Scale-dependent Effects of Coyote-predation Risk on
Patterns of White-tailed Deer Browsing Along Linear Forest
Edges
Joshua L.B. Pierce, Sabrina A. Dalinsky, Andre-Anne Chenaille, Lewis M. Lolya, Jennifer L. Maguder, Chloe Mattilio, Grace V. Mayhew, Erin Regan, and David A. Patrick
Northeastern Naturalist, Volume 22, Issue 2 (2015): 262–272
Full-text pdf (Accessible only to subscribers. To subscribe click here.)
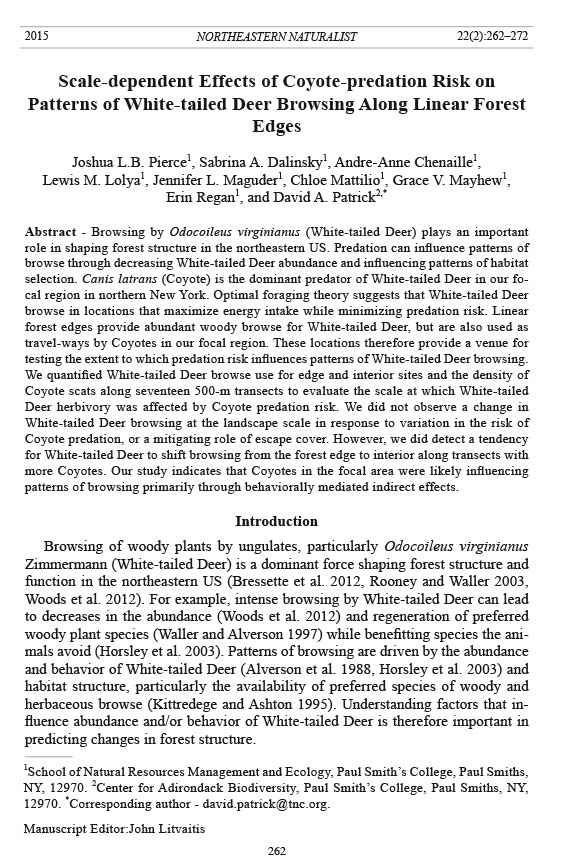
Access Journal Content
Open access browsing of table of contents and abstract pages. Full text pdfs available for download for subscribers.
Current Issue: Vol. 30 (3)
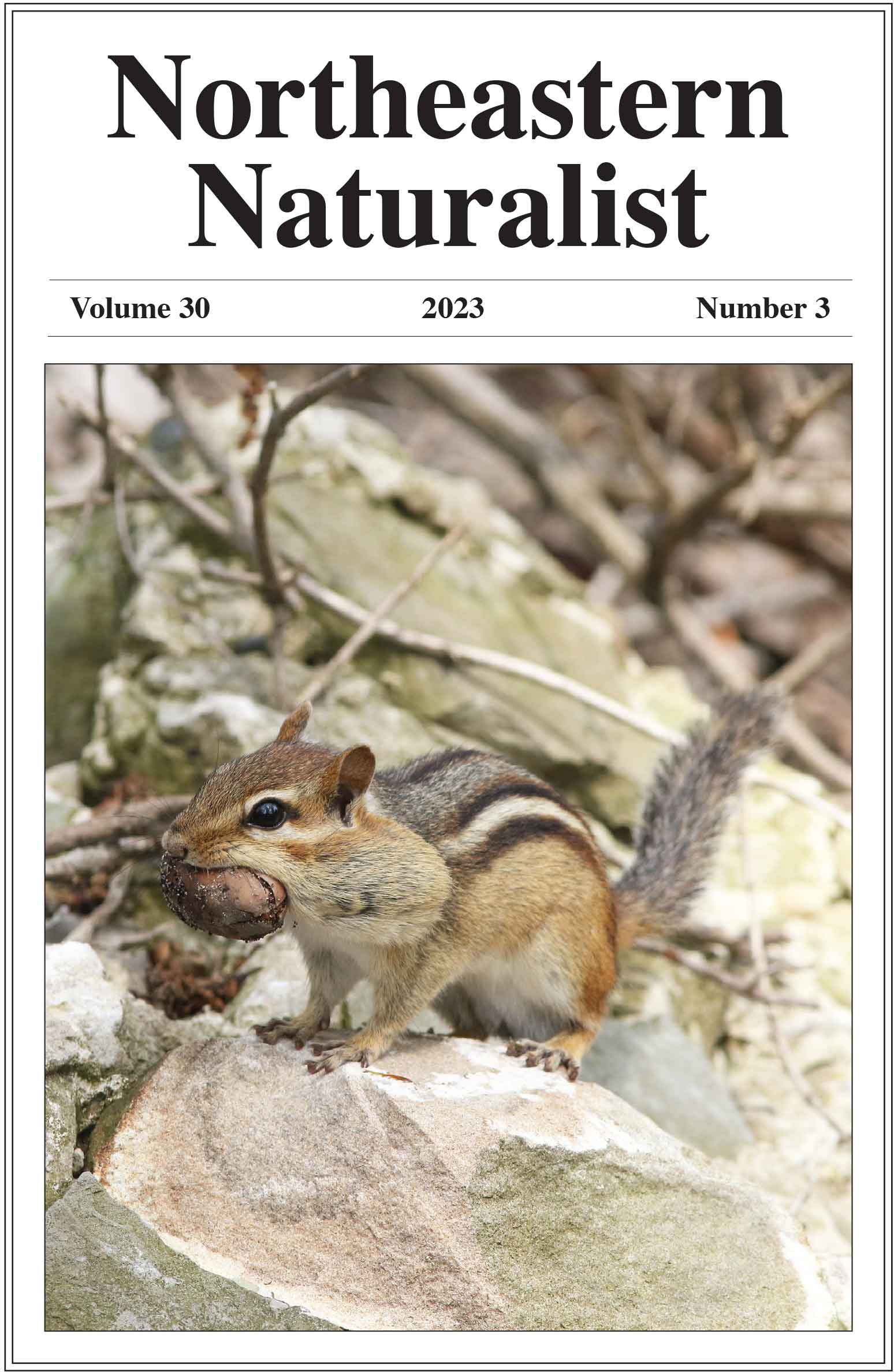
