Differential Responses to Light and Nutrient Availability by
Geographically Isolated Poison Ivy Accessions
John G. Jelesko, Elise B. Benhase, and Jacob N. Barney
Northeastern Naturalist, Volume 24, Issue 2 (2017): 191–200
Full-text pdf (Accessible only to subscribers. To subscribe click here.)
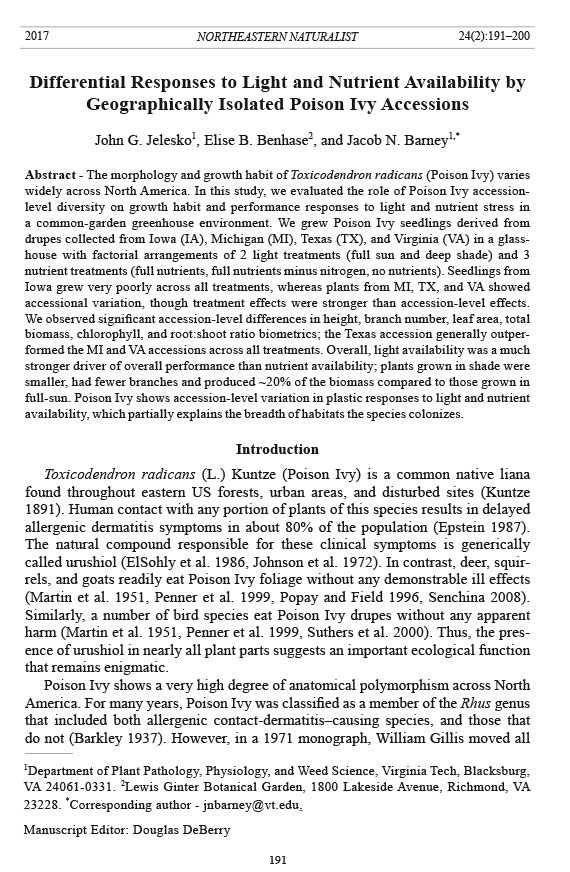
Access Journal Content
Open access browsing of table of contents and abstract pages. Full text pdfs available for download for subscribers.
Current Issue: Vol. 30 (3)
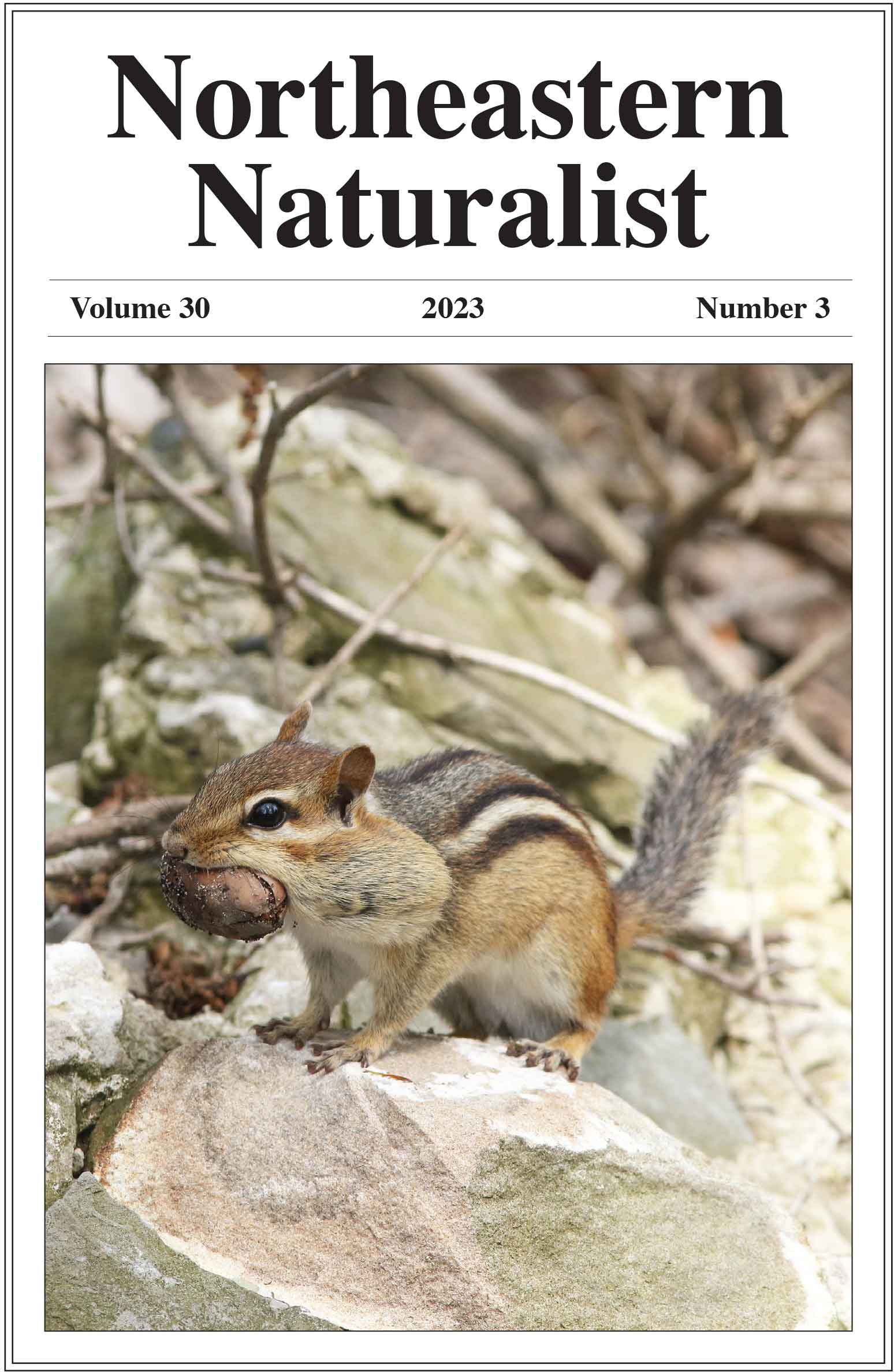
Check out NENA's latest Monograph:
Monograph 22
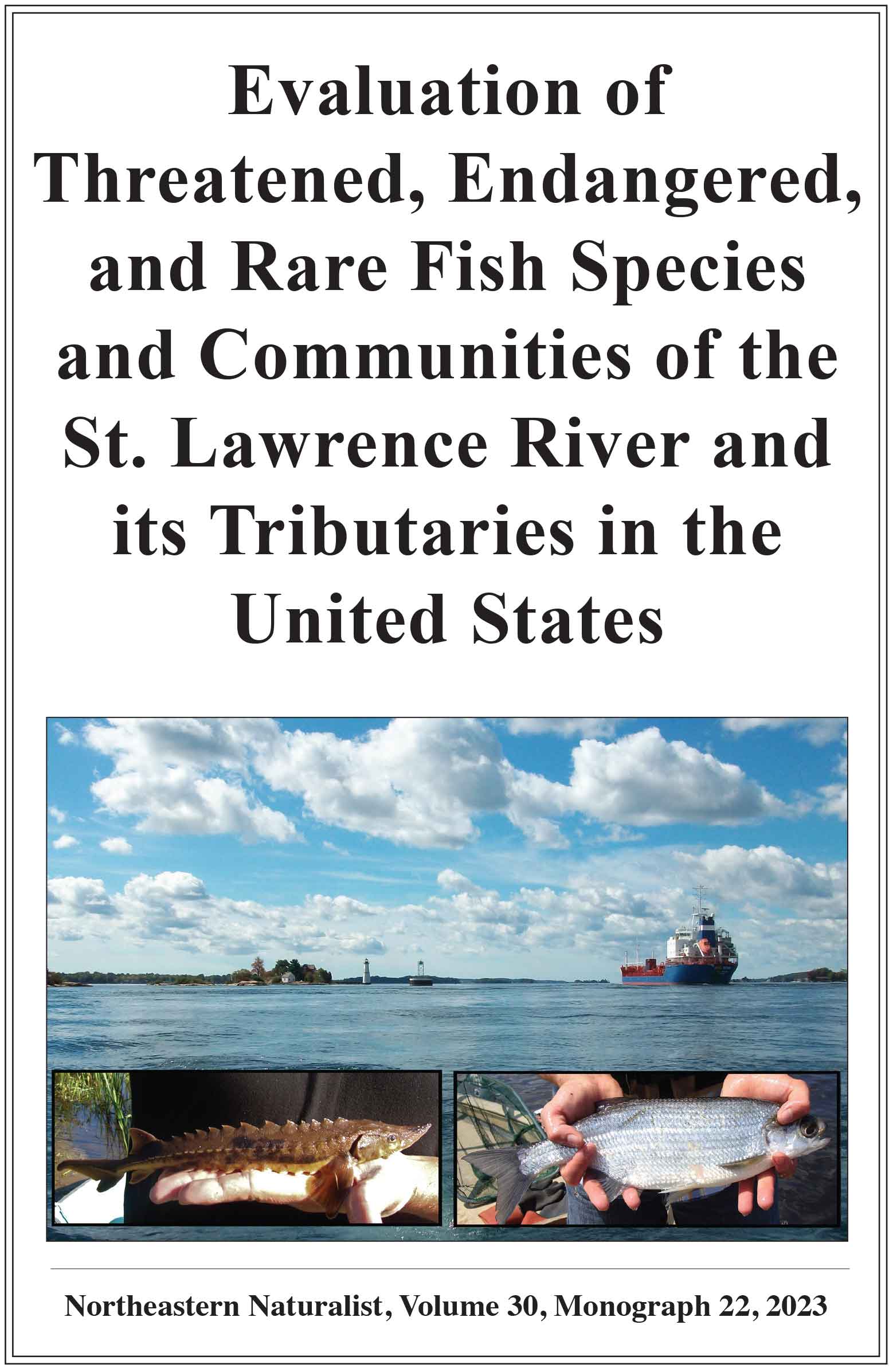
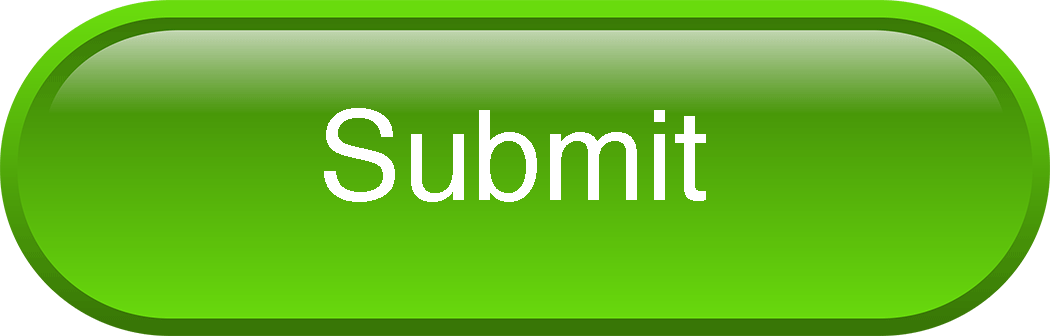
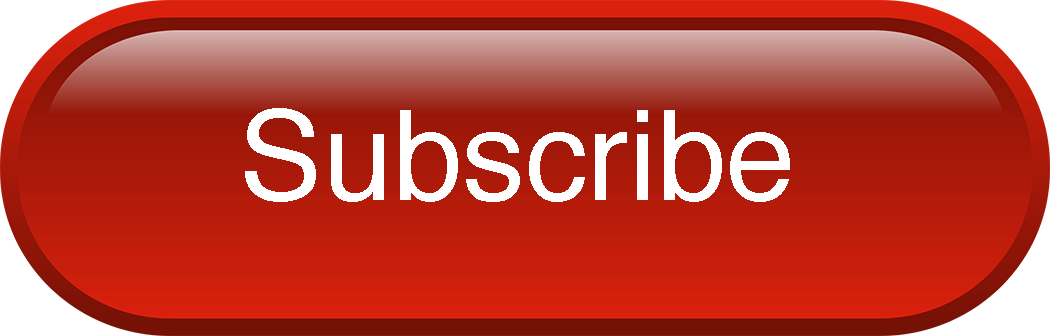
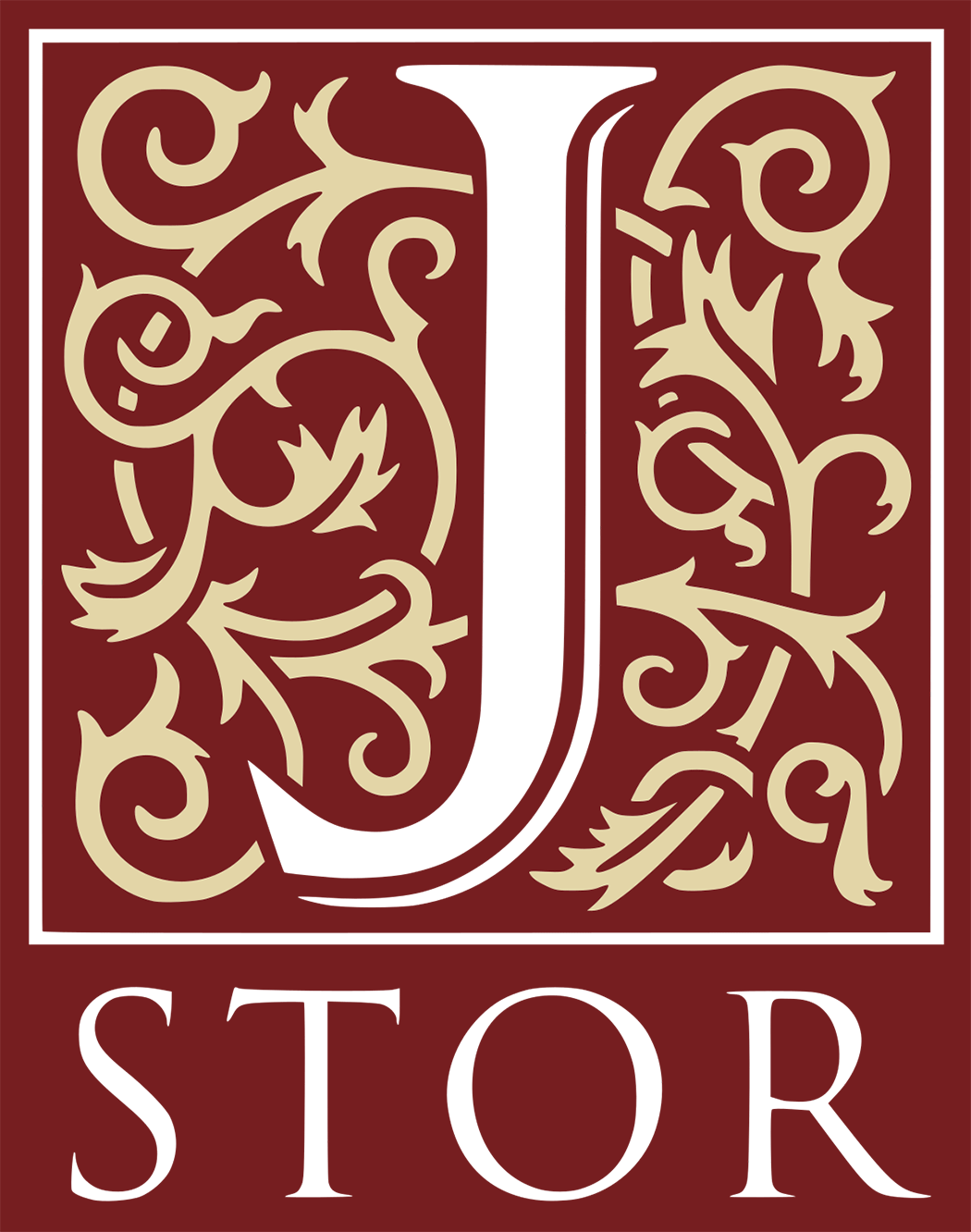


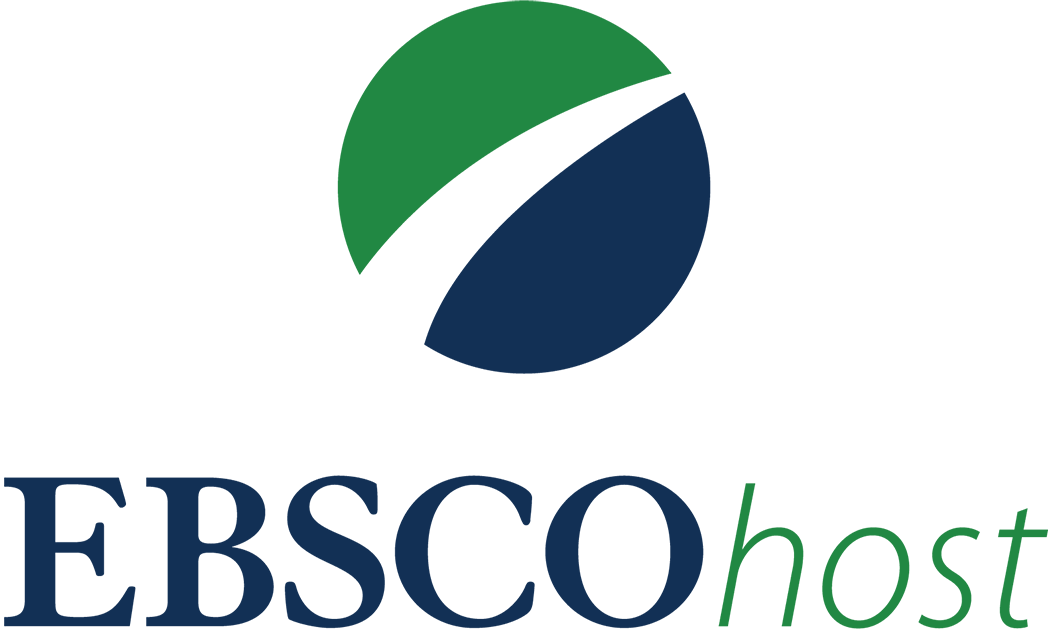

Northeastern Naturalist Vol. 24, No. 2
J.G. Jelesko, E.B. Benhase, and J.N. Barney
2017
191
2017 NORTHEASTERN NATURALIST 24(2):191–200
Differential Responses to Light and Nutrient Availability by
Geographically Isolated Poison Ivy Accessions
John G. Jelesko1, Elise B. Benhase2, and Jacob N. Barney1,*
Abstract - The morphology and growth habit of Toxicodendron radicans (Poison Ivy) varies
widely across North America. In this study, we evaluated the role of Poison Ivy accessionlevel
