). This result may indicate that mature Hemlock trees like those we sampled
have different fungal associates than juvenile trees. Additionally, results by Walker
et al. (2008) documented the seasonality of root-associated fungal communities and
indicated that ectomycorrhizal communities in forest systems cannot be adequately
characterized based on samples taken at a single time in the growing season.
This study provides valuable forest-health baseline data that will be useful in
monitoring changes in the fungal community associated with declining and oldgrowth
Eastern Hemlock forests. While the levels of fungal diversity we report rank
among the highest recorded for any temperate host-species or habitat using prepyrosequence
data, overall diversity estimates from more recent studies indicate
that we under-sampled total fungal diversity and density (Jumpponen et al. 2010).
The fact that we collected the root samples for sequence analysis from shallow soil
adjacent to the bole of the tree after removal of the litter surface may also have
affected our fungal community data. Saprobic species numbers were low overall
except in the cultural study. The lack of additional DNA sampling from woody debris
and the forest litter layer likely limited the detection of taxa that occur on these
substrates.
In spite of the limitations detailed above, the high diversity of beneficial fungi
and large number of species collected infrequently during the study have important
implications for conservation of biodiversity in Hemlock forests. This is an
important finding from the viewpoint of conservation biology and adds further
value to these forests as centers of biological diversity. However, further research
Southeastern Naturalist
R. Baird, et al.
2014
230
Vol. 13, Special Issue 6
is necessary to discover how mycorrhizal fungal communities will facilitate forest
succession by altering the competitive balance between tree species that replace
Hemlock (Lewis et al. 2008). In addition, research is needed to uncover the ecological
requirements and roles of rare, difficult-to-identify, and host-specific fungi.
High-throughput sequencing and culturing approaches could help further elucidate
the complex microbial ecosystems occupied by these diverse fungi, especially in
forests such as Eastern Hemlock stands, that are being eliminated from riparian
zones and moist upland habitats.
Acknowledgments
Appreciation is extended to Highlands Biological Station for financial support as grantsin-
aid to Mark Alexander during 2007 and 2008; to the GRSM, National Park Service,
Department of the Interior for logistical and technical support of the project; to David Pratt
for use of laboratory and housing facilities at the Tennessee University Field Station; and
to Mississippi State University (MAFES publication number 12344) for use of laboratory
facilities and supplies not covered by grants.
Literature Cited
Altschul, S.F., T.L. Madden, A.A. Schäffer, J. Zhang, Z. Zhang, W. Miller, and D.J. Lipman.
1997. Gapped BLAST and PSI-BLAST: A new generation of protein database search
programs. Nucleic Acids Research. 25:3389–3402.
Baird, R., L. Wallace, G. Baker, and M. Scruggs. 2013. Stipitate hydnoid fungi of the temperate
southeastern United States. Fungal Diversity 62:41–114.
Baird, R.E., M. Alexander, B. Stokes, A. Wood-Jones, C. Watson, T. Remaley, G. Taylor, K.
Johnson, and S. Diehl. 2014. Fleshy ectomycorrhizal fungal community associated with
health and declining Eastern Hemlock stands in Great Smoky Mountains National Park.
Southeastern Naturalist Special Issue XX:xxx–xxx
Barnett, H.L., and B.B. Hunter. 1987. Illustrated Genera of Imperfect Fungi. 4th Edition.
Macmillian Publishing Company, New York, NY. 218 pp.
Brant, D.L. 1988. A study of the Hymenoscyphus monotropae, a fungus associated with
Monotropa uniflora. Thesis in Biology, Frostburg State University, Frostburg, MD.
124 pp.
Bruns, T., J. Tan, M. Bidartondo, T. Szaro, and D. Redecker. 2002. Survival of Suillus
pungens and Amanita francheti ectomycorrhizal genets was rare or absent after a standreplacing
wildfire. New Phytologist 155:517–523.
Burke, D.J., J.C. López-Gutierrez, K.A. Smemo, and C.R. Chan 2009. Vegetation and
soil environment influence the spatial distribution of root-associated fungi in a mature
beech-maple forest. Applied and Environmental Microbiology 75:7639–7648.
Challen, M.P., R.W. Kerrigan, and P. Callac. 2003. A phylogenetic reconstruction and
emendation of Agaricus section Duploannulata. Mycologia 95:61–73.
