Potential Influence of Salamanders and Coarse Woody
Debris on the Distribution of Dryopteris intermedia in a
Hardwood Forest
Devin G. Oralls, Abbey R. Osborn, and Jack T. Tessier
Northeastern Naturalist, Volume 23, Issue 1 (2016): 151–162
Full-text pdf (Accessible only to subscribers. To subscribe click here.)
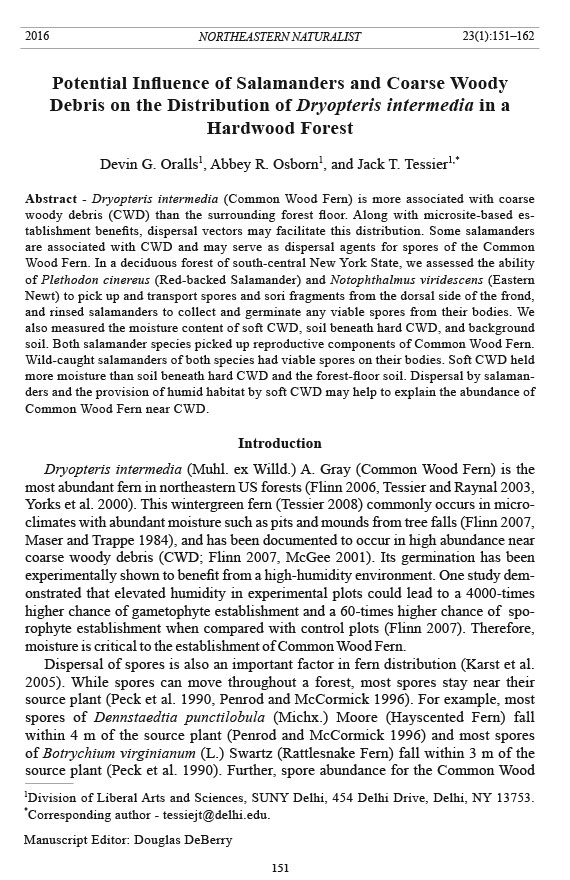
Access Journal Content
Open access browsing of table of contents and abstract pages. Full text pdfs available for download for subscribers.
Current Issue: Vol. 30 (3)
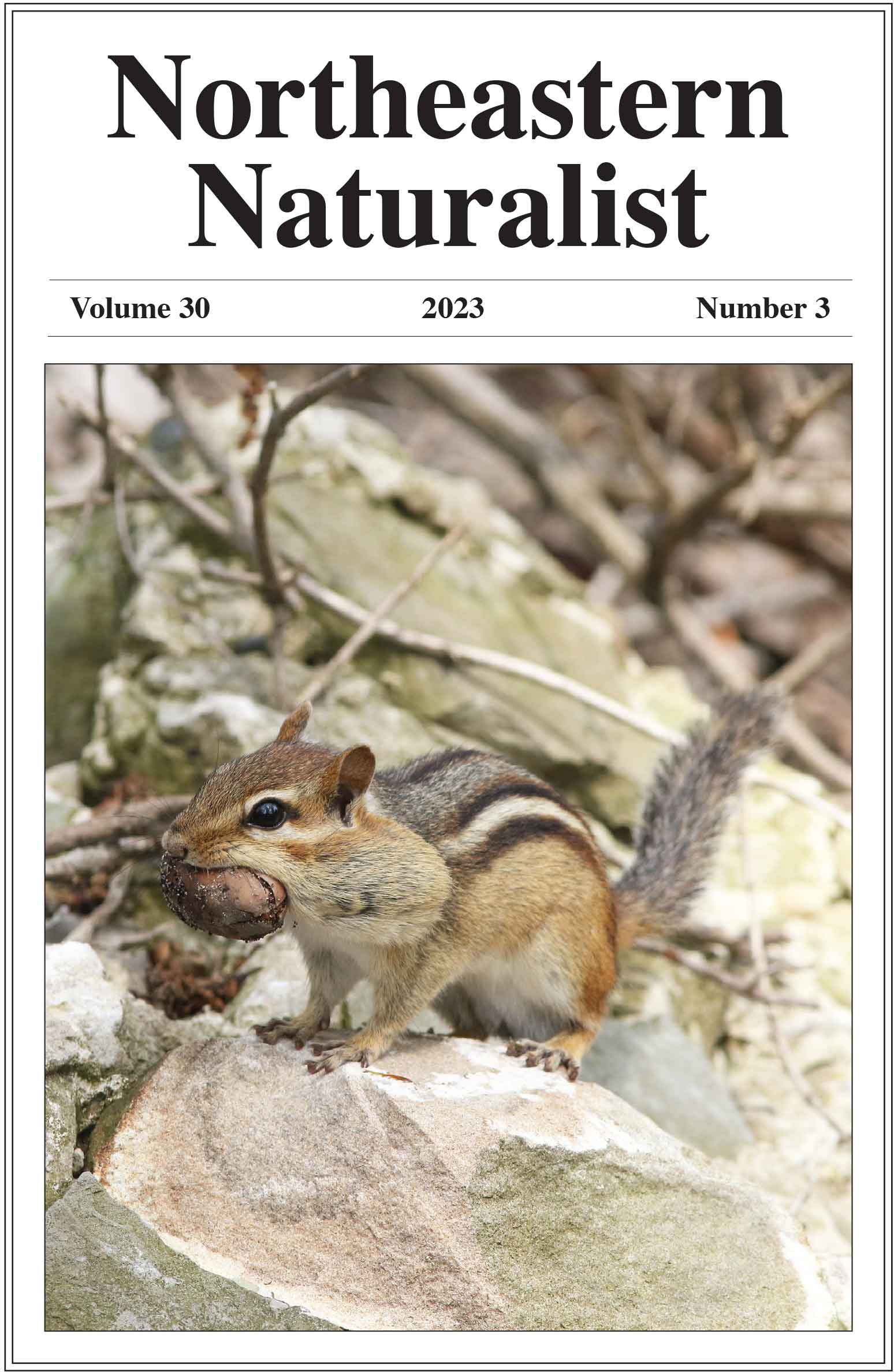
Check out NENA's latest Monograph:
Monograph 22
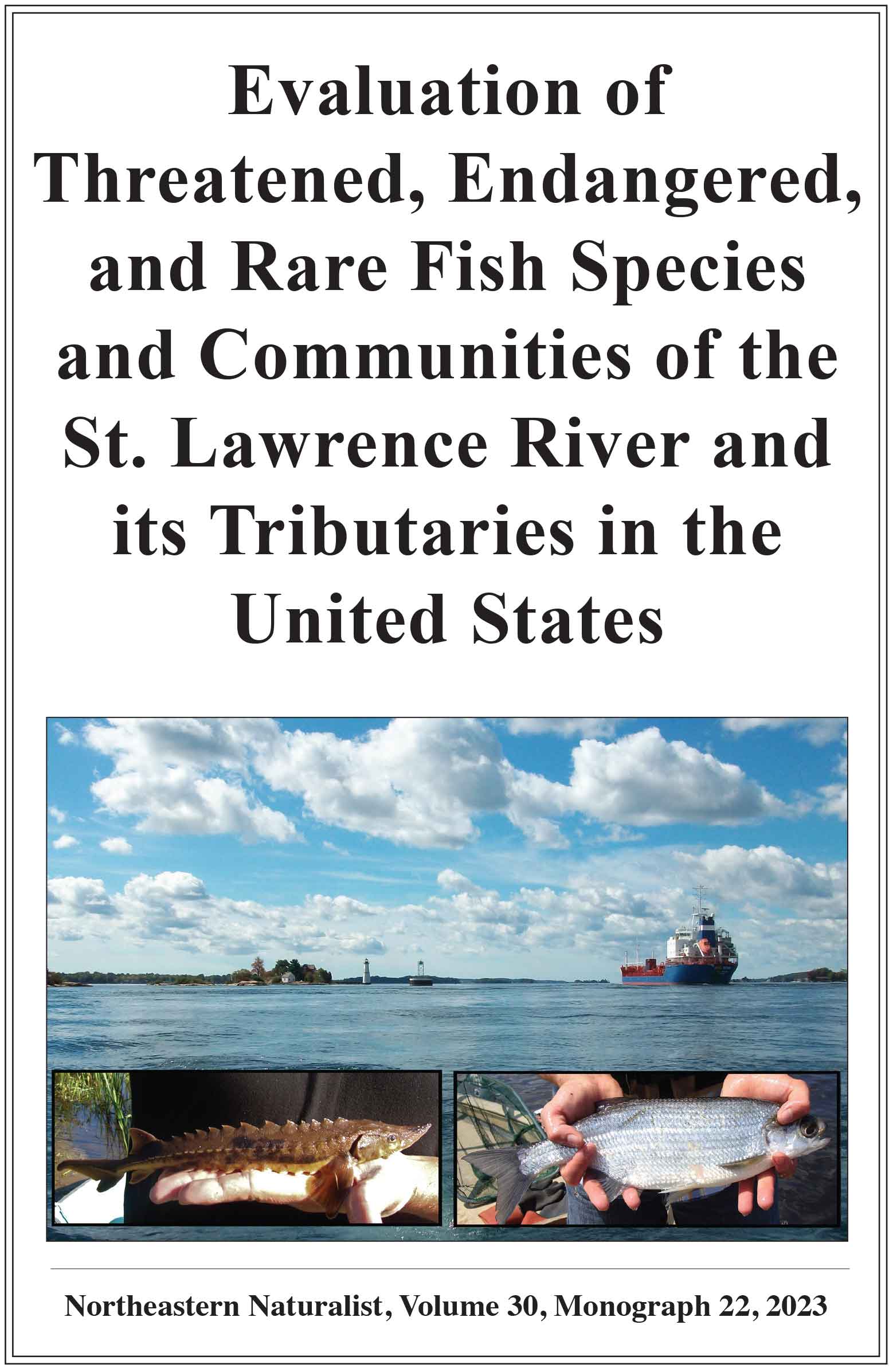