diversity on growth habit and performance responses to light and nutrient stress in
a common-garden greenhouse environment. We grew Poison Ivy seedlings derived from
drupes collected from Iowa (IA), Michigan (MI), Texas (TX), and Virginia (VA) in a glasshouse
with factorial arrangements of 2 light treatments (full sun and deep shade) and 3
nutrient treatments (full nutrients, full nutrients minus nitrogen, no nutrients). Seedlings from
Iowa grew very poorly across all treatments, whereas plants from MI, TX, and VA showed
accessional variation, though treatment effects were stronger than accession-level effects.
We observed significant accession-level differences in height, branch number, leaf area, total
biomass, chlorophyll, and root:shoot ratio biometrics; the Texas accession generally outperformed
the MI and VA accessions across all treatments. Overall, light availability was a much
stronger driver of overall performance than nutrient availability; plants grown in shade were
smaller, had fewer branches and produced ~20% of the biomass compared to those grown in
full-sun. Poison Ivy shows accession-level variation in plastic responses to light and nutrient
availability, which partially explains the breadth of habitats the species colonizes.
Introduction
Toxicodendron radicans (L.) Kuntze (Poison Ivy) is a common native liana
found throughout eastern US forests, urban areas, and disturbed sites (Kuntze
1891). Human contact with any portion of plants of this species results in delayed
allergenic dermatitis symptoms in about 80% of the population (Epstein 1987).
The natural compound responsible for these clinical symptoms is generically
called urushiol (ElSohly et al. 1986, Johnson et al. 1972). In contrast, deer, squirrels,
and goats readily eat Poison Ivy foliage without any demonstrable ill effects
(Martin et al. 1951, Penner et al. 1999, Popay and Field 1996, Senchina 2008).
Similarly, a number of bird species eat Poison Ivy drupes without any apparent
harm (Martin et al. 1951, Penner et al. 1999, Suthers et al. 2000). Thus, the presence
of urushiol in nearly all plant parts suggests an important ecological function
that remains enigmatic.
Poison Ivy shows a very high degree of anatomical polymorphism across North
America. For many years, Poison Ivy was classified as a member of the Rhus genus
that included both allergenic contact-dermatitis–causing species, and those that