Chaverri P., and G.J. Samuels. 2004. Hypocrea/Trichoderma (Ascomycota, Hypocreales,
Hypocreaceae): Species with green ascospores. Study Mycology 48:1–116.
DeBellis, T., G. Kernaghan, R. Bradley, and P. Widden. 2006. Relationships between stand
composition and ectomycorrhizal community structure in boreal mixed-wood forests.
Microbial Ecology 99:114–126.
Southeastern Naturalist
231
R. Baird, et al.
2014 Vol. 13, Special Issue 6
Dickie, I.A., B. Dentinger, P. Avis, and D.P. McLaughlin. 2009. Ectomycorrhizal fungal
communities of oak savanna are distinct from forest communities. Mycologia
101:473–483.
Dighton, J., and A.F. Harrison. 1983. Phosphorus nutrition of Lodgepole Pine and Sitka
Spruce stands as indicated by a root bioassay. Forestry 56:33–43.
Dyke, F.V. 2003. Conservation Biology: Foundations, Concepts, Applications. McGraw-
Hill. New York, NY. 414 pp.
Ellis, M.B. 1971. Dematiaceous Hyphomycetes. Commonwealth Mycological Institute,
KEW, Surrey, UK. 608 pp.
Fortin, J.A., and Y. Piché. 1979. Cultivation of Pinus strobus root-hypocotyl explants for
synthesis of ectomycorrhiza. New Phytologist 83:109–119.
Goodman, D.M., D.M. Durall, J.A. Trofymow, and S. Berch. 1996–1998. A Manual of Concise
Descriptions of North American Ectomycorrhizae, 1st–4th Editions. Mycologue,
Sidney, NS, Canada.
Hambleton, S., and L. Sigler. 2005. Meliniomyces, a new anamorph genus for root-associated
fungi with phylogenetic affinities to Rhizoscyphus eriacae (= Hymenoscyphus
ericae), Leotiomycetes. Studies in Mycology 53:1–27.
Harman, G.E. 2000. Myths and dogmas of biocontrol. Changes in perceptions derived from
research on Trichoderma harzianum T22. Plant Disease 84:377–393.
Horton, T.R., and T.D. Bruns. 2001. The molecular revolution in ecomycorrhizal ecology:
Peeking into the black-box. Molecular Ecology 10:1855–1871.
Hoshino Y.T., and N. Matsumoto. 2007. Changes in fungal community structure in bulk
soil and spinach rhizosphere soil after chemical fumigation as revealed by 18S rDNA
PCR-DGGE. Soil Science and Plant Nutrition 53:40–55.
Hughes, K.W., R.H. Petersen, and E.B. Lickey. 2009. Using heterozygosity to estimate
a percentage DNA sequence similarity for environmental species’ delimitation across
basidiomycete fungi. New Phytologist 182:795–798.
Johnson, K., T. Remaley, and G. Taylor. 2008. Managing Hemlock Woolly Adelgid at Great
Smoky Mountains National Park: Situation and response. Pp. 189–196, In B. Onken and
R. Reardon (Eds.). Proceedings Fourth Symposium on Hemlock Woolly Adelgid in the
eastern United States. FHTET-2008-01, USDA Forest Service, Forest Health Technology
Enterprise TEAM, Morgantown, WV. 222 pp.
Jumpponen, A. 2007. Soil fungal communities underneath willow canopies on a primary
successional glacier forefront: rDNA sequence results can be affected by primer selection
and chimeric data. Microbial Ecology 53:233–246.
Jumpponen, A., and J.M. Trappe. 1998. Dark septate endophytes: A review of facultative
biotrophic root colonizing fungi. New Phytologist 140:29–310.
Jumpponen, A., K.L. Jones, D. Mattox, and C. Yaege. 2010. Massively parallel 454-sequencing
of fungal communities in Quercus spp. ectomycorrhizas indicates seasonal
dynamics in urban and rural sites. Molecular Ecology (Suppl. 1) 19:41–53.
Kennedy, N., and N. Clipson. 2003. Fingerprinting the fungal community. Mycologist
17:158–164
Kropp, B R., and J.M. Trappe. 1982. Ectomycorrhizal fungi of Tsuga heterophylla. Mycologia
74:479–488.
Landeweert, R., P. Leeflang, T.W. Kuyper, E. Hoffland, A. Rosling, K. Wernars, and E.
