Potential for Monitoring Eastern Small-footed Bats on
Talus Slopes
Paul R. Moosman, Jr., Daniel P. Warner, R. Hardy Hendren, and Micah. J. Hosler
Northeastern Naturalist, Volume 22, Issue 1 (2015): NENHC-1–NENHC-13
Full-text pdf (Accessible only to subscribers. To subscribe click here.)
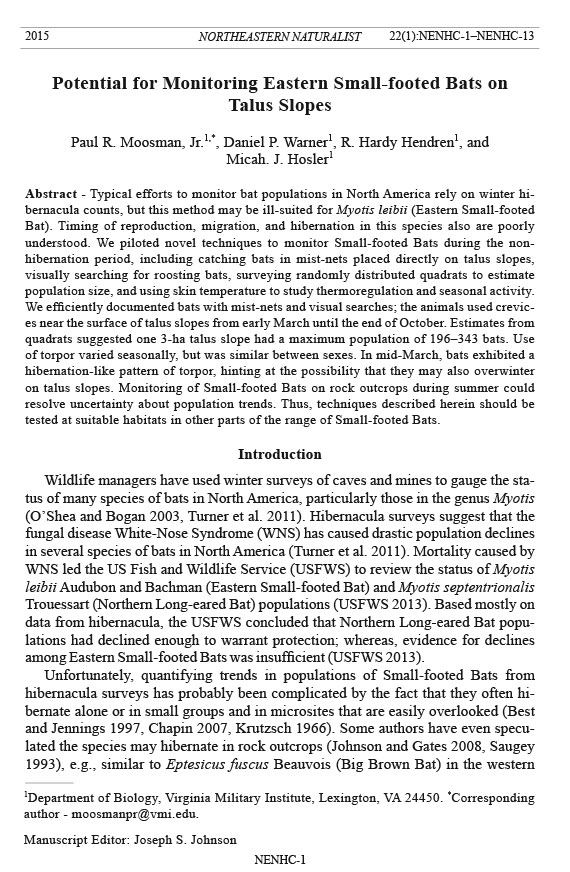
Access Journal Content