do not (Barkley 1937). However, in a 1971 monograph, William Gillis moved all
1Department of Plant Pathology, Physiology, and Weed Science, Virginia Tech, Blacksburg,
VA 24061-0331. 2Lewis Ginter Botanical Garden, 1800 Lakeside Avenue, Richmond, VA
23228. *Corresponding author - jnbarney@vt.edu.
Manuscript Editor: Douglas DeBerry
Northeastern Naturalist
192
J.G. Jelesko, E.B. Benhase, and J.N. Barney
2017 Vol. 24, No. 2
allergenic Rhus members into the unified genus Toxicodendron, and extensively
described Poison Ivy and Toxicodendron diversilobum (Torr. & A. Gray) Greene
(Poison Oak) biogeography (Gillis 1971). He assigned 4 major species within the
genus: Toxicodendron diversilobum (Torr. & A. Gray) Greene (Western Poison
Oak), T. toxicarium (Salisb.) (Eastern Poison Oak), T. rydbergii (Small ex Rydb.)
Greene (Western Poison Ivy), and T. radicans (Poison Ivy). Based upon both
biogeography and a variety of traits, Gillis further subdivided T. radicans into a
number of subspecies: barkleyi, divaricatum, eximium, hispidum, negundo, pubens,
radicans, and verrucosum. Gillis also described apparent intergrade zones with
presumed hybrids showing intermediate traits. The latter suggests localized genetic
adaption of isolated Poison Ivy populations.