Smit. 2003. Molecular identification of ectomycorrhizal mycelium in soil horizons. Applied
and Environmental Microbiology 69:327–333.
Southeastern Naturalist
R. Baird, et al.
2014
232
Vol. 13, Special Issue 6
Lewis J.D., J. Licitra, A.R. Tuininga, A. Sirulnik, G.D. Turner, and J. Johnson. 2008. Oak
seedling growth and ectomycorrhizal colonization are less in Eastern Hemlock stands
infested with Hemlock Woolly Adelgid than in adjacent oak stands. Tree Physiology
28:629–636.
Lilleskov, E.A., E.A. Hobbie, and T.J. Fahey. 2002. Ectomycorrhizal fungal taxa differing
in response to nitrogen deposition also differ in pure culture organic nitrogen use and
natural abundance of nitrogen isotopes. New Phytologist 154:219–231.
Lynch, M.D.J., and R.G. Thorn. 2006. Diversity of basidiomycetes in Michigan agricultural
soils. Applied and Environmental Microbiology 72:7050–7056.
Magurran, A.E. 2004. Measuring Biological Diversity. Blackwell Publishing: Oxford, UK.
256 pp.
Mata, J.L., K. Hughes, R.H. Petersen. 2007. An investigation of Omphalotaceae (Fungi:
Euagarics) with emphasis on the genus Gymnopus. Syndowia 58:191–289.
Mayer, M., R. Chianese, T. Scudder, J. White, K. Vongpaseuth, and R. Ward. 2002. Thirteen
years of monitoring the Hemlock Woolly Adelgid in New Jersey forests. Pp. 50–60, In
B. Onken, R. Reardon, and J. Lashomb. (Eds.). Hemlock Woolly Adelgid in the Eastern
United States Symposium Proceedings, 5–7 February 2002, Rutgers University, East
Brunswick, NJ.
McClure, M.S., S. Salom, and K.S. Shields. 2001. Hemlock Wooly Adelgid. Forest Health
Technology Enterprise Team, US Forest Service Publication FHTET–2001– 03. Morgantown,
WV. 14 pp.
McLenon-Porter, T.M. 2008. Above and below ground fungal diversity in a hemlockdominated
forest plot in southern Ontario and the phylogenetic placement of a new
Ascomycota phylum (Dissertation). Library and Archives Canada. 470 pp.
Monreal, M.A., S.M. Berch, and M. Berbee. 1999. Molecular diversity of ericoid mycorrhizal
fungi. Canadian Journal of Botany 77:1580–1594.
Orwig, D.A. 2002. Stand dynamics associated with chronic Hemlock Woolly Adelgid infestation
in southern New England. Pp. 36–46, In B. Onken, R. Reardon, and J. Lashomb
(Eds.). Hemlock Woolly Adelgid in the Eastern United States Symposium Proceedings,
5–7 February 2002, Rutgers University, East Brunswick, NJ.
Pearson, V., and D.J. Read. 1973. The biology of mycorrhiza in the Ericaceae I. The isolation
of the endophyte and synthesis of mycorrhizas in aseptic culture. New Phytologist
72:371–379.
Porter, T.M., C.W. Schadt, L. Rizui, A.P. Martin, S.K. Schmidt, L. Scott-Denton, R. Vilgalys,
and J.M. Moncalvo. 2008. Widespread occurrence and phylogenetic placement
of a soil clone group adds a prominent new branch to the fungal tree of life. Molecular
Phylogenetics and Evolution 46:635–644.
Price, P.W. 1997. Insect Ecology, Third Edition. Wiley, New York, NY. 874 pp.
Reynolds, W.N. 2008. Imidacloprid insecticide treatments for Hemlock Woolly Adelgid,
Adelges tsugae Annand (Hemiptera: Adelgidae), affect a non-target soil arthropod community
surrounding Eastern Hemlock, Tsuga canadensis (L.) Carriere. M.Sc. Thesis.
The University of Tennessee, Knoxville, TN. 125 pp.
Roy, K.W., R.E. Baird, and T.S. Abney. 2001. A review of soybean (Glycine max) seed, pod,
and flower mycofloras in North America, with methods and a key for identification of
selected fungi. Mycopathologia 150:15–27.
SAS Institute. 1999. SAS/STAT software changes and enhancements through release V8.
SAS Institute, Cary, NC.
Schomaker, M.E., S.J. Zarnoch, W.A. Bechtold, D.J. Latelle, W.G. Burkman, and S.M. Cox.