Check out NENA's latest Monograph:
Monograph 22
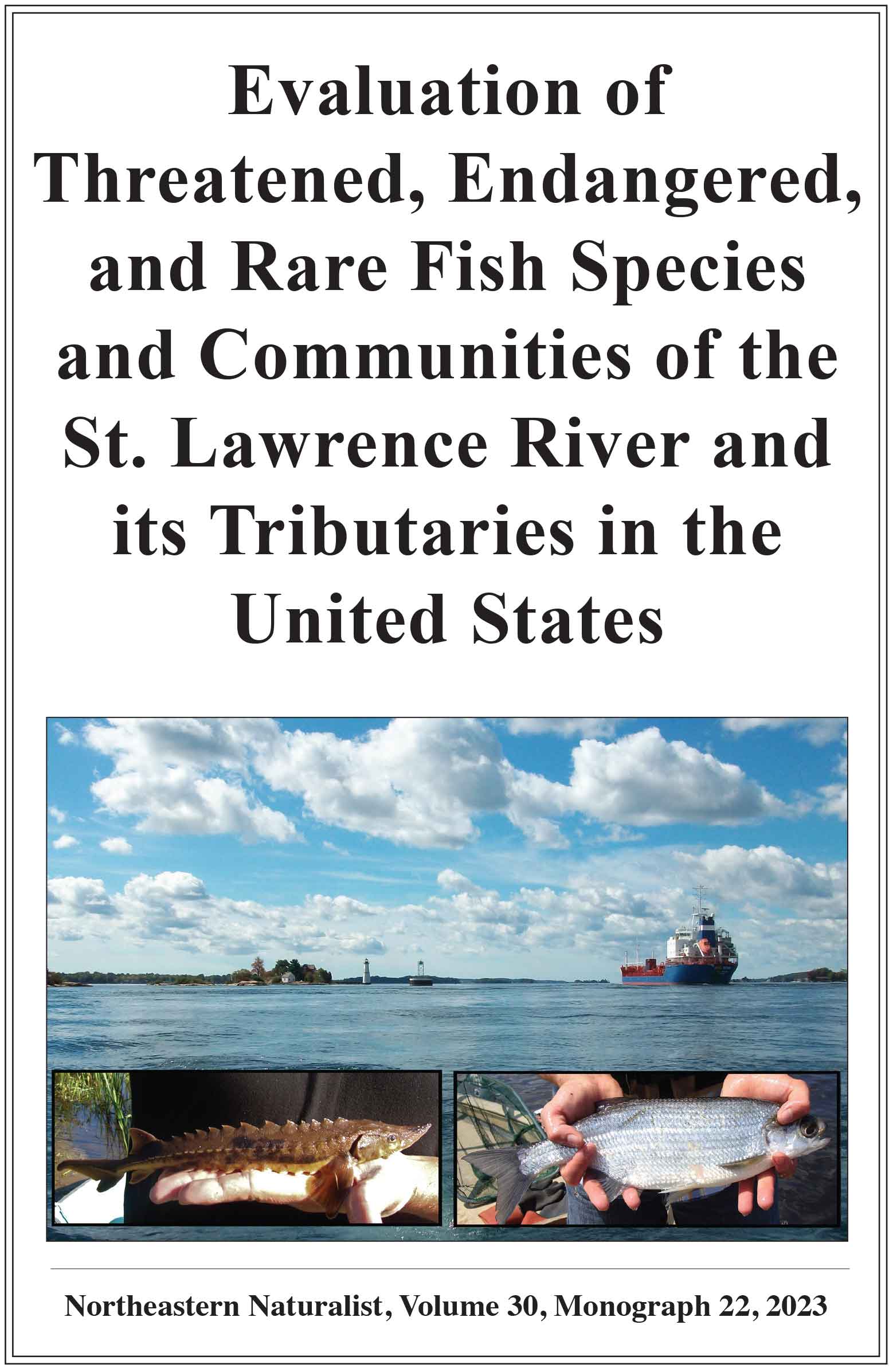
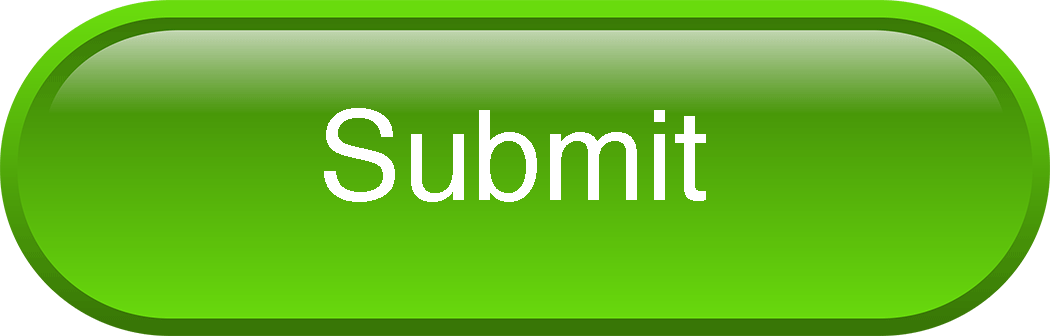
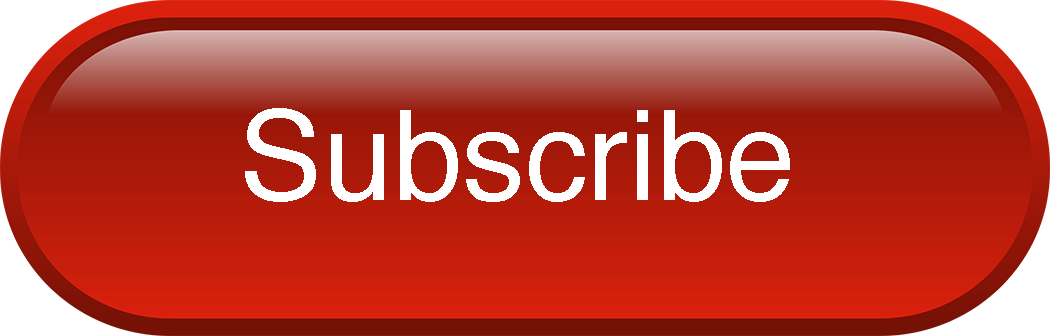
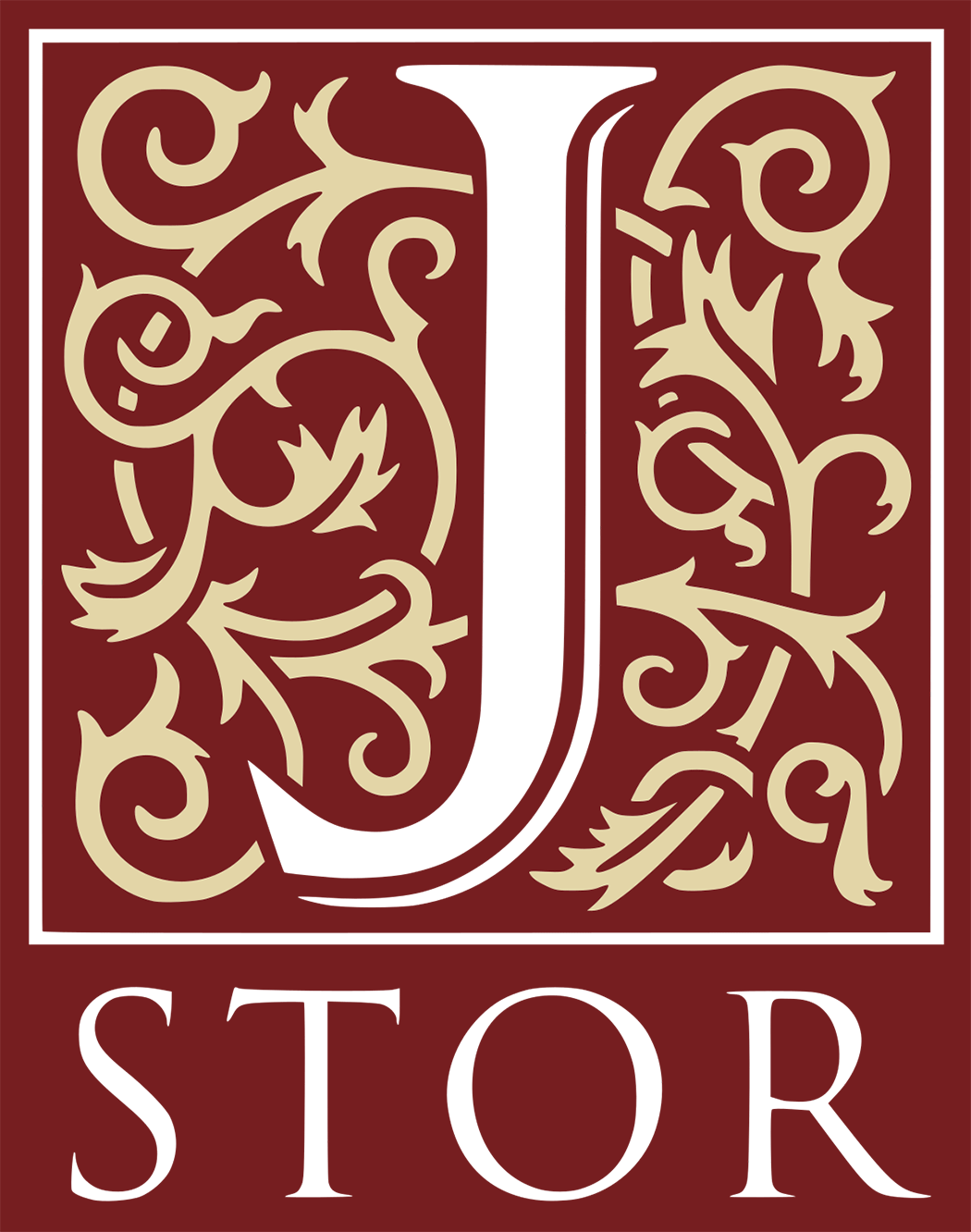


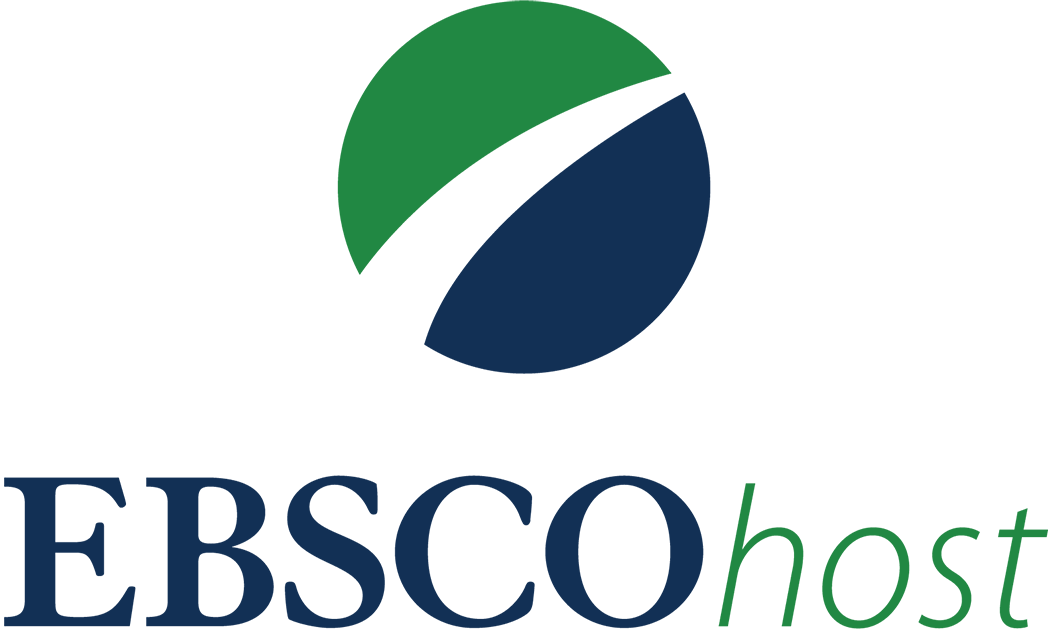

Northeastern Naturalist
262
J.L.B. Pierce, et al.