Open access browsing of table of contents and abstract pages. Full text pdfs available for download for subscribers.
Current Issue: Vol. 30 (3)
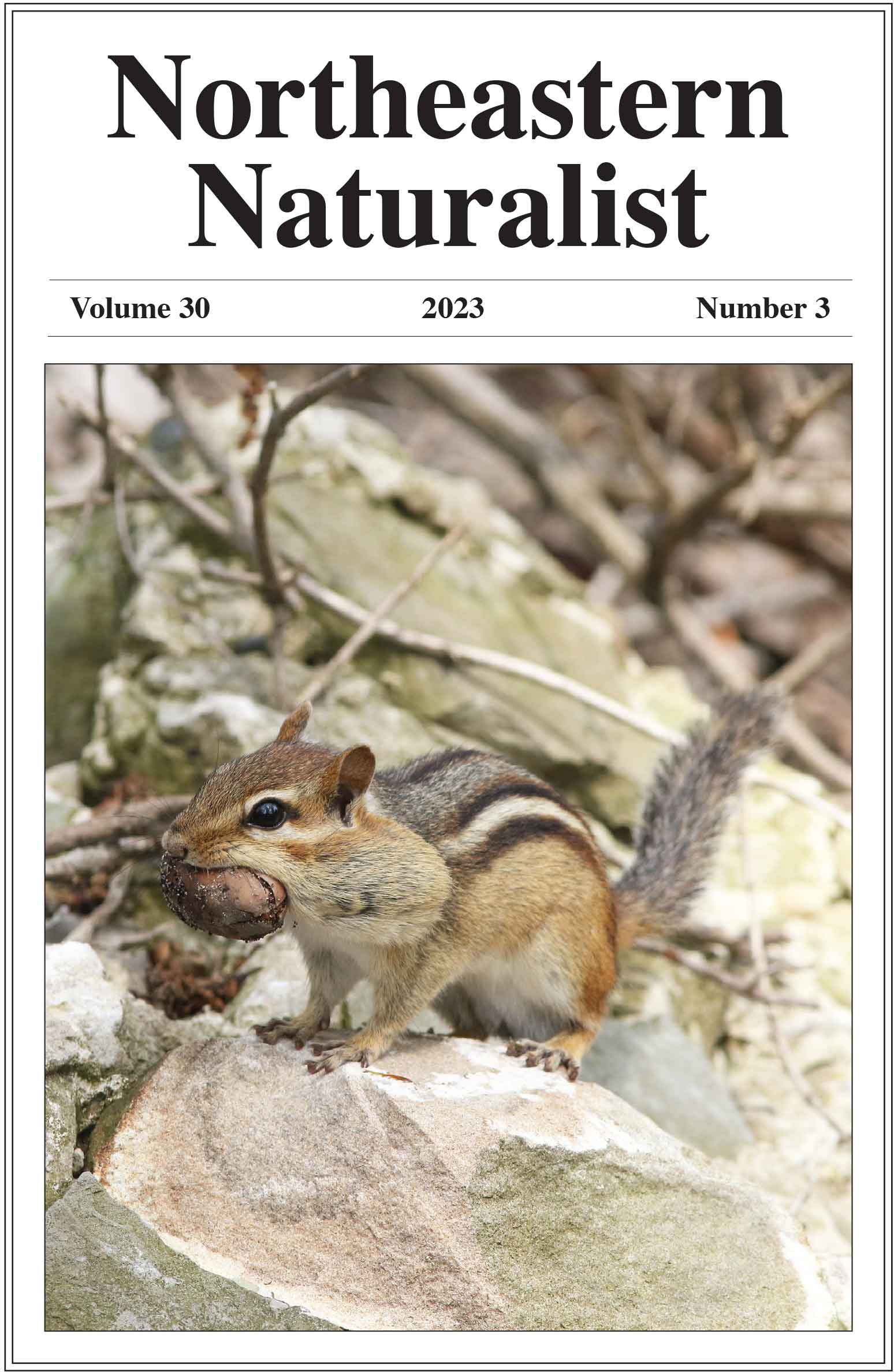
Check out NENA's latest Monograph:
Monograph 22
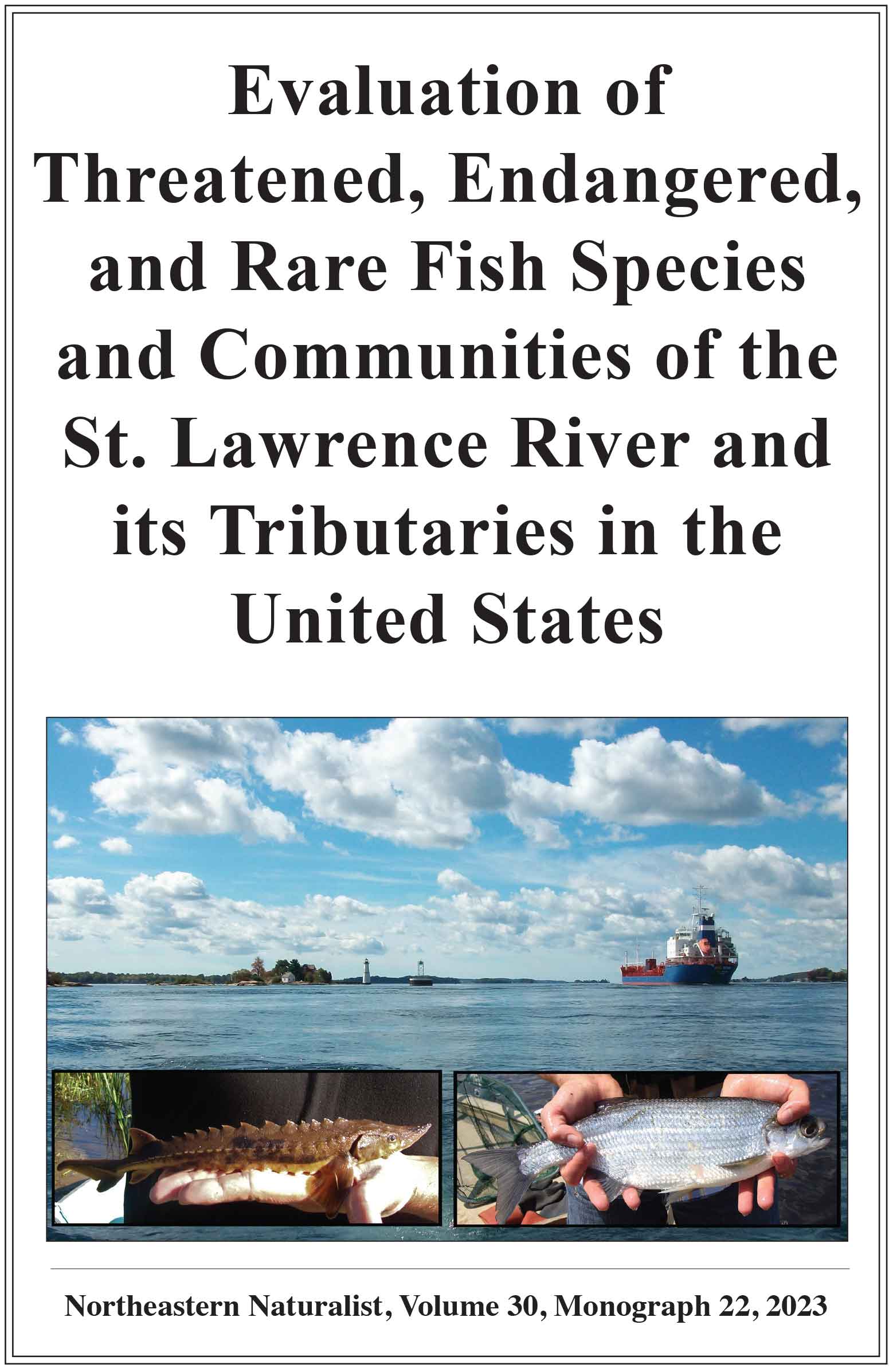
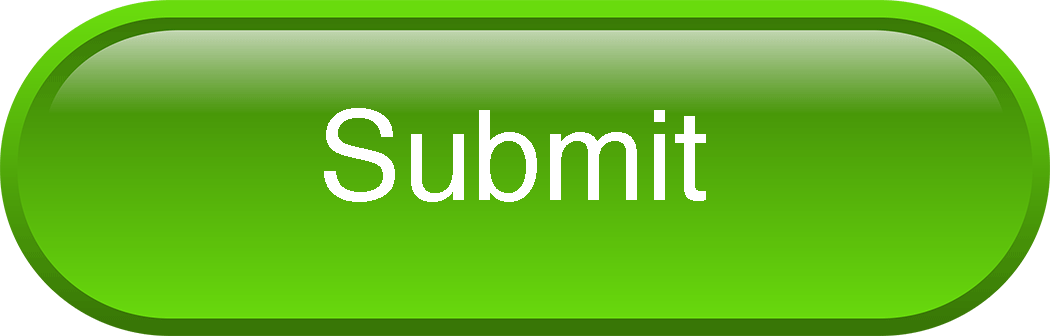
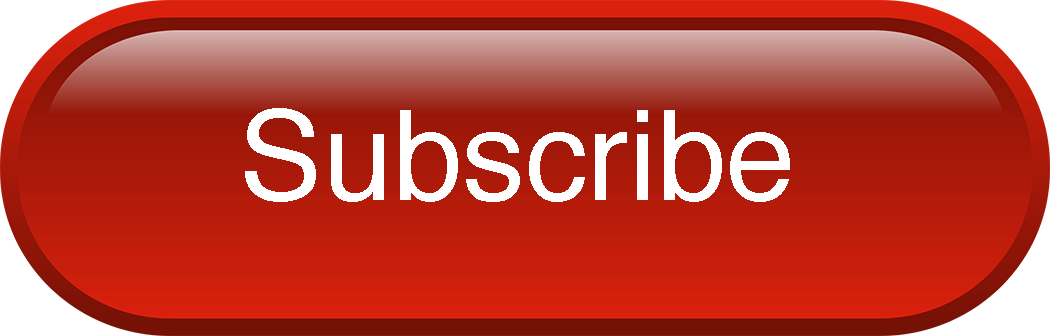