2007. Crown condition classification: A guide to data collection and analysis. General
Technical Report SRS-102, US Department of Agriculture, Forest Service, Southern
Research Station, Asheville, NC. 78 pp.
Southeastern Naturalist
233
R. Baird, et al.
2014 Vol. 13, Special Issue 6
Sharkey, M.J. 2001. The All Taxa Biological Inventory of the Great Smoky Mountains
National Park. Florida Entomologist 84:556–564.
Stephenson, S.L. 1989. Distribution and ecology of myxomycetes in temperate forests II.
Patterns of occurrence on bark surface of living trees, leaf litter, and dung. Mycologia
81:608–621.
Stephenson, S.L., M. Schnittler, and C. Lado. 2004. Ecological characterization of tropical
myxomycete assemblage-Maquipucuna Cloud Forest Reserve, Equador. Mycologia
96:488–497.
Sutton, S.C. 1980. Coelomycetes. Commonwealth Mycological Institute, Kew, Surrey, UK.
696 pp.
US Department of Agriculture, Forest Service (USDA). 1965. Silvics of Forest Trees of the
United States. H.A. Fowells (Ed.). Agriculture Handbook 271. Washington, DC. 762 pp.
Villarroel, D.A., R.E. Baird, L.E. Trevathan, C.E. Watson, and M.L. Scruggs. 2004. Pod
and seed mycoflora on transgenic and conventional soybean (Glycine max) (L.) Merrill
cultivars in Mississippi. Mycopathologia 157:207–215.
Vohnik, M, M. Fendrych, J. Albrechtova, and M. Vosatka. 2007. Intracellular colonization
of Rhododendron and Vaccinium roots by Cenococcum geophilum, Geomyces pannorum,
and Meliniomyces variabilis. Folia Microbiologicia. 52:407–414.
Walker, J.F., O.K. Miller, Jr., and J.L. Horton. 2005. Hyperdiversity of ectomycorrhizal
fungus assemblages on oak seedlings in mixed forests in the southern Appalachian
Mountains. Molecular Ecology 14:829–838.
Walker, J.F., O.K. Miller, Jr., and J.L. Horton. 2008. Seasonal dynamics of ectomycorrhizal
fungus assemblages on oak seedlings in the southeastern Appalachian Mountains.
Mycorrhiza 18:123–132.
Watling, R. 1987. How to Identify Mushrooms to Genus V: Cultural and Developmental
Features. Mad River Press, Eureka, CA. 169 pp.
Weiss, M., R. Bauer, and D. Begerow. 2004. Spotlight on heterobasidiomycetes. Pp. 7–48,
In R. Agerer, M. Pieponbring, and P. Blantz (Eds.) Frontiers in Basidiomycete Mycology.
IHW Verlag, Eching, Germany.
White, T.J., T. Brun, and L.S. Taylor. 1990. Amplification and direct sequencing of fungal
ribosomal RNA genes for phylogenetics. Pp. 315–322, In M.A. Innis, D.H. Gelfand,
J.J.Sninsky, and T.J. White (Eds.). PCR Protocols: a Guide to Methods and Applications.
Academic Press, New York, NY.
Wilcox, H.E., and C.J.K. Wang. 1987. Ectomycorrhizal and ectendomycorrhizal associations
of Phialophora landia with Pinus resinosa, Picea rubens, and Betula alleghaniensis.
Canadian Journal Forest Research 17:976–990.
Wright, S.H.A., S.M. Berch, and M.L. Berbee. 2009. The effect of fertilization on the below-
ground diversity and community composition of ectomycorrhizal fungi associated
with Western Hemlock (Tsuga heterophylla). Mycorrhiza 19:267–276.
Southeastern Naturalist
R. Baird, et al.
2014
234
Vol. 13, Special Issue 6
Appendix A. Frequency and relative abundance of fungi associated with roots of Eastern
Hemlock in Great Smoky Mountains National Park as determined by molecular techniques.