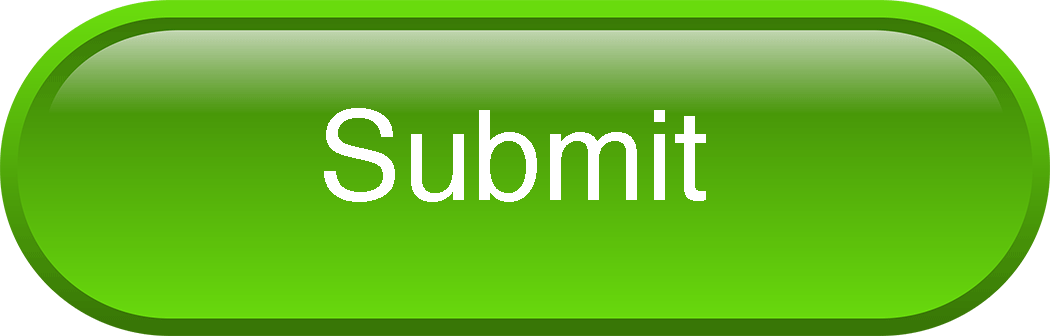
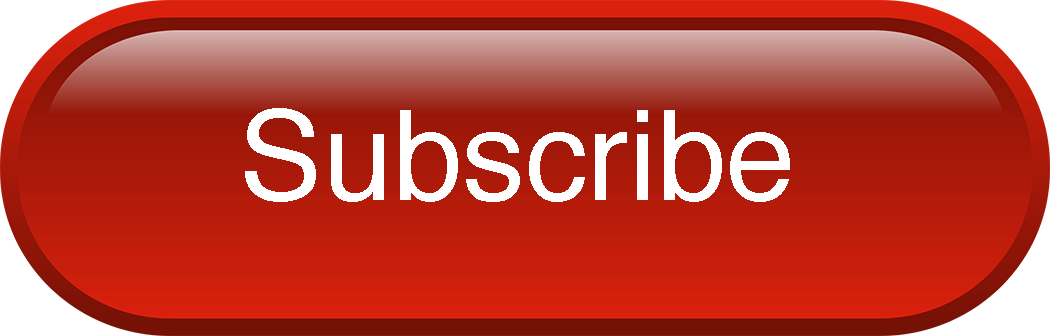
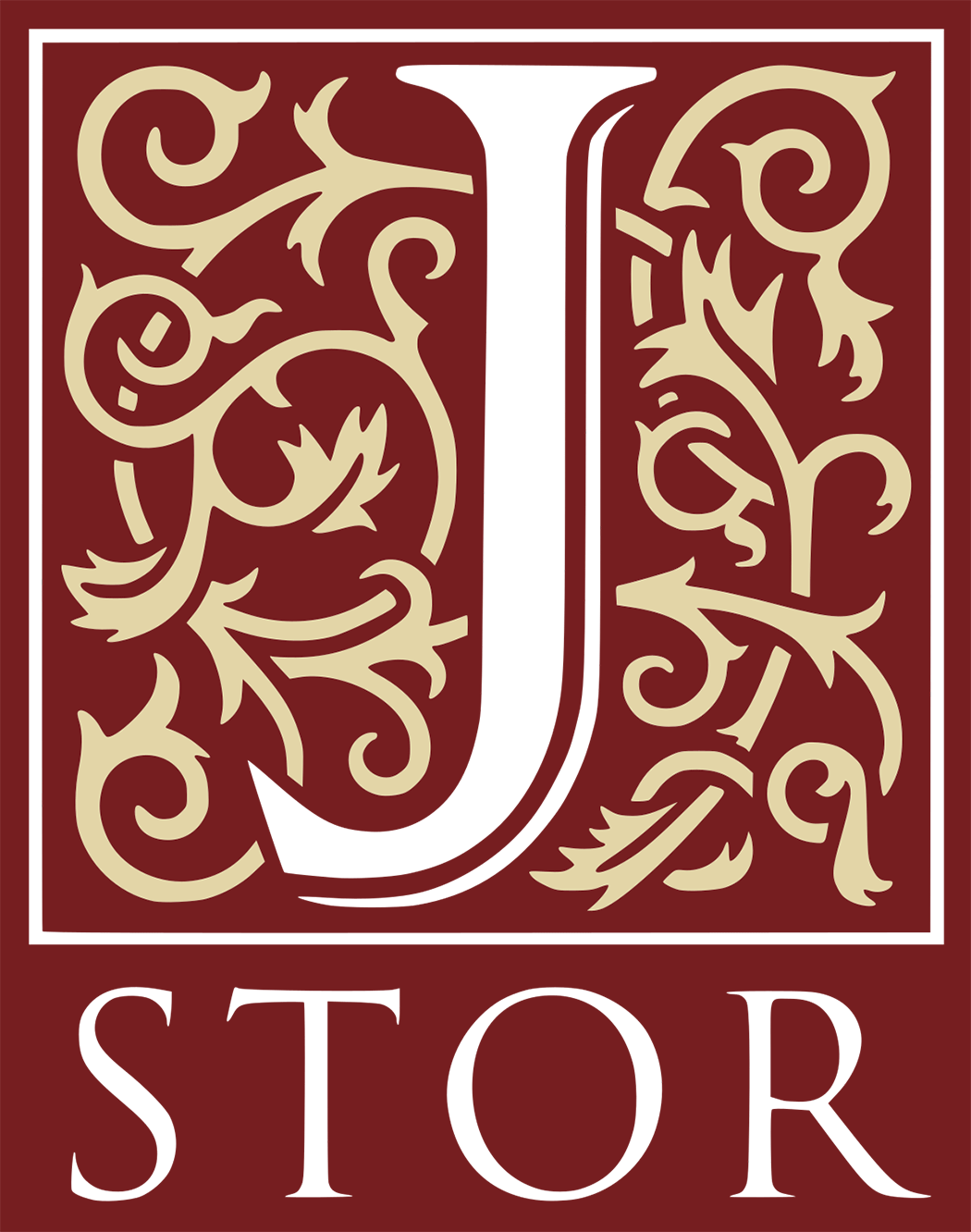


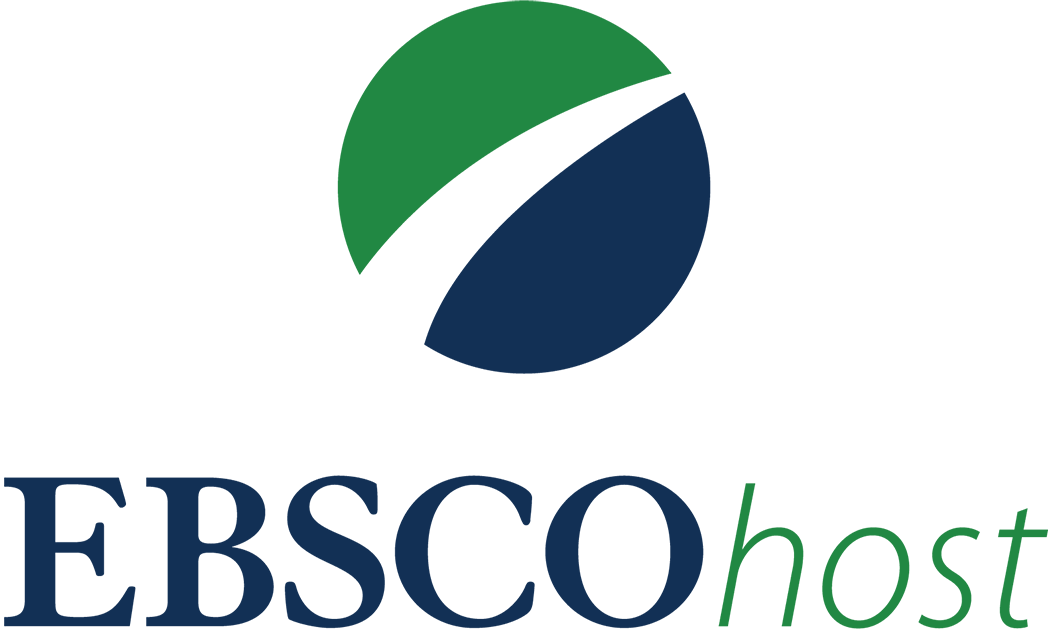

Northeastern Naturalist Vol. 23, No. 1
D.G. Oralls, A.R. Osborn, and J.T. Tessier
2016
151
2016 NORTHEASTERN NATURALIST 23(1):151–162
Potential Influence of Salamanders and Coarse Woody
Debris on the Distribution of Dryopteris intermedia in a
Hardwood Forest
Devin G. Oralls1, Abbey R. Osborn1, and Jack T. Tessier1,*
Abstract - Dryopteris intermedia (Common Wood Fern) is more associated with coarse
woody debris (CWD) than the surrounding forest floor. Along with microsite-based establishment
benefits, dispersal vectors may facilitate this distribution. Some salamanders
are associated with CWD and may serve as dispersal agents for spores of the Common
Wood Fern. In a deciduous forest of south-central New York State, we assessed the ability
of Plethodon cinereus (Red-backed Salamander) and Notophthalmus viridescens (Eastern
Newt) to pick up and transport spores and sori fragments from the dorsal side of the frond,
and rinsed salamanders to collect and germinate any viable spores from their bodies. We
also measured the moisture content of soft CWD, soil beneath hard CWD, and background
soil. Both salamander species picked up reproductive components of Common Wood Fern.