Poison Ivy is often purported to be associated with edge habitats, particularly
those formed by managed lands. Upon closer examination, these assertions seem
to be derived from anecdotal observations, rather than systematic investigations.
Gillis examined the Baker Woodlot in East Lansing, MI, for expected preferential
T. radicans ssp. negundo (Greene) growth at the edges of the woodland; however,
he concluded that light availability alone could not account for the observed patterns
of Poison Ivy presence and absence at the woodland edges (Gillis 1971). Gillis
speculated that the natural range of poison ivy and poison oak species may have
dramatically expanded in response to historical European immigrant land-management
patterns. For example, records from Spanish colonization of California during
the 16th and 17th centuries did not mention allergenic poison oak as a native plant
species, suggesting it was relatively rare during this period. In contrast, Western
Poison Oak is a widespread and common weedy species in California today. Poison
Ivy is adapted to exploit a variety of habitats because it can grow as a shrub, creeping
stolon, and/or a liana (Gillis 1971), suggesting high phenotypic plasticity. In
contrast, Western Poison Ivy diplays only shrub and creeping-stolon growth habits.
The multiplicity of poison ivy growth-habit capacity enables it to rapidly respond
to both natural (Allen et al. 1997, Allen 2005) and anthropogenic disturbances
(Catling et al. 2002, Gillis 1971). The species’ adaptive traits include rapid growth
rate with accompanying accumulation of biomass and shifting urushiol congener
levels to more-allergenic urushiol-congener composition in response to elevated
CO2 (Mohan et al. 2006, Ziska et al. 2007).
Despite the widespread incidence of poison ivy in North America, its ecology is
poorly understood, particularly regarding performance responses to common environmental
stressors. With the anticipation of increased land-use change and continued
habitat fragmentation, an understanding of how Poison Ivy responds to environmental
variation will aid characterization of how this native invader will respond to further
anthropogenic changes. Therefore, our objective was to determine if Poison Ivy exhibits
intra-specific variation in response to light and nutrient availability.
Materials and Methods
We evaluated accession-level variation in stress response by 4 Poison Ivy plants
representing a wide geographical distribution: Roanoke County, VA (37°22'52.5"N,
Northeastern Naturalist Vol. 24, No. 2
J.G. Jelesko, E.B. Benhase, and J.N. Barney
2017
193
80°06'39.2"W), collected in August 2012; Basrop County, TX (30°104.5588"N,
97°24'11.019"W), collected in September 2012; Dallas County, IA (New York
Botanical Garden herbarium barcode 02111147; 41°39'26.4954"N, 94°0'27.9714"
W), collected in September 2013; and Ingram County, MI (42°45'7.8696"N,
84°35'7.8684"W) collected in October 2013. No herbarium samples were prepared
from the original female IA, MI, and VA lianas at the time the drupes were collected.
Instead we prepared herbarium samples from seedlings generated from
seeds removed from IA, MI, and VA drupes, and we deposited vouchers in the
Virginia Tech Herbarium (specimen #109163, #109162, #109165, respectively).
A Texas seedling-vouchered specimen #109164 was also submitted to the Virginia
Tech Herbarium. We did not identify the 4 lianas to subspecies. We stored drupes
in paper bags at room temperature until the experiment began. In March 2014, we
mechanically and chemically scarified (Benhase and Jelesko 2013) and planted
in soilless Metro-Mix media (Sun Gro, Agawam, MA) all seeds; seedlings were
grown under ambient conditions in a ventilated glasshouse located at the Virginia
Tech Glade Road Research facility in Blacksburg, VA. Germinating seeds were
kept moist with overhead irrigation and given no additional nutrients. Three weeks
after germination, we transferred the Poison Ivy seedlings to 184-cm3 square pots
containing leached Metro-Mix media and kept the growing medium moist.
Two of the most common abiotic environmental stressors are light and nutrient
limitations. Therefore, we employed a factorial design to examine 2 levels of light
availability and 3 levels of nutrient availability. On 28 April 2014, we randomly assigned
seedlings to either full sun or shade environments, which were implemented
as split blocks with greenhouse benches serving as whole blocks. We constructed
shade environments to mimic light environments of a typical deciduous forest
understory of ~10% of ambient light, which was achieved by placing plants under
shade cloth supported by PVC frames. Poison Ivy is often found climbing on
adjacent supports (e.g., trees); thus, we trained the primary stem of each seedling
around a bamboo stake placed in the center of the pot throughout the experiment.
We watered the seedlings in sufficient quantity that water was non-limiting
throughout the experiment.
We imposed 3 nutrient treatments within each light environment sub-plot: (1) no
additional nutrients (control), (2) full macro- and micro-nutrient solution (full + N),
and (3) full macro- and micro-nutrients minus nitrogen (full - N). The nutrient treatments
were applied as full Hoagland solution (Hoagland and Arnon 1950) (full + N)
or a Hoagland without nitrogen (full - N) and delivered as 100 ml to each pot twice
a week. We established 4 whole blocks, with each block containing both light treatments,
and 2 replications of each accession in each nutrient treatment. Therefore,
there were a total of 48 individuals per accession (2 reps x 3 nutrient treatments x
2 light environments x 4 blocks).
During the course of the experiment, we recorded the following growth
and performance metrics bi-monthly: height, branch number, leaf number, and
chlorophyll-content proxy using the Opti-Sciences, CCM-300 (Hudson, NH). In
September 2014, after 20 weeks of growth under the experimental conditions, we
Northeastern Naturalist
194
J.G. Jelesko, E.B. Benhase, and J.N. Barney
2017 Vol. 24, No. 2
determined biomass by harvesting leaves, stems, branches, and roots from each
seedling and then weighing the samples after they had dried at 70 °C for 1 week
until mass was constant. Before drying, we used a LI-3100 Area Meter (LI-COR
Environmental, Lincoln, NE) to determine total leaf area for each individual.