22001155 NORTHEASTERN NATURALIST 2V2(o2l). :2226,2 N–2o7. 22
Scale-dependent Effects of Coyote-predation Risk on
Patterns of White-tailed Deer Browsing Along Linear Forest
Edges
Joshua L.B. Pierce1, Sabrina A. Dalinsky1, Andre-Anne Chenaille1,
Lewis M. Lolya1, Jennifer L. Maguder1, Chloe Mattilio1, Grace V. Mayhew1,
Erin Regan1, and David A. Patrick2,*
Abstract - Browsing by Odocoileus virginianus (White-tailed Deer) plays an important
role in shaping forest structure in the northeastern US. Predation can influence patterns of
browse through decreasing White-tailed Deer abundance and influencing patterns of habitat
selection. Canis latrans (Coyote) is the dominant predator of White-tailed Deer in our focal
region in northern New York. Optimal foraging theory suggests that White-tailed Deer
browse in locations that maximize energy intake while minimizing predation risk. Linear
forest edges provide abundant woody browse for White-tailed Deer, but are also used as
travel-ways by Coyotes in our focal region. These locations therefore provide a venue for
testing the extent to which predation risk influences patterns of White-tailed Deer browsing.
We quantified White-tailed Deer browse use for edge and interior sites and the density of
Coyote scats along seventeen 500-m transects to evaluate the scale at which White-tailed
Deer herbivory was affected by Coyote predation risk. We did not observe a change in
White-tailed Deer browsing at the landscape scale in response to variation in the risk of
Coyote predation, or a mitigating role of escape cover. However, we did detect a tendency
for White-tailed Deer to shift browsing from the forest edge to interior along transects with
more Coyotes. Our study indicates that Coyotes in the focal area were likely influencing
patterns of browsing primarily through behaviorally mediated indirect effects.
Introduction
Browsing of woody plants by ungulates, particularly Odocoileus virginianus
Zimmermann (White-tailed Deer) is a dominant force shaping forest structure and
function in the northeastern US (Bressette et al. 2012, Rooney and Waller 2003,
Woods et al. 2012). For example, intense browsing by White-tailed Deer can lead
to decreases in the abundance (Woods et al. 2012) and regeneration of preferred
woody plant species (Waller and Alverson 1997) while benefitting species the animals
avoid (Horsley et al. 2003). Patterns of browsing are driven by the abundance
and behavior of White-tailed Deer (Alverson et al. 1988, Horsley et al. 2003) and
habitat structure, particularly the availability of preferred species of woody and
herbaceous browse (Kittredege and Ashton 1995). Understanding factors that influence
abundance and/or behavior of White-tailed Deer is therefore important in
predicting changes in forest structure.
1School of Natural Resources Management and Ecology, Paul Smith’s College, Paul Smiths,
NY, 12970. 2Center for Adirondack Biodiversity, Paul Smith’s College, Paul Smiths, NY,
12970. *Corresponding author - david.patrick@tnc.org.
Manuscript Editor:John Litvaitis
Northeastern Naturalist Vol. 22, No. 2
J.L.B. Pierce, et al.
2015
263
Predation is one of the principal forces driving patterns of ungulate browsing
(Beschta and Ripple 2009). For example, extirpation of top predators such as Canis
lupus L. (Gray Wolf), has been linked to over-abundance of and over-browsing by
ungulate species including White-tailed Deer, Cervus elaphus canadensis Erxleben
(Elk), and Alces alces L. (Moose) (Beschta and Ripple 2009). In addition to limiting
ungulate abundance, top predators also may influence patterns of behavior including
where and when ungulates choose to browse (Halofsky and Ripple 2008, Ripple
and Beschta 2004). The interrelationship between predators and prey and the subsequent
effects on prey-habitat selection and patterns of feeding are well described
by the concept of optimal foraging theory (MacArthur and Pianka 1966, Pyke 1984,
Pyke et al. 1977). This general theory has more recently been adapted to specifically
focus on predator–prey relationships between top carnivores and ungulate species,
particularly through the use of resource-selection functions (Hebblewhite et al.
2005). Application of this theory demonstrates that predation can influence patterns
of ungulate browsing at multiple spatial scales depending on the effects of the
predator on prey abundance and/or prey behavior, and the structure of the environment
(Altendorf et al. 2001, Frair et al. 2005, Halofsky and Ripple 2008, Jones and
Hudson 2002).
Canis latrans Say (Coyote) is the dominant predator of White-tailed Deer
throughout much of the northeastern US and southeastern Canada (Messier et al.
1986). This species is a relatively recent addition to the region, having expanded
its range likely in response to the extirpation of the Canis lupus lycaon Schreber
(Eastern Timber Wolf). By the late 1950s, Coyotes had arrived in our focal region
in the Northern Adirondacks, NY, in appreciable numbers (Fener et al. 2005,
Gompper 2002b). Although the ecology of Coyotes in the Northeast seems to be
variable, there is strong evidence in our focal region indicating that Coyotes have
adopted a functional role similar to the extirpated Eastern Timber Wolf, including
group hunting and depredating adult White-tailed Deer, particularly during winter
months (Gompper 2002a, Patterson and Messier 2001, Warsen 2012). Coyotes can
potentially influence patterns of White-tailed Deer browsing at a number of scales
including reducing their regional and local densities through predation, and influencing
patterns of habitat use.
Shifts in behavior are likely to occur in areas where there is an abundance of
high-quality woody browse coupled with frequent occurrence of Coyotes. Linear
forest edges represent areas where these conditions are present. Edges typically
provide readily accessible woody browse and hence are heavily used by ungulates
(Alverson et al. 1988, Jones and Hudson 2002, Williamson and Hirth 1985). However,
linear edges are also easily accessible to and offer abundant food for canid
predators (Heske et al. 1999). In our study region, White-tailed Deer spend the
winter months in forested deer-yards, but use edges during the remainder of the
year. While most Coyote depredation of adult White-tailed Deer is likely to occur
in winter and hence not when deer are on edges, we observed White-tailed Deer
bones in fresh Coyote scat in the fall; thus, it is likely that White-tailed Deer are at
risk of predation by Coyotes throughout the year. The extent to which predator and
Northeastern Naturalist
264
J.L.B. Pierce, et al.