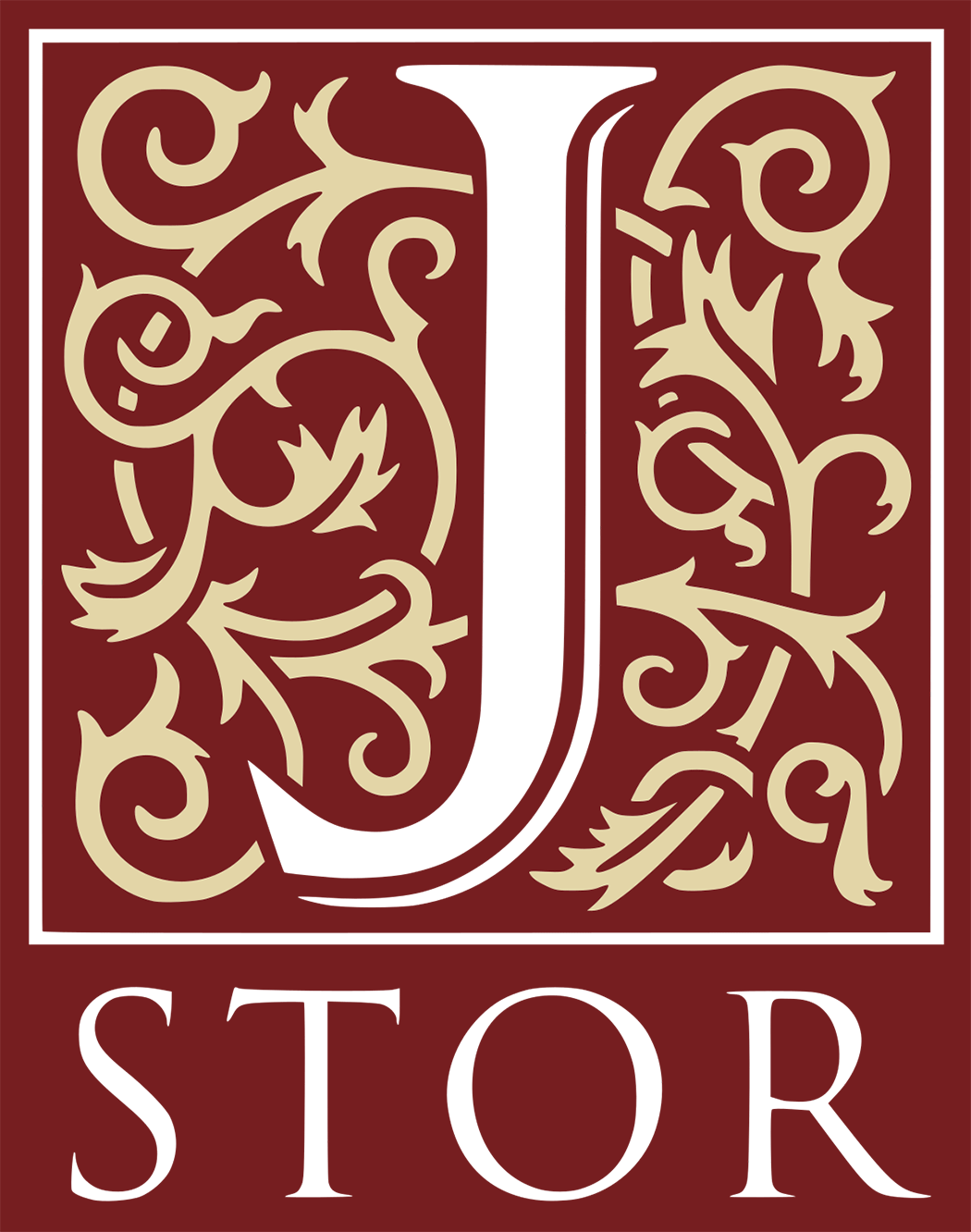


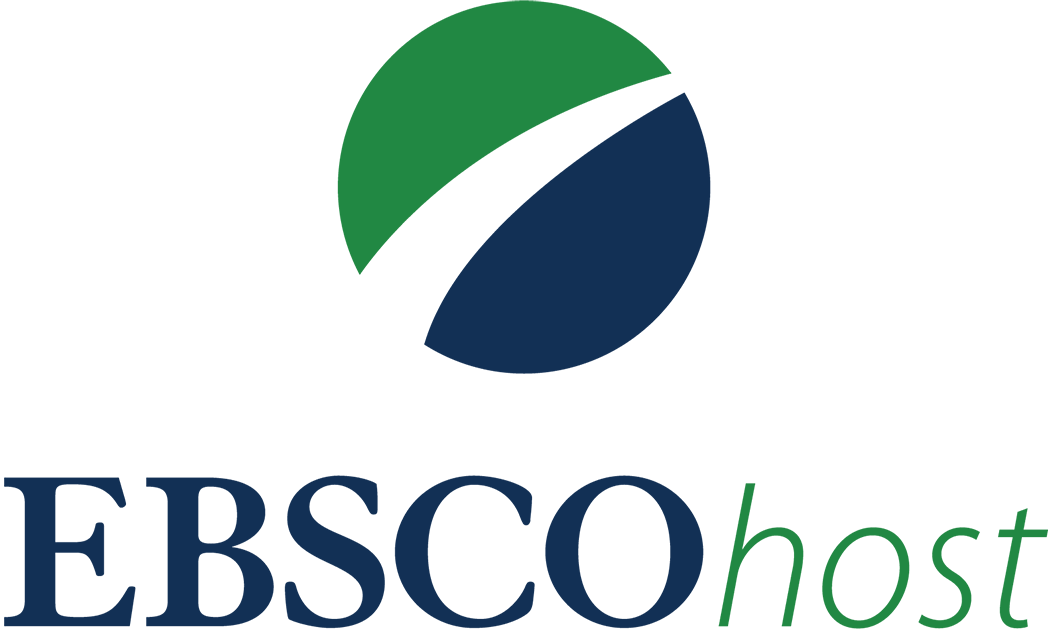

Northeastern Naturalist Vol. 22, No. 1
P.R. Moosman, Jr., D.P. Warner, R.H. Hendren, and M.J. Hosler
2015
NENHC-1
2015 NORTHEASTERN NATURALIST 22(1):NENHC-1–NENHC-13
Potential for Monitoring Eastern Small-footed Bats on
Talus Slopes
Paul R. Moosman, Jr.1,*, Daniel P. Warner1, R. Hardy Hendren1, and
Micah. J. Hosler1
Abstract - Typical efforts to monitor bat populations in North America rely on winter hibernacula
counts, but this method may be ill-suited for Myotis leibii (Eastern Small-footed
Bat). Timing of reproduction, migration, and hibernation in this species also are poorly
understood. We piloted novel techniques to monitor Small-footed Bats during the nonhibernation
period, including catching bats in mist-nets placed directly on talus slopes,
visually searching for roosting bats, surveying randomly distributed quadrats to estimate
population size, and using skin temperature to study thermoregulation and seasonal activity.