We employed ANOVA to assess our data and considered final height (cm), leaf
and branch number, chlorophyll content (mg m-2), leaf area (cm2), specific leaf area
(cm2 g-1), total biomass (g), and root-to-shoot biomass ratio as response variables
and Poison Ivy accession, light environment, and nutrient treatment as fixed effects.
Higher-order interactions were backward-stepwise removed to improve
model fit. Response variables were transformed as necessary to meet assumptions
of normality of residuals and equal variance. Height, leaf area, and biomass were
log10 transformed, and specific leaf area and root:shoot ratio were square-root
transformed. All statistical analyses were performed in SAS JMP version 12 (Martin-
Rodrigues 2013).
Results
Seeds from all 4 geographically isolated Poison Ivy accessions readily germinated
after mechanical and chemical scarification. Seedlings from the Michigan,
Texas, and Virginia accessions produced a first true-leaf pair and subsequent alternate
true leaves. In contrast, the Iowa seedlings grew extremely poorly across
all treatments, all individuals produced relatively few true leaves, and never progressed
beyond that small growth-stage in any treatment throughout the remainder
of the experiment. We did not include data from the Iowa accession in the statistical
analyses because the growth habit of these seedlings was dramatically different
from the other 3 accessions.
The Texas, Michigan, and Virginia Poison Ivy accessions performed consistently
for most traits among treatments. There were significant accession-level effects
on Poison Ivy plant height (P < 0.0001), leaf area (P < 0.0001), root:shoot biomass
ratio (P < 0.0001; Fig. 1b), total biomass (P < 0.0001), and chlorophyll levels (P =
0.0122) (Table 1). We observed significant light-environment effects for all parameters,
except chlorophyll content (Table 1). We found significant effects in Poison
Ivy response to the light environment for plant height (P = 0.0003), branch number
(P < 0.0001; Fig. 2c), leaf area (P < 0.0001), specific leaf-area (P < 0.0001, Fig.
1c), root:shoot biomass (P = 0.0225), and total biomass (P < 0.0001). Significant
interactions between accession and light environment were only observed for leaf
area and plant height (Table 1); the Texas accession was consistently taller than the
Virginia and Michigan accessions (Fig. 2a).
Nutrient availability also affected Poison Ivy biometrics (Table 1). Branch
number (P < 0.0001), leaf area (P < 0.0001), total biomass (P < 0.0001), and chlorophyll
content (P = 0.0024) were all strongly affected by the nutrient content.
The greatest effect appeared to be in response to nitrogen availability, rather than
mineral micronutrients (Fig. 2d). Nutrient availability interacted strongly with the
light environment for leaf area (P < 0.0001), total biomass (P < 0.0001; Fig. 1a),
chlorophyll content (P < 0.0497; Fig. 2b), and weakly interacted for plant height
Northeastern Naturalist Vol. 24, No. 2
J.G. Jelesko, E.B. Benhase, and J.N. Barney
2017
195
(P < 0.0766). Interaction between nutrient availability and accession-level effects
was limited to a weak interaction with chlorophyll content (P < 0.0841).
Table 1. Statistical model P-values for performance metrics assessed at the end of the experiment.
We used backwards step-wise regression to improve model fit. Model parameters with a dash (-)
were not included in the model for that metric. A = accession, L = light environment, and N = nutrient
availability.
Leaf Specific Root/ Total
Height Branch # area leaf area shoot biomass Chlorophyll
A less than 0.0001 0.7297 less than 0.0001 0.2162 less than 0.0001 less than 0.0001 0.0122
L 0.0003 less than 0.0001 less than 0.0001 less than 0.0001 0.0225 less than 0.0001 0.9772
A*L 0.0245 - 0.0428 - - - -
N 0.1331 less than 0.0001 less than 0.0001 0.3611 0.2157 less than 0.0001 0.0024
A*N - - - - - - 0.0841
L*N 0.0766 - less than 0.0001 - - less than 0.0001 0.0497
A*L*N - - - - - - -
Figure 1. Treatment differences for Poison Ivy (a) total biomass as a function of nutrient
treatment and light environment,( b) root-to-shoot ratio as a function of accession, and (c)
specific leaf area as a function of light environment. See Table 1 for statistical analyses.
Northeastern Naturalist
196
J.G. Jelesko, E.B. Benhase, and J.N. Barney
2017 Vol. 24, No. 2
Discussion
Poison Ivy is a disturbance-adapted native liana that appears to quickly colonize
areas affected by both natural and anthropogenic disturbance. In 1989, hurricane
Hugo caused extensive storm damage to an old-growth forest in South Carolina,
resulting in widespread localized opening of the forest canopy due to downed trees.
The destruction of many large trees and their associated Poison Ivy lianas, resulted
in an initial decrease in Poison Ivy incidence (Allen et al. 1997). However, within
12 years Poison Ivy reestablished to higher incidence levels than before hurricane
Hugo (Allen et al. 2005). This situation suggests that Poison Ivy was likely light
limited. Here, we show that Poison Ivy growth (height and biomass) was significantly
greater in full light compared to shade. Poison Ivy also responded favorably
to nutrient additions; nitrogen was the strongest limiting nutrient. Both responses
Figure 2. Treatment differences for Poison Ivy (a) height as a function of accession and light
environment, (b) leaf chlorophyll content [UNITS?] as a function of light environment and
nutrient treatment, (c) branch number as a function of light environment, and (d) branch
number as a function of nutrient treatment. See Table 1 for statistical analyses.