2015 Vol. 22, No. 2
prey use of edges (and hence encounter frequency) translates into predation risk
(either actual or perceived) may also be driven by fine-scale variation in habitat
because habitat characteristics can influence the probability that an encounter will
result in a successful kill (Hebblewhite et al. 2005). For example, denser cover may
serve as either escape cover for ungulates such as White-tailed Deer or concealment
cover for predators (Creel et al. 2005, Husseman et al. 2003). Forest roads created
during timber harvests (and analogous disused railway lines) represent one of the
most prevalent types of linear edges in the Adirondack Park. High-use roadways
are often avoided by wild canids (Mladenhoff et al. 1995, Roy and Dorrance 1985),
but low-use travel ways may be preferred corridors for movement (Whittington et
al. 2005, but see Kolbe et al. 2010). The use of low-intensity roads and rail lines
as travel ways is supported by observational evidence (tracks and scat) indicating
linear movement of Coyotes along these pathways in the focal region throughout
the year (D.A. Patrick, unpubl. data).
The goal of our study was to evaluate the scale at which White-tailed Deer
herbivory was affected by how frequently Coyotes used linear forest edges, and to
use this information to understand the likely effects of predators in driving forest
structure through influencing patterns of browsing on woody plants. To address
this goal, we tested 3 hypotheses: (1) higher use of edges by Coyotes reduces the
amount of browsing of woody plants at the landscape scale by reducing the abundance
of White-tailed Deer via either predation or stimulating deer to locate home
ranges in different areas, (2) higher use of edges by Coyotes causes White-tailed
Deer to shift foraging into the forest interior but not to relocate home ranges, and
(3) fine-scale habitat heterogeneity (escape cover) along forest edges mitigates
some of the effects of Coyote use on White-tailed Deer browsing.
Methods
Study area
We conducted our study from 2 October to 8 November 2013 in second-growth
forest in the vicinity of Paul Smith’s College in the northern Adirondack Park, NY
(44°26'N, 74°15'W). Focal stands consisted of mixed deciduous-coniferous forest
with dominant overstory species including Acer saccharum Marsh. (Sugar Maple),
A. rubrum L. (Red Maple), Betula alleghaniensis Britton (Yellow Birch), Fagus
grandifolia Ehrh. (American Beech), Pinus strobus L. (Eastern White Pine), Tsuga
canadensis (L.) Carrière (Eastern Hemlock), Picea rubens Sarg. (Red Spruce),
and Abies balsamea (L.) Mill. (Balsam Fir). Dominant woody understory species
included Viburnum lantanoides Michx. (Hobblebush) and Vaccinium spp. (blueberries).
Forest harvesting in the focal area consisted of partial harvesting, typically
for production of woody biofuels.
Field sampling
We established 17 forest-edge transects, each 500 m in length and separated by
a minimum Euclidean distance of 500 m along gated logging roads and disused
railroad lines running into forest stands. No active use of roadways by vehicles
Northeastern Naturalist Vol. 22, No. 2
J.L.B. Pierce, et al.
2015
265
occurred during the study period. We set the transects at a minimum of 50 m from
the nearest paved highway. We only sampled transects with limited herbaceous
vegetation and leaf litter to ensure detection of Coyote scats.
We identified Coyote scats based on size, shape, and the presence of Whitetailed
Deer hair and larger bone fragments (Elbroch 2003). To determine the abundance
of Coyote scats, 2 technicians walked the transect once, recorded each scat,
marked its position, and tallied all scats regardless of their level of decomposition.
During preliminary studies, we also walked an additional 500 m beyond the end of
the transect to ensure that Coyote scats were not spatially clumped at a scale that
would influence our results; they were not.
We assessed browsing of woody plants by sampling one time within 5 paired
edge and interior plots (1 m x 2 m, from ground level to 2 m high). Paired plots were
located at 100-m intervals along the transects on alternating sides. We placed forestedge
plots with the outside edge of the plot (closest to the forest edge) established
on the woody stem nearest to the road- or railway. We placed the forest interior
plots 50-m into the forest from the edge plot perpendicular to the linear forest edge.
We chose 50 m as the distance from edge for our interior plots based on preliminary
observations indicating that this distance provided complete visual obscurity
at the majority of our sites. To enumerate browsing within each plot, we counted
the total number of terminal buds on twigs >2.5 cm in length for each woody plant
species present and the number browsed. We considered twigs browsed if the tip
of the apical meristem was missing. We only counted twigs if they occurred in the
3-dimensional sampling area. We then calculated the proportion of available twigs
of preferred woody plant species browsed within each plot. We used data provided
by the New York State Department of Environmental Conservation (NYSDEC) to
identify preferred browse species (NYSDEC 2014), and defined preferred browse
as all woody species not considered starvation food for White-tailed Deer.
We quantified escape cover at each browse plot using a 20 cm x 100 cm visual
obscurity board (divided into 80 alternating black and white squares) positioned
horizontally at 1 m from the ground surface and placed in the center of the plot.
We took 4 measurements of obscurity at each plot. For the edge plots, we took 2
measurements along the forest edge (1 in each direction down the roadway) and
2 perpendicular to the forest edge. In the interior plots, we took measurements of
obscurity in the 4 cardinal directions. For each measurement, an observer stood
15-m from the board and counted the number of squares that were not obscured in
any way; we then converted these data into the mean percent obscurity per plot.
Statistical analyses
We initially compared the number of preferred woody browse species in edge
versus interior plots using a 2-tailed t-test to assess if differences in food availability
could potentially mask any effects of differences in perceived predation
risk. To assess if higher use of edges by Coyotes reduced the amount of browsing
of woody plants at the landscape scale, we employed a linear regression with the
mean proportion of preferred woody species browsed per transect (i.e., averaged
Northeastern Naturalist
266
J.L.B. Pierce, et al.
2015 Vol. 22, No. 2
across edge and interior plots) as the dependent variable and the number of Coyote
scats as our independent variable. To test if higher use of edges by Coyotes corresponded
with more White-tailed Deer browsing in the forest interior, we first
quantified the difference in the proportion of preferred species browsed between
the edge and interior at each pair of plots. This metric provided a measure of the
relative difference in edge and interior browsing with a value of ~0 indicating no
difference between the edge and interior, positive numbers indicating more browsing
in the interior, and negative values indicating more browsing on the edge. We
then used linear regression to compare the mean difference in edge/interior browsing
per transect to the number of Coyote scats. To assess if escape cover mitigated
some of the effects of Coyote use on White-tailed Deer browsing, we compared the
mean preferred species browsed per transect with the mean percent escape cover.