Wild-caught salamanders of both species had viable spores on their bodies. Soft CWD held
more moisture than soil beneath hard CWD and the forest-floor soil. Dispersal by salamanders
and the provision of humid habitat by soft CWD may help to explain the abundance of
Common Wood Fern near CWD.
Introduction
Dryopteris intermedia (Muhl. ex Willd.) A. Gray (Common Wood Fern) is the
most abundant fern in northeastern US forests (Flinn 2006, Tessier and Raynal 2003,
Yorks et al. 2000). This wintergreen fern (Tessier 2008) commonly occurs in microclimates
with abundant moisture such as pits and mounds from tree falls (Flinn 2007,
Maser and Trappe 1984), and has been documented to occur in high abundance near
coarse woody debris (CWD; Flinn 2007, McGee 2001). Its germination has been
experimentally shown to benefit from a high-humidity environment. One study demonstrated
that elevated humidity in experimental plots could lead to a 4000-times
higher chance of gametophyte establishment and a 60-times higher chance of sporophyte
establishment when compared with control plots (Flinn 2007). Therefore,
moisture is critical to the establishment of Common Wood Fern.
Dispersal of spores is also an important factor in fern distribution (Karst et al.
2005). While spores can move throughout a forest, most spores stay near their
source plant (Peck et al. 1990, Penrod and McCormick 1996). For example, most
spores of Dennstaedtia punctilobula (Michx.) Moore (Hayscented Fern) fall
within 4 m of the source plant (Penrod and McCormick 1996) and most spores
of Botrychium virginianum (L.) Swartz (Rattlesnake Fern) fall within 3 m of the
source plant (Peck et al. 1990). Further, spore abundance for the Common Wood
1Division of Liberal Arts and Sciences, SUNY Delhi, 454 Delhi Drive, Delhi, NY 13753.
*Corresponding author - tessiejt@delhi.edu.
Manuscript Editor: Douglas DeBerry
Northeastern Naturalist
152
D.G. Oralls, A.R. Osborn, and J.T. Tessier
2016 Vol. 23, No. 1
Fern is greater in primary forests than in the secondary forests (Flinn 2007) that
dominate the northeast United States (Dyer 2006). These dispersal limitations
suggest that there may be a separate dispersal vector that helps fern spores reach
coarse woody debris.
Like ferns, salamanders are extremely common in northeastern forests.
Plethodon cinereus Green (Red-backed Salamander) is so abundant that its summer
biomass (when fern spores are released) can exceed that of birds during the
breeding season and equal the biomass of mammals in the northeastern United
States (Burton and Likens 1975, Grover 1998, Hairston 1996, Mathewson 2009).
This species is most commonly found beneath cover structures such as logs and
rocks (Bishop 1943, Jaeger 1980, Richmond and Trombulak 2009) but avoids
permanently wet areas (Burger 1935). Red-backed Salamanders roam above leaf
litter during wet periods and can climb more than 2 m up plants in search of food
(Burton and Likens 1975). Notophthalmus viridescens Rafinesque (Eastern Newt)
has a four-stage life cycle. Eastern Newt eggs, larvae, and adults are aquatic,
though juveniles (efts) are terrestrial and, therefore, the focus of this study (Chambers
2008, Healy 1975). Eastern Newt efts occur at an average density of 0.03
per m2 in the northeast United States (Healy 1975), and each individual eft roams
throughout 266.9 m2 on average in a year (Healy 1975). Eastern Newts need to
migrate to ponds for breeding as adults (Hurlbert 1969, Jones and Smyers 2010).
They move the most (mean of 2 m per day) during the early summer (when fern
spores are released) and less (mean of 0.5 to 1.5 m per day) through mid-summer
and fall (Healy 1975). The efts tend to stay on land for 4 to 5 years (Healy 1975)
and prefer moist conditions, with the extent and timing of their activity on top of
the leaf litter being influenced by rain (Healy 1975).
Both macro- and microhabitat features have an important influence on the
abundance of salamanders in the environment. Slopes facing the north tend to be
moister than other slopes because the sun’s rays are not as strong, thereby offering
better habitat for salamanders (Sariyildiz et al. 2005). Habitats for the Red-backed
Salamander and Eastern Newt are near and under coarse woody debris and rocks
(Burger 1935). The Red-backed Salamander is most active near seeps due to the
high moisture (Grover 1998, Grover and Wilbur 2002) and forages best near cover
structures (Grover 1998). High moisture levels provided by CWD help with gas
exchange through the skin in the lungless Red-backed Salamander (Grover 1998).
Common Wood Fern provides shade (George and Bazzaz 1999), which increases
humidity that salamanders prefer (Grover 1998). The spores of the Common Wood
Fern, therefore, may get attached to the salamanders’ porous skin and feet, increasing
dispersal across the forest (as speculated for fern gemmae by Farrar [1985]).
The propensity for these salamander species to live under, on, and around CWD due
to the moisture it provides (Bishop 1943, Burger 1935, Grover 1998, Healy 1975,
Jaeger 1980, Richmond and Trombulak 2009) and the much greater extent of their
annual movement relative to the distance that most fern spores disperse from the
parent plant (Peck et al. 1990, Penrod and McCormick 1996) indicate the potential
for salamanders to be dispersal vectors for fern spores to CWD.
Northeastern Naturalist Vol. 23, No. 1
D.G. Oralls, A.R. Osborn, and J.T. Tessier
2016
153
These facets of fern and salamander ecology led us to 2 questions related to the
abundance of Common Wood Fern near CWD. First, can salamanders function as
a dispersal vector for fern spores? Second, does CWD provide more moisture than
background soil? These questions led to 2, non-mutually exclusive hypotheses.
First, we hypothesized that spores of the Common Wood Fern can get stuck on
salamander bodies through physical contact resulting in viable spores being present
on salamander bodies. Second, we hypothesized that CWD provides a humid
habitat for fern spores thereby promoting germination and survival (Flinn 2007).
We predicted that (1) spores will attach to a salamander if it walks through them,
(2) viable spores can be rinsed from salamander bodies, and (3) soil associated with
CWD will be moister than that in the surrounding area.
Field-Site Description
We conducted the fieldwork in a north-facing deciduous forest in the town of
Delhi, Delaware County, NY (42°14'53"N, 74°56'06"W). The site is dominated
in the overstory by Acer saccharum Marshall (Sugar Maple), A. rubrum L. (Red
Maple), and Fraxinus americana L. (White Ash). The understory is dominated by
Common Wood Fern and D. marginalis (L.) A. Gray (Marginal Wood Fern).