Northeastern Naturalist Vol. 24, No. 2
J.G. Jelesko, E.B. Benhase, and J.N. Barney
2017
197
are consistent with vigorous growth following habitat disturbances that increase
exposure to direct sunlight and reduce resource competition. Interestingly, we observed
that 4 Poison Ivy accessions collected from across its US distribution varied
in their response to stress.
Fire (either natural or anthropogenic) is another type of habitat disturbance
that increases both light and mineral-nutrient availability (Kutiel and Shaviv
1992). Poison Ivy incidence is positively associated with small fires in forest
environments (Catling 2002). Similarly, Eastern Poison Oak is more prevalent
in areas subjected to recurrent small fires (Gillis 1971); however, the exact environmental
component responsible for the positive association with these burned
environments was not identified. Gillis (1971) noted that Poison Ivy generally
favors nutrient-rich soils with adequate drainage. The Gillis monograph summarized
results from hydroponic experiments with Poison Ivy in which either iron
or nitrate deficiency resulted in chlorosis, and calcium was critical for poison
ivy growth and viability. In our study, the Michigan, Texas, and Virginia Poison
Ivy accessions showed significant increases in total biomass and branching in response
to nitrogen (full + N vs. full-N treatments); total biomass also increased in
response to other mineral nutrients (full - N vs. control treatment).
Interestingly, we found that Poison Ivy performance was notably enhanced
when nitrogen was available in the full-sun environment, while biomass production
was similar in the shade among all nutrient treatments. Leaf-chlorophyll
content was higher in full-sun plants, but only in nitrogen-fertilized plants; leafchlorophyll
content was lower in full sun than shade when all nutrients but nitrogen
were supplied. The shade stress was not magnified by low nutrient-availability as
expected; rather low light-availability reduced overall biomass production, which
was not enhanced by nitrogen application. Disturbance is often associated with an
increase in resource availability (e.g., nutrients, light), which is known to increase
susceptibility to invasion (Davis et al. 2000). From our results, we predict that, following
common forest disturbances that result in canopy gaps or opening of forest
interiors, Poison Ivy will be particularly well suited to capitalizing on both light
and nutrient availability. Current and future poison ivy invasiveness may continue
to be enhanced as a result of an “anthropogenically induced adaptation to invade”
(Hufbauer et al. 2012).
There is a remarkable degree of phenotypic diversity in poison ivy populations
across North America (Barkley 1937, Gillis 1971). To what degree this
phenotypic diversity is a manifestation of either innate phenotypic plasticity
(i.e., the general-purpose genotype) or genetic adaptation of local populations is
an important topic worthy of further research. Gillis (1971) identified suspected
hybrid zones comprising populations with intermediate phenotypes. He also
made presumed interspecific crosses that yielded individuals with intermediate
morphological characters. Although these results indicate the presence of genetically
differentiated populations, differences in morphological characters did not
indicate whether the 2 parental Poison Ivy populations were differentially adapted
for specific ecophysiological traits. Our study found Poison Ivy exhibited significant
accession-level differential growth-responses, such as plant height, leaf
Northeastern Naturalist
198
J.G. Jelesko, E.B. Benhase, and J.N. Barney
2017 Vol. 24, No. 2
area, chlorophyll level, total biomass, and root:shoot biomass. Moreover, there
was significant accession-level variation in Poison Ivy plant height and leaf area
in response to the light environment, and weak interaction between nutrient availability
and chlorophyll content. These findings indicate that Poison Ivy phenotypic
diversity extends beyond morphological characters, and includes presumed genetic
adaptation of biometric ecophysiological traits that are likely important for the species’
success in specific disturbed or native habitats. Our conclusions might have
been stronger if we had determined the taxonomic subspecies of each of the 4 lianas
that gave rise to the 4 geographically isolated drupe accessions. Thus, future studies
using a broader diversity of phytogeographical accessions (with T. radicans ssp.
characterization) are needed to characterize the degree to which poison ivy exhibits
local adaptation across diverse habitat types (Atwater et al. 2016).
The observed poor growth of the Iowa accession was unexpected. We were unable
to determine the environmental parameter that restricted the vigor of the Iowa
accession in these experiments. Gillis (1971) reported that calcium deficiency has
a profound effect limiting poison ivy growth and viability. In the present work,
we used Hoagland’s solution, which contains calcium, as the base fertilizer; yet,
neither full + N nor full - N fertilizer treatments improved overall growth of the
Iowa accession relative to the control treatment (no Hoagland’s). Thus, the poor
vigor of the Iowa plants was a consistent accession-level effect, likely caused by
an underlying genetic deficiency that was not displayed in the other 3 Poison Ivy
accessions. Clearly, Poison Ivy accessions vary in their growth form and response
to environmental conditions.
Lianas are known to have a variety of ecological impacts due to their structurally
parasitic growth form and rapid response to disturbance (Horvitz and Koop 2001).
Lianas have been shown to outcompete other plant species, reduce seedling recruitment,
and reduce ecosystem carbon dynamics (Horvitz and Koop 2001). Thus, it is
important that we better understand liana ecology in this era of rapid global change.
Here we show that the native weedy vine, Poison Ivy, responds positively to light
and nutrient availability in a non-additive fashion—the presence of both light and
nitrogen enhanced overall performance more than either alone. Furthermore, there
was significant intra-specific variation in the observed plastic responses. Thus,
phenotypic plasticity enables Poison Ivy to capitalize on increased-resource availability,
which commonly follows habitat disturbance.
Acknowledgments
We thank Dan Atwater, Eugene Dollete, and Dan Tekiela for assistance in harvesting
plant tissue for the experiment. The Poison Ivy drupes from Iowa, Michigan, and Texas
were kindly provided by Andy Schmitz, Hope Ann Rankin (Michigan State University), and
Daniel Atha (New York Botanical Garden).