Abundance values are based on mean percent relative abundance of fungal taxa from 2 sites:
Copeland Creek (CC; 487 m) and Gabes Mountain (GM; 1158 m). Abundance = total number
clones per species ÷ 2000 (= 2 sites x 20 trees x 50 clones) x 100. The National Center
of Biotechnology Institute (NCBI) accession numbers listed are based on ≥98% sequence
homology over >80% coverage of fungal taxa in the GenBank database. Taxa with less than
80% homology and under 70% coverage are designated Unknown. Freq. = mean percent
occurrence of fungal taxa per root sample, each collected from a single Eastern Hemlock
tree (mean percent ÷ 40 (= 2 sites x 20 trees) x 100. Overall abundance values are based on
mean percent relative abundance of total fungal taxa identified from Eastern Hemlock root
samples (= total mean percent relative abundance from all treatments ÷ 124 unique taxa).
Abundance
by site
NCBI Overall
Fungal taxa accession Freq. CC GM abund.
Ascomycota
Ascomycota sp. KF359553 6.5 <1.0 0.0 1.1
Uncultured Ascomycete clone 1 KF359554 9.6 <1.0 3.3
Unknown Ascomycete clone 2 KF359555 3.2 1.7 0.0 1.5
Cenococcum geophilum Fr. KF359556 3.2 0.0 <1.0 <1.0
Chalara microchona W. Gams KF359557 3.2 0.0 0.0 <1.0
Chaunopycnis alba W. Gams KF359558 3.2 0.0 <1.0 <1.0
Cladophialophora chaetospira (Grove) KF359559 16.1 3.2 <1.0 3.4
Crous & Arzanlou
Elaphomyces sp. KF359560 3.2 0.0 <1.0 <1.0
Fusidium sp. KF359561 3.2 0.0 <1.0 <1.0
Uncultured Helotiaceae 1 KF359562 3.2 <1.0 0.0 <1.0
Uncultured Helotiaceae 2 KF359563 16.0 1.0 <1.0 4.9
Uncultured Helotiales clone 1 KF359564 25.7 3.0 1.0 4.8
Uncultured Helotiales clone 2 KF359565 3.2 0.0 <1.0 <1.0
Uncultured Helotiales clone 3 KF359566 6.4 1.0 0.0 1.3
Uncultured Helotiales clone 4 KF359567 6.4 <1.0 <1.0 <1.3
Uncultured ecto (Helotiales) KF359568 3.2 0.0 <1.0 <1.0
Uncultured Hyaloscypaceae contains KF359569 3.2 0.0 <1.0 <1.0
Lachnum spp.
Hymenoscyphus monotropae Kernan & KF359570 3.2 0.0 <1.0 <1.0
Finocchio
Ilyonectria radicicola (Gerlach & L. KF359571 6.5 <1.0 0.0 <1.0
Nilsson) P. Chaverri & Salgado (= Neonectria radicicola)
Lachnum virgineum (Batsch.) P. Karst KF359572 6.4 <1.0 <1.0 <1.3
Uncultured Leotiomycetes 1 KF359573 9.7 2.6 0.0 2.3
Uncultured Leotiomycota clone KF359574 3.2 0.0 <1.0 <1.0
Uncultured Leotiomycetes clone 2 KF359575 6.4 <1.0 0.0 <1.5
Uncultured Leotiomycetes clone 3 KF359576 9.7 2.6 3.0 3.3
Uncultured Magnaportheaceae KF359577 3.2 0.0 <1.0 <1.0
Meliniomyces variabilis Hambl. & Sigler KF359578 22.6 <1.0 <1.0 1.2
Southeastern Naturalist
235
R. Baird, et al.
2014 Vol. 13, Special Issue 6
Abundance
by site
NCBI Overall
Fungal taxa accession Freq. CC GM abund.