Methods
Prior to the start of field activities, we obtained permission to conduct the work
from SUNY Delhi’s Institutional Animal Care and Use Committee and from the
New York State Department of Environmental Conservation. We chose to work
with Red-backed Salamanders and Eastern Newts because they are the most common
salamanders at our study site.
Spore attachment
First, we assessed if spores can become attached to salamander bodies. This
experiment was necessary to determine the quantity of spores that could become
attached to salamander bodies without background variation from spore availability
in the environment or spore loss from bodies from prior dispersal of spores.
We obtained salamanders for this research component by searching 10-m–wide
belt transects for 100 m, or until 10 individuals of each salamander species had
been found. Transects were used to provide a guided search path such that our
search for salamanders was not biased toward one area or another at the site, and
thus would be much less likely to result in finding salamanders in environmental
conditions (e.g., wet or dry) that made them more or less likely to collect spores
from their surroundings than other salamanders.
We placed each salamander onto the dorsal side of a fertile frond of Common
Wood Fern in a small, cardboard box and coaxed them to walk across a microscope
slide with a thin coat of petroleum jelly (sensu Kimmerer and Young 1995).
Petroleum jelly was used to maximize removal of spores from the salamanders in
order to get as accurate as possible a count of spores collected from the frond. The
Northeastern Naturalist
154
D.G. Oralls, A.R. Osborn, and J.T. Tessier
2016 Vol. 23, No. 1
frond was 10 cm across and the microscope slide was placed directly adjacent to
the frond. The box was the width of the microscope slide. The back end of the box
was left intact to offer a shaded area to which the salamanders would prefer to go.
Once placed on the frond, each salamander completed the movement across the
slide within 10 seconds. We repeated this procedure 10 times with each species of
salamander. We restricted the sample size to 10 in order to limit our impact on the
populations of these vertebrate animals. The same frond was used for each salamander
to minimize the impact of variability in spore abundance among salamander
runs. The vast quantity of spores on a fertile frond (Wagner and Lim Chen 1965,
Whittier and Wagner 1971) relative to the amount that might be removed by any
of the salamanders also ensured an effectively similar degree of spore exposure for
each salamander run.
Salamanders were included as found, resulting in a temporally heterogeneous
mix of the 2 species, also precluding a difference in spore availability between the 2
species. As the sampling took place, 6 of 10 Red-backed Salamanders were among
the first 10 salamanders caught and 6 of 10 Eastern Newts were among the last 10
salamanders caught, demonstrating species evenness in the order of sampling. We
wore lab gloves when handling salamanders to provide them with a smoother surface
compared to human skin, thereby offering greater comfort and less stress to the
animals. We released salamanders adjacent to the cover structure under which they
were found immediately after the procedure. This approach limited their handling
to less than 5 minutes, requiring no extended captivity. As a control, we placed a microscope
slide with petroleum jelly adjacent to the fern frond in the box, but without
allowing a salamander to walk across it. This control provides for a background
count of spores that could have been accidentally moved to the slide via handling
and placement of the slide in the box adjacent to the frond. We examined the microscope
slides with a light microscope at 100x, looking for spores and fragments
of sori with spores of Common Wood Fern. This combination of spores and sori
with spores allowed us to quantify the total number of reproductive pieces moved
by the salamanders. We calibrated our search image by intentionally scraping sori
and spores onto a separate microscope slide and viewing these components prior to
visually searching the experimental slides.
Spore dispersal
To assess if salamanders move fern spores on their bodies, we rinsed salamanders
with rain water and cultured that water on sterile potting soil. We collected a
second set of animals from their habitat for this experiment. Collection, handling,
and release of salamanders were conducted as above such that we collected 10 individuals
of each species. Immediately upon collection, each salamander was held
over a funnel and rinsed with 25 mL of locally collected rainwater. We directed this
water onto pre-moistened, sterile potting soil in a 118-mL, square-shaped, plastic
food-storage container. We collected control samples by rinsing the ends of our
gloved fingers with 25 mL of rain water and collecting that water in soil as above.
This control, therefore, took into account any spores that may have contaminated
Northeastern Naturalist Vol. 23, No. 1
D.G. Oralls, A.R. Osborn, and J.T. Tessier
2016
155
the rainwater during collection or that may have been on our fingers or the funnel
during the field work. These containers were sealed with their cover to retain
moisture and kept in the lab under fluorescent white light until establishment of
gametophytes ceased.
Moisture content
To document the moisture of soil and associated CWD, we established a 10-m–
wide belt transect for 100 m. A transect was used to provide an unbiased sampling
of the CWD and soil, avoiding any tendency to oversample in areas with abundant
CWD due to soil conditions that may make trees prone to falling and thereby confounding
the results. For the purposes of this study, we defined CWD as downed
wood that was at least 10 cm in diameter (McGee 2000). Along this transect, we
searched for both hard and soft CWD. If a gardening trowel could not be easily
inserted into the CWD, we categorized it as hard CWD. If a gardening trowel could
be easily inserted into the CWD, we categorized it as soft CWD. We collected soil
from beneath the hard CWD and from soil under leaf litter that was both 1 m from
this CWD along the transect in reverse of our direction of sampling and 1 m away
from any other CWD for comparison. From the soft CWD, we collected a sample
of the CWD itself along with a sample of soil from under leaf litter 1 m along the
transect in reverse of our direction of sampling. The soft CWD itself was sampled
because this is the medium in which Common Wood Fern has been shown to be
abundant (Flinn 2007, McGee 2001) and it provides a contrast with the soil available
in other locations in the field (i.e., forest soil and soil beneath hard CWD).
Forest floor soil was collected from near hard CWD and soft CWD to control for
variation in soil conditions near the 2 types of CWD (e.g., moist soil and not just
decay duration could help to make the soft CWD softer than the hard CWD). We
sampled 30 replicates of each soil type. The soil samples were brought to the lab
at SUNY Delhi, weighed immediately, and allowed to dry to a constant mass. We
then calculated their soil moisture content (Brower et al. 1990 ).
Statistical analysis
We compared the number of spores and sori components with spores of Common
Wood Fern found on the microscope slides and the number of gametophytes
established from rinse water among the control and 2 salamander species using
2 separate Kruskal-Wallis tests due to the small sample size. Following the significant
Kruskal-Wallis result when comparing reproductive components moved
to the microscope slides, we made post-hoc comparisons among the species and
control using Mann-Whitney tests with an adjusted α = 0.01667 to control for the
experiment-wise error rate. We compared the moisture of substrate material gathered
from the 4 location types (soil beneath hard CWD, soil nea r hard CWD, soft
CWD, and soil near soft CWD) using analysis of variance followed by a Tukey’s
family error rate means comparison. All statistical analyses were conducted using
Minitab version 16 (Minitab, Inc., State College, PA) at α = 0.05, unless otherwise
stated.