Literature Cited
Allen, B.P., E.F. Pauley, and R.R. Sharitz. 1997. Hurricane impacts on liana populations in
an old-growth southeastern bottomland forest. Journal of the Torrey Botanical Society
124:34–42.
Northeastern Naturalist Vol. 24, No. 2
J.G. Jelesko, E.B. Benhase, and J.N. Barney
2017
199
Allen, B.P., R.R. Sharitz, and P.C. Goebel. 2005. Twelve years post-hurricane liana dynamics
in an old-growth southeastern floodplain forest. Forest Ecology and Management
218:259–269.
Atwater, D., U. Sezen, V.H. Goff, W. Kong, A. Paterson, and J.N. Barney. 2016. Reconstructing
changes in the genotype, phenotype, and climatic niche of an introduced species.
Ecography 39:894–903.
Barkley, F.A. 1937. A monographic study of Rhus and its immediate allies in North and
Central America, including the West Indies. Annals of the Missouri Botanical Garden
37:265–498.
Benhase, E.B., and J.G. Jelesko. 2013. Germinating and culturing axenic poison ivy seedlings.
HortScience 48:1–5.
Catling, P.M., A. Sinclair, and D. Cuddy. 2002. Plant community composition and relationships
of disturbed and undisturbed alvar woodland. Canadian Field-Naturalist
116:571–579.
Davis, M.A., J. P. Grime, and K. Thompson 2000. Fluctuating resources in plant communities:
A general theory of invasibility. Journal of Ecology 88:528–534..
ElSohly, M.A., P.D. Adawadkar, D.A. Benigni, E.S. Watson, and T.L. Little Jr. 1986.
Analogues of poison ivy urushiol: Synthesis and biological activity of disubstituted nalkylbenzenes.
Journal of Medicinal Chemistry 29:606–611.
Epstein, W.L. 1987. Plant-induced dermatitis. Annals of Emergency Medicine 16:950–955.
Gillis, W.T. 1971. The systematics and ecology of poison-ivy and the poison-oaks (Toxicodendron,
Anacardiaceae). Rhodora 73:72–159, 161–237, 370–443, 465–540.
Hoagland, D.R., and D.I. Arnon. 1950. The water-culture method for growing plants without
soil. University of California, College of Agriculture, Agricultural Experiment Station,
Berkeley, CA.
Horvitz, C.C., and A. Koop. 2001. Removal of nonnative vines and post-hurricane recruitment
in tropical hardwood forests of Florida. Biotropica 33:268–281.
Hufbauer, R.A., B. Facon, V. Ravigné, J. Turgeon, J. Foucaud, C.E. Lee, O. Rey, and A.
Estoup. 2012. Anthropogenically induced adaptation to invade (AIAI): Contemporary
adaptation to human-altered habitats within the native range can promote invasions,
Evolutionary Applications, 5(1):89–101
Johnson, R.A., H. Baer, C.H. Kirkpatrick, C.R. Dawson, and R.G. Khurana. 1972. Comparison
of the contact allergenicity of the four pentadecylcatechols derived from poison
ivy urushiol in human subjects. Journal of Allergy and Clinical Immunology 49:27–35.
Kuntze, O. 1891. Revisio Generum Plantarum. Arthur Feliz, Liepzig, Germany. [# PP?].
Kutiel, P., and A. Shaviv. 1992. Effects of soil type, plant composition, and leaching on soil
nutrients following a simulated forest-fire. Forest Ecology and Management 53:329–343.
Martin, A.C., H.S. Zim, and A.L. Nelson. 1951. American Wildlife and Plants. Dover Publications,
Inc., New York, NY.
Martin-Rodrigues, N., S. Espinel, J. Sanchez-Zabala, A. Ortiz, C. Gonzalez-Murua, and
M.K. Dunabeitia. 2013. Spatial and temporal dynamics of the colonization of Pinus radiata
by Fusarium circinatum, of conidiophora development in the pith and of traumatic
resin-duct formation. New Phytologist 198:1215–1227.
Mohan, J.E., L.H. Ziska, W.H. Schlesinger, R.B. Thomas, R.C. Sicher, K. George, and J.S.
Clark. 2006. Biomass and toxicity responses of Poison Ivy (Toxicodendron radicans)
to elevated atmospheric CO2. Proceedings of the National Academy of Sciences of the
United States of America 103:9086–9089.
Northeastern Naturalist
200
J.G. Jelesko, E.B. Benhase, and J.N. Barney
2017 Vol. 24, No. 2
Penner, R., G.E.E. Moodie, and R.J. Staniforth. 1999. The dispersal of fruits and seeds of
Poison-ivy, Toxicodendron radicans, by Ruffed Grouse, Bonasa umbellus, and squirrels,
Tamiasciurus hudsonicus and Sciurus carolinensis. Canadian Field-Naturalist
113:616–620.
Popay, I., and R. Field. 1996. Grazing animals as weed-control agents. Weed Technology
10:217–231.
Senchina, D.S. 2008. Fungal and animal associates of Toxicodendron spp. (Anacardiaceae)
in North America. Perspectives in Plant Ecology Evolution and Systematics 10:197–216.
Suthers, H.B., J.M. Bickal, and P.G. Rodewal. 2000. Use of successional habitat and fruit
resources by songbirds during autumn migration in central New Jersey. Wilson Bulletin
112:249–260.
Ziska, L.H., R.C. Sicher, K. George, and J.E Mohan. 2007. Rising atmospheric carbon
dioxide and potential impacts on the growth and toxicity of Poison Ivy (Toxicodendron
radicans). Weed Science 55:288–292.