Uncultured Oidiodendron clone KF359579 3.2 0.0 <1.0 <1.0
Oidiodendron maius G.L. Barron for KF359580 3.2 0.0 <1.0 <1.0
ericaceous mycorrhizae and saprobe by A. Rice and R. Currah
Oidiodendron sp. 1 KF359581 3.2 <1.0 0.0 <1.0
Oidiodendron sp. 2 KF359582 6.5 0.0 2.0 1.6
Penicillium spinulosum Thom KF359583 3.2 0.0 <1.0 <1.0
Penicillium simplicissimum (Oudem.) KF359584 3.2 <1.0 0.0 <1.0
Thom
Uncultured Pezizomycotina KF359585 3.2 1.7 0.0 1.6
Phialocephala fortinii C.J.K. Wang & KF359586 9.7 <1.0 <1.0 160
H.E. Wilcox
Rhytismataceae sp. clone KF359587 6.4 <1.0 2.7 2.1
Trichoderma asperellum Samuels, KF359588 6.5 0.0 <1.0 <1.0
Lieckf. & Nirenberg
Venturaceae KF359589 12.9 2.1 <1.0 2.8
Basidiomycota
Amanita cf. constricta Theirs & Ammirati KF359590 9.7 1.7 <1.0 2.0
Clavulina cinerea (Bull.) J. Schröt. KF359591 6.4 0.0 1.3 1.8
Clavulina cristata (Holmsk.) J. Schröt. KF359592 6.5 2.6 0.0 2.3
Clavulina sp. KF359593 3.2 0.0 <1.0 <1.0
Unknown Cortinariaceae clone KF359594 12.9 0.0 1.4 1.5
Herpotrichiellaceae sp. 1 KF359595 6.5 0.0 <1.0 <1.0
Galerina sp. KF359596 3.2 0.0 <1.0 <1.0
Lactarius chrysorrheus Fr. KF359597 3.2 <1.0 0.0 <1.0
Lactarius cf. gerardii Peck KF359598 3.2 0.0 <1.0 <1.0
Lactarius pseudomucidus Hesler & KF359599 3.2 1.7 0.0 1.3
A.H. Sm.
Lactarius tabidus Fr. KF359600 6.4 1.0 0.0 1.2
Mycena sp. 1 KF359601 3.2 <1.0 0.0 <1.0
Mycena sp. 2 KF359602 3.2 0.0 <1.0 <1.0
Mycena sanguinolenta (Alb. & Schwein.) KF359603 3.2 <1.0 0.0 <1.0
P. Kumm.
Mycena silvae-nigrae Maas Geest. & KF359604 3.2 0.0 <1.0 <1.0
Schwobel
Piloderma byssinum (P. Karst.) Jülich KF359605 9.7 1.7 0.0 1.7
Piloderma croceum J. Erikss. & Hjortstam KF359606 3.2 <1.0 0.0 1.1
Piloderma fallax (Lib.) Stalpers KF359607 6.5 <1.0 <1.0 1.3
Piloderma olivaceum (Parmasto) Hjortstam KF359608 3.2 <1.0 0.0 1.2
Piloderma sp. 1 KF359609 6.4 1.3 0.0 1.5
Piloderma sp. 2 KF359610 3.2 2.6 0.0 1.9
Piloderma sp. 3 KF359611 16.1 <1.0 3.4 3.6
Piloderma sp. 4 KF359612 3.2 <1.0 0.0 <1.0
Piloderma sp. 5 KF359613 3.2 <1.0 0.0 <1.0
Southeastern Naturalist
R. Baird, et al.
2014
236
Vol. 13, Special Issue 6
Abundance
by site
NCBI Overall
Fungal taxa accession Freq. CC GM abund.
Rhodocollybia butyracea (Bull.) Lennox KF359614 3.2 <1.0 0.0 <1.0
Russula atropurpurea Peck KF359615 6.5 0.0 5.0 3.2
Russula casadensis Shaffer KF359616 9.6 <1.0 1.3 1.7
Russula fragilis Fr. KF359617 3.2 0.0 <1.0 <1.0
Russula granulata voucher Peck KF359618 16.1 2.6 0.0 2.5
Russula sp. 1 KF359619 3.2 0.0 <1.0 <1.0
Russula sp. 2 KF359620 3.2 <1.0 <1.0 1.6
Sebacinales sp. 1 KF359621 9.7 0.0 <1.0 <1.0
Sebacinales sp. 2 KF359622 6.5 0.0 <1.0 <1.0
Tomentella sp. 1 KF359623 9.7 <1.0 <1.0 <1.0
Tomentella sp. 2 KF359624 6.4 <1.0 <1.0 1.2
Tomentella sublilacina (Ellis & Holw.) KF359625 9.7 <1.0 2.0 2.3
Wakef.