Northeastern Naturalist
156
D.G. Oralls, A.R. Osborn, and J.T. Tessier
2016 Vol. 23, No. 1
Results
While Red-backed Salamanders moved twice as much reproductive components
of Common Wood Fern than Eastern Newts, the difference was not statistically
significant ( W = 134.0, P = 0.0290; Fig. 1). More reproductive components were
found on slides from the 2 salamander species treatments than on the control slides
(H2 = 15.21, P < 0.0001; Control vs. v: W = 61.0, P = 0.0005; Control vs. Eastern
Newt: W = 74.0, P = 0.0114; Fig. 1). There was no pattern between spore count and
order of the sampling in the field, indicating that there was not a decline in spore
availability from the frond as the experiment was conducted.
Three out of 10 samples of rinse water from each of the 2 salamander species
grew fern gametophytes, while none of the control samples did. The lack of
gametophytes from the control samples indicates that there was no spore contamination
of the gloves used in the experiment. The number of gametophytes grown per
sample was not significantly different between species nor from the control (H2 =
3.68, P = 0.159; Fig. 2).
The moistest soil was that of the soft CWD (F3,116 = 29.17, P < 0.0001; Fig. 3),
while that beneath hard CWD and both adjacent soils did not differ significantly
(Fig. 3). The soft CWD held more than twice as much water (405.9 g/100 g dry soil)
as the other soil types (158.6 g/100 g dry soil) (Fig. 3).
Figure 1. Number
of spores or sori
components with
spores found on microscope
slides that
had been walked
across by each salamander
species after
being placed on
the dorsal side of
a fertile frond of
Dryopteris intermedia
(Common Wood
Fern) in the Town
of Delhi, NY. Center
line represents
the 50th percentile,
dashed line represents
the mean, box
represents the 25th
and 75th percentiles,
and whiskers represent the 10th and 90th percentiles. Treatments with different letters are
significantly different at α = 0.05 based on a Kruskall-Wallis test and subsequent Mann-
Whitney post-hoc tests.
Northeastern Naturalist Vol. 23, No. 1
D.G. Oralls, A.R. Osborn, and J.T. Tessier
2016
157
Discussion
Red-backed Salamanders and Eastern Newts are possible dispersal agents of
fern spores (Figs. 1, 2). To our knowledge, this is the first study to document uptake
and movement of spores by salamanders. Our first experiment demonstrated
Figure 2. Number of
gametophytes grown
from water used to
rinse salamanders
from the Town of
Delhi, NY. Center
line represents
the 50th percentile,
dashed line represents
the mean, box
represents the 25th
and 75th percentiles,
and whiskers represent
the 10th and 90th
percentiles. Means
were not significantly
different based on
a Kruskall-Wallis
test at ɑ = 0.05.
Figure 3. Moisture
of 4 types of soil
in a second-growth
hardwood forest in
the Town of Delhi,
NY, in the summer
of 2014. Center line
represents the 50th
percentile, dashed
line represents the
mean, box represents
the 25th and
75th percentiles, and
whiskers represent
the 10th and 90th percentiles.
Soil types
with different letters
are significantly different
at α = 0.05
based on an analysis
of variance and
Tukey’s family error
rate tests.
Northeastern Naturalist
158
D.G. Oralls, A.R. Osborn, and J.T. Tessier
2016 Vol. 23, No. 1
that both salamander species collect spores from the environment (Fig. 1). Our
second experiment showed that viable spores remain on the salamanders as they
move from the spore source (Fig. 2). The lower count of viable spores on the freeroaming
salamanders compared to the spore count on animals that had just picked
up spores from a frond suggests that spores are lost from the salamanders as they
move in the environment, indicating dispersal of the spores. Salamanders may
move more spores on their bodies than our data suggest because our rinsing experiment
took place in early August due to logistical delays, whereas spore release in
Common Wood Fern peaks in late June (Flinn 2007) coincident with the time of
greatest movement for Eastern Newts (Healy 1975). Salamanders could encounter
fern spores under non-experimental conditions as they climb vegetation (Burton
and Likens 1975, Trauth et al. 2000) and move in the understory among plants, leaf
litter, and soil (Peck et al. 1990, Penrod and McCormick 1996). Flinn (2007) clearly
demonstrated that high humidity benefits fern germination and survivorship. The
moisture in the soft CWD, therefore, provides important habitat for young ferns
(Fig. 3), and the salamander species we studied are commonly found in these areas
(Burger 1935, Grover 1998). In our study area, we found 10 individuals of each
species within 100 m of a 10-m belt transect each time we searched for them in and
around CWD. Collectively, these results show that dispersal by salamanders and the
moisture in old CWD may both help to explain the prevalence of Common Wood
Fern near CWD in forests (Flinn 2007, McGee 2001).
Dispersal of fern spores by salamanders could significantly chan ge the distance
and location of dispersal. First, these salamanders move greater distances than most
fern spores that don’t have the aid of active transport. Most fern spores fall within
4 m of the adult plant (Peck et al. 1990, Penrod and McCormick 1996). Eastern
Newts can move 2 m per day during the time when Common Wood Fern spores are
released (Healy 1975) and male Red-backed Salamanders can move as far as 10 m
or more per day during the growing season (Liebgold et al. 2011). Second, these
salamanders are associated with the high-moisture environment of CWD (Fig. 3;
e.g., Healy 1975, Richmond and Trombulack 2009) that dramatically raises the
germination and establishment success of the Common Wood Fern (Flinn 2007).
Therefore, these salamanders are likely to increase the dispersal distance of spores
of the Common Wood Fern and to move them to a suitable location for germination
and survival.
Other animals are known to disperse spores. Ants are known to disperse moss
gemmae (Rudolphi 2009), and slugs can disperse moss spores (Kimmerer and
Young 1995). Fungal spores can be dispersed by worms and birds (McIlveen
and Cole 1976) and in the feces of small mammals (Zaharick et al. 2015). Following
dispersal of propagules, microsite can play an important role in the establishment
of plants (Beatty 1984, Flinn 2007, Peterson and Campbell 1993). The increase in
moisture content through decay class in CWD (Maser and Trappe 1984) provides
the cover and moisture preferred by Red-backed Salamanders (Grover 1998), further
encouraging dispersal of fern spores to CWD and establishment of ferns (Flinn
2007, McGee 2001, Peck et al. 1990).