We efficiently documented bats with mist-nets and visual searches; the animals used crevices
near the surface of talus slopes from early March until the end of October. Estimates from
quadrats suggested one 3-ha talus slope had a maximum population of 196–343 bats. Use
of torpor varied seasonally, but was similar between sexes. In mid-March, bats exhibited a
hibernation-like pattern of torpor, hinting at the possibility that they may also overwinter
on talus slopes. Monitoring of Small-footed Bats on rock outcrops during summer could
resolve uncertainty about population trends. Thus, techniques described herein should be
tested at suitable habitats in other parts of the range of Small-footed Bats.
Introduction
Wildlife managers have used winter surveys of caves and mines to gauge the status
of many species of bats in North America, particularly those in the genus Myotis
(O’Shea and Bogan 2003, Turner et al. 2011). Hibernacula surveys suggest that the
fungal disease White-Nose Syndrome (WNS) has caused drastic population declines
in several species of bats in North America (Turner et al. 2011). Mortality caused by
WNS led the US Fish and Wildlife Service (USFWS) to review the status of Myotis
leibii Audubon and Bachman (Eastern Small-footed Bat) and Myotis septentrionalis
Trouessart (Northern Long-eared Bat) populations (USFWS 2013). Based mostly on
data from hibernacula, the USFWS concluded that Northern Long-eared Bat populations
had declined enough to warrant protection; whereas, evidence for declines
among Eastern Small-footed Bats was insufficient (USFWS 2013).
Unfortunately, quantifying trends in populations of Small-footed Bats from
hibernacula surveys has probably been complicated by the fact that they often hibernate
alone or in small groups and in microsites that are easily overlooked (Best
and Jennings 1997, Chapin 2007, Krutzsch 1966). Some authors have even speculated
the species may hibernate in rock outcrops (Johnson and Gates 2008, Saugey
1993), e.g., similar to Eptesicus fuscus Beauvois (Big Brown Bat) in the western
1Department of Biology, Virginia Military Institute, Lexington, VA 24450. *Corresponding
author - moosmanpr@vmi.edu.
Manuscript Editor: Joseph S. Johnson
Northeastern Naturalist
NENHC-2
P.R. Moosman, Jr., D.P. Warner, R.H. Hendren, and M.J. Hosler
2015 Vol. 22, No. 1
US (Neubaum et al. 2006). The hibernating habits of Small-footed Bats might explain
why estimates of declines from WNS have been substantially lower in winter
counts (12% decline) versus mist-net surveys in summer (68–84% declines) (Francl
et al. 2012, Moosman et al. 2013, Turner et al. 2011). Although acoustic surveys
have been used to study responses to WNS in other species of bats, none have included
Small-footed Bats because they are infrequently detected and their calls are
prone to misidentification (Brooks 2011, Dzal et al. 2011, Ford et al. 2011).
Uncertainty over the status of Small-footed Bats is likely to continue unless alternative
means to monitor their populations are developed. Monitoring populations
during the non-hibernation period has rarely been attempted in North America because
bats are generally dispersed on the landscape and difficult to count. However,
a growing body of evidence suggests Small-footed Bats are concentrated at exposed
rock outcrops during the non-hibernation period (Johnson and Gates 2008, Johnson
et al. 2011, Saugey et al. 1993). Visually searching under rocks has also resulted in
both accidental and intentional encounters with the species (Roble 2004, Whitby et
al. 2013). These observations suggest monitoring Small-footed Bats may best be
accomplished at rock outcrops, but techniques to do so have not been developed.