Xeromphalina aff. parvibulbosa KF359626 3.2 <1.0 0.0 <1.0
(Kauffman & A.H. Sm.) Redhead
Uncultured ecto (Agaricales) KF359627 3.2 <1.0 0.0 1.0
Uncultured ecto (Cortinariaceae) KF359628 3.2 0.0 <1.0 <1.0
Uncultured Basidiomycota clone 1 KF359629 3.2 0.0 <1.0 <1.0
Uncultured Basidiomycota clone 2 KF359630 3.2 <1.0 0.0 <1.0
Uncultured Basidomycete clone 3 KF359631 3.2 <1.0 <1.0 1.2
Uncultured Basidiomycete clone 4 KF359632 3.2 <1.0 0.0 <1.0
Basidiomycete sp. KF359633 19.2 <3.4 <1.0 <4.9
Glomeromycota
Glomeromycete sp. KF359634 6.4 <1.0 <1.0 1.1
Unknown fungi
Unknown uncultured ectomycorrhizal sp. 1 KF359635 3.2 0.0 <1.0 <1.0
Unknown uncultured ectomycorrhizal sp. 2 KF359636 6.5 <1.0 0.0 <1.0
Unknown ectomycorrhizal clone 1 KF359637 3.2 0.0 <1.0 <1.0
Unknown ectomycorrhizal clone 2 KF359638 3.2 1.7 0.0 1.6
Unknown fungus clone 1 KF359639 9.6 3.1 0.0 2.6
Unknown fungus clone 2 KF359640 3.2 <1.0 0.0 <1.0
Unknown fungus clone 3 KF359641 12.8 3.7 0.0 3.3
Unknown fungus clone 4 KF359642 6.4 <1.0 <1.0 1.6
Unknown fungus clone 5 KF359643 3.2 <1.0 0.0 <1.0
Unknown fungus clone 6 KF359644 3.2 <1.0 0.0 <1.0
Unknown fungus clone 7 KF359645 3.2 <1.0 0.0 1.0
Unknown fungus clone 8 KF359646 3.2 <1.0 0.0 <1.0
Unknown fungus clone 9 KF359647 3.2 0.0 <1.0 <1.0
Unknown fungus clone 10 KF359648 3.2 <1.0 0.0 <1.0
Unknown fungus clone 11 KF359649 3.2 0.0 <1.0 <1.0
Unknown fungus clone 12 KF359650 3.2 <1.0 0.0 <1.0
Unknown fungus clone 13 KF359651 9.6 <1.0 1.4 1.8
Southeastern Naturalist
237
R. Baird, et al.
2014 Vol. 13, Special Issue 6
Abundance
by site
NCBI Overall
Fungal taxa accession Freq. CC GM abund.
Unknown fungus clone 14 KF359652 3.2 <1.0 0.0 <1.0
Unknown fungus clone 15 KF359653 9.6 1.2 <1.0 <2.6
Unknown fungus clone 16 KF359654 6.4 <1.0 <1.0 1.6
Unknown fungus clone 17 KF359655 3.2 0.0 <1.0 <1.0
Unknown fungus clone 18 KF359656 3.2 <1.0 0.0 <1.0
Unknown fungus clone 19 KF359657 3.2 <1.0 0.0 <1.0
Unknown fungus clone 20 KF359658 3.2 <1.0 0.0 <1.0
Unknown fungus clone 21 KF359659 9.6 0.0 5.2 4.2
Unknown fungus clone 22 KF359660 3.2 <1.0 0.0 <1.0
Unknown fungus clone 23 KF359661 3.2 0.0 <1.0 <1.0
Unknown fungus clone 24 KF359662 3.2 0.0 <1.0 <1.0
Unknown fungus clone 25 KF359663 3.2 0.0 <1.0 <1.0
Unknown fungus clone 26 KF359664 3.2 0.0 <1.0 <1.0
Unknown fungus clone 27 KF359665 3.2 <1.0 0.0 <1.0
Unknown fungus clone 28 KF359666 3.2 0.0 <1.0 <1.0
Unknown fungus clone 29 KF359667 3.2 <1.0 0.0 <1.0
Unknown fungus clone 30 KF359668 3.2 1.7 0.0 1.3
Unknown fungus clone 31 KF359669 3.2 0.0 <1.0 <1.0
Unknown fungus clone 32 KF359670 6.4 <1.0 <1.0 <1.0
Unknown fungus clone 33 KF359671 3.2 0.0 <1.0 <1.0
Unknown fungus clone 34 KF359672 3.2 <1.0 0.0 <1.0
Unknown fungus clone 35 KF359673 3.2 <1.0 0.0 <1.0
Unknown fungus clone 36 KF359674 3.2 0.0 <1.0 <1.0
Unknown fungus clone 37 KF359675 3.2 0.0 <1.0 <1.0
Unknown fungus clone 38 KF359676 6.4 <1.0 <1.0 1.6