All statistical analyses were conducted using Program R, version 3.0.2; we set α ≤
0.05 as the threshold for statistical significance.
Results
We found no significant difference in the amount of available preferred woody
browse in edge versus interior plots (t32 = 0.776, P = 0.443). The frequency of use
by Coyotes varied considerably among transects (mean ± SE = 3.2 ± 0.6, range =
0–9). Similarly, the proportion of preferred twigs browsed varied from low levels
of browsing to transects where almost half of the available twigs were browsed
(mean ± SE = 0.21 ± 0.03, range = 0.08–0.44). We found no significant relationship
between the mean proportion of preferred species browsed at the transect
level and the number of Coyote scats (F1,15 = 0.182, P = 0.675, r2 = 0.01; Fig. 1).
However, we did find a marginally significant effect of Coyotes on the difference
in the proportion of preferred species browsed between the edge and interior, with
a trend towards a higher proportion of browsing in the interior as the frequency of
Coyote scats increased (F1,15 = 4.452, P = 0.052, r2 = 0.23; slope = 0.036 ± 0.017,
y-intercept = -0.165 ± 0.068; Fig. 2). The mean escape cover at the transect scale
was not a significant driver of the proportion of preferred species browsed (F1,15 =
3.95, P = 0.065, r2 = 0.21), although we did observe a tendency towards a higher
proportion of browse where there was less escape cover (Fig. 3).
Discussion
Our results support the findings of prior research in showing that the response
of ungulates to predation pressure was scale-dependent (Altendorf et al. 2001,
Anderson et al. 2005, Jones and Hudson 2002, Kittle et al. 2008). Specifically, we
found that White-tailed Deer did not respond to predation risk (as inferred from
the number of Coyote scats) at the transect scale, but shifted to foraging more in
the forest interior. Similar risk-driven shifts in patterns of habitat selection have
been demonstrated for Elk in the presence of wolves in the western US (Creel et al.
2005); however, we are unaware of any previous evidence of a similar relationship
between Coyotes and White-tailed Deer in the northeast. The scale of the response
Northeastern Naturalist Vol. 22, No. 2
J.L.B. Pierce, et al.
2015
267
Figure 1. The relationship between the number of Coyote scats and the proportion of
preferred woody plant species browsed along 17 linear transects sampled in the northern
Adirondacks, NY. Each transect consisted of 5 browse plots on the forest edge and 5 browse
plots in the forest interior.
Figure 2. The relationship between the number of Coyote scats and the mean difference
between the proportion of preferred browsed tree species in the forest interior and forest
edge along 17 linear forest-edge transects sampled in the northern Adirondacks, NY. Positive
values represent more browse in the forest interior compared to the edge, and negative
values the opposite trend.
Northeastern Naturalist
268
J.L.B. Pierce, et al.
2015 Vol. 22, No. 2
we observed likely represented shifts in the feeding ecology of individual animals,
i.e., within-population effects, and supported the findings of previous studies in
showing that non-lethal effects of predators may be more prevalent than lethal effects
at the population scale (Altendorf et al. 2001, Brown 1999, Brown and Kotler
2004, Creel et al. 2005, Schmitz et al. 1997). We did not find strong evidence that
White-tailed Deer prefer to browse in areas with greater escape cover; this finding
may represent a flaw in the logic of our study in that denser cover may serve as
concealment for predators. However, we hypothesize that the trend we observed
toward less browsing in areas with more cover is due to dense cover in our study
area primarily representing Red Spruce and Balsam Fir, which are considered to be
starvation foods for White-tailed Deer.
Optimal foraging theory (MacArthur and Pianka 1966, Pyke 1984, Pyke et al.
1977) provides a strong theoretical framework for understanding the patterns we
observed in our data. In general, organisms should choose to adopt a foraging strategy
that minimizes costs, while maximizing energy intake (Brown and Kotler 2004,
Gilliam and Fraser 1987). The costs associated with foraging in a patch include
(1) the metabolic costs of foraging, (2) missed-opportunity costs of not being able
to engage in other activities, and (3) predation costs (Brown 1988). Because we
found no significant difference in the amount of available browse in edge versus
interior plots, we are able to exclude this potentially confounding variable from
our analyses and focus on differences in perceived predation risk. Individuals can
demonstrate habitat selection, i.e., the manifestation of optimal foraging theory
as it relates to the spatial distribution of organisms, at 2 scales: (1) the location of
Figure 3. The relationship between mean percent escape-cover and the mean proportion of
preferred woody plant species browsed per transect along 17 linear forest-edge transects
sampled in the northern Adirondacks, NY.
Northeastern Naturalist Vol. 22, No. 2
J.L.B. Pierce, et al.
2015
269
the home range in relation to available habitat, and (2) the areas utilized within the
home range (Anderson et al. 2005, Johnson 1980). Our study was conducted within
a relatively small spatial extent within forest-stand types known to host high abundances
of Coyotes in the focal region (Kays et al. 2008). These site characteristics
may explain why we did not observe patterns of browsing indicative of White-tailed
Deer relocating home ranges to areas with fewer Coyotes: dispersal to unfamiliar
territory would likely have been energetically costly, and the probability of Whitetailed
Deer finding an area with fewer Coyotes was low within our study region.
Conversely, the concentration of Coyotes along linear forest/shrub edges—as observed
in our focal region and in prior research (Knowlton et al. 1985)—represented
a more predictable risk that was avoided by moving into the forest interior. Optimal
foraging theory typically assumes that there is a trade-off between predation risk
and the quality of browse, i.e., the best places to browse are likely to have the highest
risk of predation (Brown and Kotler 2004). However, research has shown that
this is not always the case (Pierce et al. 2004). Our study provided evidence that the
most energetically efficient place for White-tailed Deer to browse woody vegetation
was on the forest edge because these areas were favored when Coyote use of
the edge was low.