We explored novel methods to study Small-footed Bats on talus slopes in
the Blue Ridge Mountains of Virginia. Our goals were to test the feasibility of:
(1) capturing bats in mist-nets placed directly on talus slopes, (2) visually searching
for roosting bats, and (3) combining these methods with remote monitoring of skin
temperature (Tsk) to infer timing of important life-history events, including migration
or hibernation. We also explored the potential for using surveys of randomly
distributed quadrats to estimate local population size.
Methods
Study sites
We conducted our research at a 3.0-ha talus slope known as the Devil’s Marbleyard
(hereafter, Marbleyard) in the James River Face Wilderness in Rockbridge
County, 2 small (0.15-ha and 0.3-ha) talus slopes that were part of a network of
rock outcrops in the Saint Mary’s Wilderness (hereafter, St. Mary’s) in Rockbridge
County, and a 3.34-ha talus slope at Sherando Lake Recreation Area in Augusta
County (hereafter, Sherando). Presence of Small-footed Bats at Sherando had been
previously determined by mist-netting and radio-telemetry, but the species had not
been documented at Marbleyard or St. Mary’s prior to the present study (P.R. Moosman
et al., unpubl. data). We selected sites by examining SPOT satellite imagery in
Google Earth Pro (Google, Inc., Mountain View, CA) for patches of exposed rock.
Study sites were on primarily south-facing slopes and were composed of sedimentary
rock ranging in size from boulders several meters wide to weathered fragments
less than 0.25 m wide. We measured the non-forested area of each talus slope using the
polygon tool in Google Earth Pro. Elevations ranged from 530–600 m at the bases
to 700–800 m at the tops of the talus slopes. The surrounding forest was mixeddeciduous
with overstory trees dominated by Quercus prinus L. (Chestnut Oak),
Q. rubra L. (Northern Red Oak), Q. coccinea Münchhausen (Scarlet Oak), Pinus
Northeastern Naturalist Vol. 22, No. 1
P.R. Moosman, Jr., D.P. Warner, R.H. Hendren, and M.J. Hosler
2015
NENHC-3
virginiana Miller (Virginia Pine), and Acer rubrum L. (Red Maple) (Mengkak and
Castleberry 2008). Edges of the talus slopes had relict shoots of Castanea dentata
Marshall (American Chestnut).
Visual searches
We conducted timed diurnal visual searches for roosting bats at Marbelyard
on 22 visits from 5 June 2013 to 30 March 2014, on 4 visits to Sherando from 18
July to 25 October 2013, and on 2 visits to St. Mary’s on 16 and 24 July 2013. We
quantified search effort in person-hours (e.g., 1 person-hour = 1 person searching
for 1 h, or 2 people searching for 0.5 h). Searching ceased when at least 1 bat was
located or after 2.5 person-hours if no bats were located. Searches were conducted
by 1–3 of the authors using LED lights to inspect crevices for roosting bats. While
walking on talus slopes, we wore helmets and chose our footsteps carefully to avoid
stepping on unstable rocks or potential roosts. These precautions were particularly
necessary on portions of talus slopes with small rocks because they tended to be the
least stable. We captured by hand bats that were alone or in small groups (composed
of 1 adult female and 1–3 juveniles) by coaxing them out of the crevice using 2
flexible 1.0-m-long vinyl-coated wire probes. To minimize disturbance to colonies,
we did not capture bats in maternity colonies (>1 adults).
Mist-netting
We deployed mist nets with 38-mm mesh in 2 manners. Because Marbleyard
lacked corridors traditionally considered suitable for surveys with mist-nets, we
placed two 12-m-long x 3-m-high nets end to end directly on the talus slope (hereafter,
each pair will be considered a single net). We placed nets perpendicular to the
forest edge with one end against the tree-line and the other end extending toward
the center of the boulder field. Nets were attached to light-weight telescoping aluminum
poles that were anchored to the substrate by guy ropes and were operated
from 15 min before sunset until 1.25 h after sunset to capture bats that had recently
emerged from crevices on the slope. We conducted sampling in this manner on 4
June, 26 June, and 24 September.