Northeastern Naturalist Vol. 23, No. 1
D.G. Oralls, A.R. Osborn, and J.T. Tessier
2016
159
This evidence of spore dispersal by salamanders suggests that amphibian decline
may have a significant impact on movement of fern spores. While these salamander
species are very common and abundant in northeastern forests (Burton and Likens
1975, Healy 1975), amphibians are experiencing a global decline in abundance
(Hof et al. 2011), and body size in the Red-backed Salamander is decreasing in the
southern part of its range due to climate change (Caruso et al. 2014). Depending
on future changes in climate (Peterson et al. 2013) and land use (Thompson et al.
2013), the ability of fern spores to get to the microhabitat that benefits them most
(Flinn 2007) may be hampered if salamander abundance decreases.
Other questions relating to salamanders’ role in spore disperal deserve study.
Given that the gut contents of Red-backed Salamanders include ants and beetles
(Hamilton 1932), spores attached to these insects may show up in the feces of
salamanders and serve as an additional dispersal mechanism mediated by salamanders.
Vegetation has been found in the stomachs of both of these salamander
species (Cochran 1911, Ries and Bellis 1966), further suggesting that feces could
be an additional dispersal mechanism in need of quantification. Spores of other
species that salamanders can move need to be identified. The burrowing capacity of
salamanders (Heatwole 1960) raises the possibility that they may play a role in the
development of deep spore banks (Dyer and Lindsay 1992). An important next step
is to document how readily spores move from the bodies of the salamanders to soil
and CWD. Given this potential for salamanders to be important to fern dispersal,
current and potential reductions in amphibian abundance may impact fern distribution
and should be studied (Caruso et al. 2014, Martel et al. 2 014).
In conclusion, the movement of fern spores by salamanders, the presence of fern
spores on salamander bodies, and the high moisture content of soft CWD offer a
mechanism for the observed abundance of Common Wood Fern adjacent to CWD.
Further study should address alternative dispersal mechanisms by salamanders, additional
species that salamanders can disperse, and the impact of amphibian decline
on plant dispersal.
Acknowledgments
The authors thank an anonymous donor for funding, Karen Teitelbaum for assistance
with obtaining permission for the study from the New York State Department of Environmental
Conservation, SUNY Delhi for the use of the study site, and anonymous reviewers
for constructive comments on the manuscript.
Literature Cited
Beatty, S.W. 1984. Influence of microtopography and canopy species on spatial patterns of
forest understory plants. Ecology 65:1406–1419.
Bishop, S.C. 1943. Handbook of Salamanders: The Salamanders of the United States, of
Canada, and of Lower California. Volume 3. Cornell University Press, Ithaca, NY. 557
pp.
Brower, J.E., J.H. Zar, and C.N. von Ende. 1990. Field and Laboratory Methods for General
Ecology. Wm. C. Brown Publishers, Dubuque, IA. 237 pp.
Northeastern Naturalist
160
D.G. Oralls, A.R. Osborn, and J.T. Tessier
2016 Vol. 23, No. 1
Burger, J.W. 1935. Plethodon cinereus (Green) in eastern Pennsylvania and New Jersey.
American Naturalist 69:578–586.
Burton, T.M., and G.E. Likens. 1975. Salamander populations and biomass in the Hubbard
Brook Experimental Forest, New Hampshire. Copeia 1975:541–546.
Caruso, N.M., M.W. Sears, D.C. Adams, and K.R. Lips. 2014. Widespread rapid reductions
in body size of adult salamanders in response to climate change. Global Change Biology
20:1751–1759.
Chambers, D.L. 2008. Logging road effects on breeding-site selection in Notophthalmus
viridescens (Red-spotted Newt) and three ambystomatid salamanders in south-central
Pennsylvania. Northeastern Naturalist 15:123–130.
Cochran, M.E. 1911. The biology of the Red-backed Salamander (Plethodon cinereus erythronotus
Green). Biological Bulletin 20:332–349.
Dyer, A.F., and S. Lindsay. 1992. Soil spore banks of temperate ferns. American Fern Journal
82:89–122.
Dyer, J.M. 2006. Revisiting the deciduous forests of eastern North America. BioScience
56:341–352.
Farrar, D.R. 1985. Independent fern gametophytes in the wild. Proceedings of the Royal
Society of Edinburgh 86B:361–369.
Flinn, K.M. 2006. Reproductive biology of three fern species may contribute to differential
colonization success in post-agricultural forests. American Journal of Botany
93:1289–1294.
Flinn, K.M. 2007. Microsite-limited recruitment controls fern colonization of post-agricultural
forests. Ecology 88:3103–3114.
George, L.O., and F.A. Bazzaz. 1999. The fern understory as an ecological filter: Growth
and survival of canopy-tree seedlings. Ecology 80:846–856.
Grover, M.C. 1998. Influence of cover and moisture on abundances of the terrestrial
salamanders Plethodon cinereus and Plethodon glutinosus. Journal of Herpetology
32:489–497.
Grover, M.C., and H.M. Wilbur. 2002. Ecology of ecotones: Interactions between salamanders
on a complex environmental gradient. Ecology 83:21 12–2123.
Hairston, N.G., Sr. 1996. Predation and competition in salamander communities. Pp.161–
190, In M.L. Cody and J.A. Smallwood (Eds.). Long-term studies of vertebrate communities.
Academic Press, New York, NY. 597 pp.
Hamilton , W.J., Jr. 1932. The food and feeding habits of some eastern salamanders. Copeia
1932:83–86.
Healy, W.R. 1975. Terrestrial activity and home range in efts of Notophthalmus viridescens.
American Midland Naturalist 93:131–138.
Heatwole, H. 1960. Burrowing ability and behavioral responses to desiccation of the salamander
Plethodon cinereus. Ecology 41:661–668.
Hof, C., M.B. Araújo, W. Jetz, and C. Rahbek. 2011. Additive threats from pathogens, climate
and land-use change for global amphibian diversity. Nature 480:516–519.
Hurlbert, S.H. 1969. The breeding migrations and interhabitat wandering of the Vermilion-
spotted Newt, Notophthalmus viridescens (Rafinesque). Ecological Monographs
39:465–488.
Jaeger, R.G. 1980. Microhabitats of a terrestrial forest salamander . Copeia 1980:265–268.