Our study was based on the premise that the density of scat was indicative of
the intensity of use by Coyotes, and that this intensity of use translated into predation
risk for White-tailed Deer. This assumption was supported by prior research
in our study area that found that higher numbers of Coyote scat were associated
with a larger number of individuals at the landscape scale (Kays et al. 2008). Our
method for quantifying browsing inevitably included some twigs that were removed
by abiotic processes or by other species of browsers. Although this likely
led to an overestimation of the proportion of browsing, we have no reason to suspect
that abiotic damage to twigs varied among transects. Additional species that
may have consumed woody vegetation in the region include Lepus americanus
Erxleben (Snowshoe Hare) and Moose. Snowshoe Hares in the northern Adirondacks
tend to be concentrated in stands with dense cover (J. Favreau, School of
Natural Resources and Management, Paul Smith's College, Paul Smiths, NY,
unpubl. data) that are dissimilar to our focal stands; we observed very little sign
of browsed twigs that were cut (hares) rather than torn (ungulates). We excluded
the few twigs that were clearly browsed by Snowshoe Hares from our analyses.
Moose occur at very low densities in our focal region (Hickey 2008, Sage et
al. 2003), with the NYSDEC estimating a maximum of 1000 individuals in the
2,400,000-ha park (i.e., less than 0.0004/ha). In comparison, White-tailed Deer densities
in the central Adirondacks range from 0.019/ha to 0.104/ha (Sage et al 2003).
Browsing by White-tailed Deer is a dominant force that shapes forest structure
and function in the northeastern US (Sage et al. 2003). Of particular concern
to land managers is the potential for White-tailed Deer browsing to reduce the
recruitment of commercially valuable tree species including birch species and
Eastern Hemlock (Rooney and Waller 2003). Current approaches to mitigating the
effects of deer browsing on forest composition tend to focus on directly reducing
Northeastern Naturalist
270
J.L.B. Pierce, et al.
2015 Vol. 22, No. 2
the abundance of White-tailed Deer through hunting (Sage et al. 2003). However,
the efficacy of hunting as a tool for widespread reductions in White-tailed Deer
densities is uncertain (Brown et al. 2000). Natural processes such as predation may
offer an alternative approach to hunting if they result in patterns of White-tailed
Deer browsing and forest regeneration that meet forest-management objectives.
While restoring top carnivores following their extirpation is controversial, understanding
the consequences of natural colonization/recolonization events such as
the movement of Coyotes into the northeastern US on patterns of browsing is of
great importance. Our study, while limited in scope, indicated that Coyotes may
be driving local browsing patterns primarily through behaviorally mediated indirect
effects as has been demonstrated with other taxa (Creel et al. 2005, Schmitz
et al. 1997). This finding suggests that future research should assess how browsing
affects the recruitment of tree species, and how the variation in the ecology of
Coyotes reported across the northeastern US may influence their relationship with
White-tailed Deer and patterns of browsing.
Acknowledgments
We would like to thank Steve Campbell and Celia Evans for helping to guide this research
project, and Ben Tabor and Ed Reed for advice on White-tailed Deer browsing in the
region. We would also like to thank the manuscript editor and an anonymous reviewer for
feedback on an earlier draft of this manuscript. This project was supported by a National
Science Foundation S-STEM award to Paul Smith’s College.
Literature Cited
Altendorf, K.B., J.W. Laundré, C.A. López González, and J.S. Brown. 2001. Assessing
effects of predation risk on foraging behavior of Mule Deer. Journal of Mammalogy
82:430–439.
Alverson, W.S., D.M. Waller, and S.L. Solheim. 1988. Forests too deer: Edge effects in
northern Wisconsin. Conservation Biology 2:348–358.
Anderson, D.P., M.G. Turner, J.D. Forester, J. Zhu, M.S. Boyce, H. Beyer, and L. Stowell.
2005. Scale-dependent summer-resource selection by reintroduced Elk in Wisconsin,
USA. Journal of Wildlife Management 69:298–310.
Beschta, R.L., and W.J. Ripple. 2009. Large predators and trophic cascades in terrestrial
ecosystems of the western United States. Biological Conservation 142:2401–2414.
Bressette, J.W., H. Beck, and V.B. Beauchamp. 2012. Beyond the browse line: Complex
cascade effects mediated by White-tailed Deer. Oikos 121:1749–1760.
Brown J.S. 1988. Patch use as an indicator of habitat preference, predation risk, and competition.
Behavioral Ecology and Sociobiology 22:37–47.
Brown, J.S. 1999. Vigilance, patch use, and habitat selection: Foraging under predation risk.
Evolutionary Ecology Research 1:49–71.
Brown, J.S., and B.P. Kotler. 2004. Hazardous-duty pay and the foraging cost of predation.
Ecology Letters 7:999–1014.
Brown, T.L., D.J. Decker, S.J. Riley, J.W. Enck, T.B. Lauber, P.D. Curtis, and G.F. Mattfeld.
2000. The future of hunting as a mechanism to control White-tailed Deer populations.
Wildlife Society Bulletin 28:797–807.
Northeastern Naturalist Vol. 22, No. 2
J.L.B. Pierce, et al.
2015
271
Creel, S., J. Winnie., Jr., B. Maxwell, K. Hamlin, and M. Creel. 2005. Elk alter habitat selection
as an antipredator response to wolves. Ecology 86:3387–3397.
Elbroch, M. 2003. Mammal Tracks and Sign: A Guide to North American Species. Stackpole
Books, Mechanicsburg, PA. 779 pp.
Fener, H.M., J.R. Ginsberg, E.W. Sanderson, and M.E. Gompper. 2005. Chronology of
the range expansion of the Coyote, Canis latrans, in New York. The Canadian Field-
Naturalist 119:1–5.
Frair, J.L., E.H. Merrill, D.R. Visscher, D. Fortin, H.L. Beyer, and J.M. Morales. 2005.
Scales of movement by Elk (Cervus elaphus) in response to heterogeneity in forage
resources and predation risk. Landscape Ecology 20:273–287.
Gilliam, J.F., and D.F. Fraser. 1987. Habitat selection under predation hazard: Test of a
model with foraging minnows. Ecology 68:1856–1862.
Gompper, M.E. 2002a. The ecology of Northeast Coyotes: Current knowledge and priorities
for future research. Wildlife Conservation Society Working Paper No. 17. Bronx,
NY. 46 pp.