Jones, M.T., and S.D. Smyers. 2010. Occurrence of pond-breeding amphibians at alpine
ponds in the White Mountains, New Hampshire. Northeastern Naturalist 17:161– 166.
Karst, J., B. Gilbert, and M.J. Lechowicz. 2005. Fern community assembly: The roles of
chance and the environment at local and intermediate scales. Ec ology 86:2473–2486.
Northeastern Naturalist Vol. 23, No. 1
D.G. Oralls, A.R. Osborn, and J.T. Tessier
2016
161
Kimmerer, R.W., and C.C. Young. 1995. The role of slugs on dispersal of the asexual propagules
of Dicranum flagellare. The Bryologist 98:149–153.
Liebgold, E.B., E.D. Brodie III, and P.R. Cabe. 2011. Female philopatry and male-based
dispersal in a direct-developing salamander, Plethodon cinereus. Molecular Ecology
20:249–257.
Martel, A., M. Blooi, C. Adriaensen, P. Van Rooij, W. Beukema, M.C. Fisher, R.A. Farrer,
B.R. Schmidt, U. Tobler, K. Goka, K.R. Lips, C. Muletz, K.R. Zamudio, J. Bosch,
S. Lötters, E. Wombwell, T.W.J. Garner, A.A. Cunningham, A. Spitzen-van der Sluijs,
S. Salvidio, R. Ducatelle, K. Nishikawa, T.T. Nguyen, J.E. Kolby, I. Van Bocxlaer, F.
Bossuyt, and F. Pasmans. 2014. Recent introduction of a chytrid fungus endangers western
palearctic salamanders. Science 346:630–631.
Maser, C., and J.M. Trappe. 1984. Seen and unseen world of the fallen tree. General Technical
Report PNW-164. USDA Forest Service, Pacific Northwest Forest and Range
Experiment Station, Portland, OR. 56 pp.
Mathewson, B. 2009. The relative abundance of Eastern Red-backed Salamanders in Eastern
Hemlock-dominated and mixed deciduous forests at Harvard Forest. Northeastern
Naturalist 16:1–12.
McGee, G.G. 2000. The contribution of beech bark disease-induced mortality to coarse
woody debris in northern hardwood stands of Adirondack Park, New York, USA. Canadian
Journal of Forest Research 30:1453–1462.
McGee, G.G. 2001. Stand-level effects on the role of decaying logs as vascular plant habitat
in Adirondack northern hardwood forests. Journal of the Torrey Botanical Society
128:370–380.
McIlveen, W.D., and H. Cole Jr. 1976. Spore dispersal of endogonaceae by worms, ants,
wasps, and birds. Canadian Journal of Botany 54:1486–1489.
Peck, J.H., C.J. Peck, and D.R. Farrar. 1990. Influences of life-history attributes on formation
of local and distant fern populations. American Fern Journal 80:126–142.
Penrod, K.A., and L.H. McCormick. 1996. Abundance of viable hay-scented fern spores
germinated from hardwood forest soils at various distances from a source. American
Fern Journal 86:69–79.
Peterson, C.J., and J.E. Campbell. 1993. Microsite differences and temporal change in plant
communities of treefall pits and mounds in an old-growth forest. Bulletin of the Torrey
Botanical Club 120:451–460.
Peterson, T.C., R.R. Heim Jr., R. Hirsch, D.P. Kaiser, H. Brooks, N.S. Diffenbaugh, R.M.
Dole, J.P. Giovannettone, K. Guirguis, T.R. Karl, R.W. Katz, K. Kunkel, D. Lettenmaier,
G.J. McCabe, C.J. Paciorek, K.R. Ryberg, S. Schubert, V.B.S. Silva, B.C. Stewart, A.V.
Vecchia, G. Villarini, R.S. Vose, J. Walsh, M. Wehner, D. Wolock, K. Wolter, C.A.
Woodhouse, and D. Wuebbles. 2013. Monitoring and understanding changes in heat
waves, cold waves, floods, and droughts in the United States. Bulleting of the American
Meteorological Society 94:821–834.
Richmond, L.S., and S.C. Trombulak. 2009. Distribution of Red-backed Salamander
(Plethodon cinereus) with respect to cover-object characteristics in the Green Mountains
of Vermont. Northeastern Naturalist 16:13–26.
Ries, K.M., and E.D. Bellis. 1966. Spring food habits of the Red-spotted Newt in Pennsylvania.
Herpetologica 22:152–155.
Rudolphi, J. 2009. Ant-mediated dispersal of asexual moss propagules. The Bryologist
112:73–79.
Sariyildiz, T., J.M. Anderson, and M. Kucuk. 2005. Effects of tree species and topography
on soil chemistry, litter quality, and decomposition in northeast Turkey. Soil Biology
and Biochemistry 37:1695–1706.
Northeastern Naturalist
162
D.G. Oralls, A.R. Osborn, and J.T. Tessier
2016 Vol. 23, No. 1
Tessier, J.T. 2008. Leaf habit, phenology, and longevity of eleven forest understory plant
species in Algonquin State Forest, northwest Connecticut, USA. Botany 86:4 57–465.
Tessier, J.T., and D.J. Raynal. 2003. Vernal nitrogen and phosphorus retention by forest
understory vegetation and soil microbes. Plant and Soil 256:443 –453.
Thompson, J.R., D.N. Carpenter, C.V. Cogbill, and D.R Foster. 2013. Four centuries of
change in northeastern United States forests. PLoS ONE 8:e72540. Doi:10.1371/journal.
pone.0072540.
Trauth, S.E., M.L. McCallum, B.J. Ball, and V.E. Hoffman. 2000. Plethodon caddoensis
(Caddo Mountain Salamander) and Plethodon serratus (Southern Redback Salamander).
Nocturnal climbing activity. Herpetological Review 31:232–233.
Wagner, W.H., Jr., and K. Lim Chen. 1965. Abortion of spores and sporangia as a tool in the
detection of Dryopteris hybrids. American Fern Journal 55:9–29.
Whittier, P., and W.H. Wagner Jr. 1971. The variation in spore size and germination in
Dryopteris taxa. American Fern Journal 61:123–127.
Yorks, T.E., D.J. Leopold, and D.J. Raynal. 2000. Vascular plant propagule banks of six
Eastern Hemlock stands in the Catskill Mountains of New York. Journal of the Torrey
Botanical Society 127:87–93.
Zaharick, J., H. Beck, and V. Beauchamp. 2015. An experimental test of epi- and endozoochory
of arbuscular mychorrhizal fungi spores by small mammals in a Maryland
forest. Northeastern Naturalist 22:163–177.