Gompper, M.E. 2002b. Top carnivores in the suburbs? Ecological and conservation issues
raised by colonization of northeastern North America by Coyotes. Bioscience
52:185–190.
Halofsky, J.S., and W.J. Ripple. 2008. Fine-scale predation risk on Elk after wolf reintroduction
in Yellowstone National Park, USA. Oecologia 155:869–877.
Hebblewhite, M., E.H. Merrill, and T.L. McDonald. 2005. Spatial decomposition of predation
risk using resource-selection functions: An example in a wolf–Elk predator–prey
system. Oikos 111:101–111.
Heske, E.J., S.K. Robinson, and J.D. Brawn. 1999. Predator activity and predation on songbird
nests on forest-field edge in east-central Illinois. Landsc ape Ecology 14:345–354.
Hickey, L. 2008. Assessing re-colonization of Moose in New York with HSI models. Alces
44:117–126.
Horsley, S.B., S.L. Stout, and D.S. deCalesta. 2003. White-tailed Deer impact on the vegetation
dynamics of a northern hardwood forest. Ecological Applications 13:98–119.
Husseman, J.S., D.L. Murray, G. Power, C. Mack, C.R. Wenger, and H. Quigley. 2003.
Assessing differential prey-selection patterns between two sympatric large carnivores.
Oikos 101:591–601.
Johnson, D.H. 1980. The comparison of usage and availability measurements for evaluating
resource preference. Ecology 61:65–71.
Jones, P.F., and R.J. Hudson. 2002. Winter-habitat selection at three spatial scales by
American Elk, Cervus elaphus, in west-central Alberta. The Canadian Field-Naturalist
116:183–191.
Kays, R.W., M.E. Gompper, and J.C. Ray. 2008. Landscape ecology of eastern Coyotes
based on large-scale estimates of abundance. Landscape Ecology 18:1014–1027.
Kittle, A.M., J.M. Fryxell, G.E. Desy, and J. Hamr. 2008. The scale-dependent impact
of wolf predation risk on resource selection by three sympatric ungulates. Oecologia
157:163–175.
Kittredege, D.B., and P.M.S. Ashton. 1995. Impact of deer browsing on regeneration
in mixed stands in southern New England. Northern Journal of Applied Forestry
12:115–120.
Knowlton, F.F., L.A. Windberg, and C.E. Wahlgren. 1985. Coyote vulnerability to several
management techniques. Great Plains Wildlife Damage Control Workshop Proceedings.
172:165–176.
Northeastern Naturalist
272
J.L.B. Pierce, et al.
2015 Vol. 22, No. 2
Kolbe, J.A., J.R. Squires, D.H. Pletscher, and L.F. Ruggiero. 2010. The effects of snowmobile
trails on Coyote movements within Lynx home ranges. The Journal of Wildlife
Management 71:1409–1418.
MacArthur, R.H., and E.R. Pianka. 1966. On optimal use of a patchy environment. The
American Naturalist 100:603–609.
Messier, F., C. Barrette, and J. Huot. 1986. Coyote predation on a White-tailed Deer population
in southern Quebec. Canadian Journal of Zoology 64:1134–1136.
Mladenhoff, D.J., T.A. Sickley, R.G. Haight, and A.P. Wydeven. 1995. A regional landscape
analysis and prediction of favorable Gray Wolf habitat in the Northern Great Lakes region.
Conservation Biology 9:279–294.
New York State Department of Environmental Conservation (NYSDEC). 2014. Winter
deer foods. Available online at http://www.dec.ny.gov/animals/7195.html. Accessed 1
February 2014.
Patterson, B.R., and F. Messier. 2001. Social organization and space use of Coyotes in
eastern Canada relative to prey distribution and abundance. Journal of Mammalogy
82:463–477.
Pierce, B.M., R.T. Bowyer, and V.C. Bleich. 2004. Habitat selection by Mule Deer: Forage
benefits or risk of predation? Journal of Wildlife Management 68:533–541.
Pyke, C.R. 1984. Optimal foraging theory: A critical review. Annual review of Ecology and
Systematics 15:523–575.
Pyke, C.R., H.R. Pulliam, and E.L. Charnov. 1977. Optimal foraging: A selective review of
theory and tests. Quarterly Review of Biology 52:137–154.
Ripple, W.J., and R.L. Beschta. 2004. Wolves and the ecology of fear: Can predation risk
structure ecosystems? Bioscience 54:755–766.
Rooney, T.P., and D.M. Waller. 2003. Direct and indirect effects of White-tailed Deer in
forest ecosystems. Forest Ecology and Management 181:165–176.
Roy, L.D., and M.J. Dorrance. 1985. Coyote movements, habitat use, and vulnerability in
central Alberta. The Journal of Wildlife Management 49:307–313.
Sage, R.W., Jr., W.F. Porter, and H.B. Underwood. 2003. Windows of opportunity: Whitetailed
Deer and the dynamics of northern hardwood forests of the northeastern US.
Journal for Nature Conservation 10:1–8.
Schmitz, O.J., A.P. Beckerman, and K. O’Brien. 1997. Behaviorally mediated trophic cascades:
Effects of predation risk on food-web interactions. Ecology 78:1388–1399.
Waller, D.M., and W.S. Alverson. 1997. The White-tailed Deer: A keystone herbivore.
Wildlife Society Bulletin 25:217–226.
Warsen, S.A. 2012. Evolving niche dynamics of Coyotes in the Adirondack Mountains of
New York: Long-term dietary trends and interspecific competition. M.Sc. Thesis. State
University of New York College of Environmental Science and Forestry, Syracuse, NY.
90 pp.
Whittington, J., C.C. St. Clair, and G. Mercer. 2005. Spatial responses of wolves to roads
and trails in mountain valleys. Ecological Applications 15:543–553.
Williamson, S.J., and D.H. Hirth. 1985. An evaluation of edge use by White-tailed Deer.
Wildlife Society Bulletin 13:252–257.
Woods, K.D., D.J. Hicks, and J. Schultz. 2012. Losses in understory diversity over three
decades in an old-growth cool-temperate forest in Michigan, USA. Canadian Journal of
Forest Research 42:532–549.