At Sherando, we placed mist nets in traditional arrangements of 6–9-m-long nets
stacked vertically to block a stream corridor adjacent to the talus slope (hereafter,
each stacked set of nets is considered a single net). We placed 2 nets >30 m apart
and operated them 15 min before sunset until 4 h after dark on 13 and 23 September.
We removed the captured bats from the net; weighed them to the nearest 0.1 g
using a spring scale (Pesola AG, Baar, Switzerland); determined their sex, age, and
reproductive state; and placed a numbered aluminum band (Porzana Limited, East
Sussex, UK) on the forearm (left for females, right for males) of each bat before
release. Handling of bats followed Sikes et al. (2011).
Quantifying populations
We assessed population density of Small-footed Bats at Marbleyard by surveying
random quadrats. Because no studies had reported use of talus slopes by Smallfooted
Bats in closed-canopy forest, and crevices in this habitat at our study site
Northeastern Naturalist
NENHC-4
P.R. Moosman, Jr., D.P. Warner, R.H. Hendren, and M.J. Hosler
2015 Vol. 22, No. 1
were filled with leaf litter, we only considered as available roosting habitat parts
of the slope that appeared non-forested on SPOT satellite images. We sampled
occurrence of bats in available habitat by searching all crevices in 13 randomly
distributed, circular 78.5-m2 quadrats (i.e., 3.4% of the talus slope). We determined
quadrat placement by overlaying a grid with cells representing 10 m x 10 m onto
an aerial image of the site and created a pool of 284 points at the intersections of
grid lines, each marking the center of a potential quadrat. Quadrats were selected
randomly from this pool with SPSS version 20.0 (IBM Corp., Armonk, NY) and
observers navigated to the center of each with a Triton 500 GPS device with 3–5-m
accuracy (Magellan, Santa Clara, CA). We used a measuring tape to define a 5-mradius
circle as the boundary of the quadrat, and 2 of the authors searched every
crevice in the quadrat, recording the number of bats and suitable crevices present.
We considered crevices suitable if they were large enough for a Small-footed Bat to
fit inside (i.e., some portion ≥0.75 cm wide) and were ≤4 cm wide at the narrowest
(the widest roosting cavity we have documented; P.R. Moosman, pers. observ.). We
marked crevices with colored chalk to prevent mistakes during counting. Because
Eastern Small-footed Bats often use a different roost each day, make consecutive
movements of less than 50 m (Johnson and Gates 2008; Johnson et al. 2011; P.R. Moosman,
pers. observ.), and all quadrats could not be surveyed in the same day, we surveyed
quadrats in 3 groups (on 2, 5, and 12 July 2013) that were >50 m apart to reduce
chances of recounting the same bats.
We explored 2 methods to estimate population. In the most conservative method,
we calculated population estimates by scaling up the 95% confidence limits for the
number of bats counted to the entire area of talus slope. In the second method, we
made population estimates by multiplying a pooled occupancy rate (proportion of
suitable crevices with bats) by the 95% confidence limits of the projected number
of crevices on the entire slope. These methods were meant to represent crude and
ecological estimates of population density, respectively. We based confidence limits
in both methods on the Poisson distribution (Zar 1999).
Monitoring of skin temperature
A temperature-sensitive 0.24-g radio transmitters (LB-2XT, Holohil Systems
Ltd., Carp, ON, Canada; representing 4–6% of body weight) was used to study
Tsk and activity of a subset of 16 bats (9 males, 7 females) at Marbleyard and Sherando.
We radio-tagged 3 females and 1 male bat twice as opportunity allowed.
This tagging provided data from 3 scrotal and 6 non-scrotal males and 1 pregnant, 3
lactating, and 5 non-reproductive females. We collected data longitudinally over the
study by radio-tagging 1–2 bats on average every 10.8 ± 0.5 days. Skin temperature
(Tsk) was recorded every 2 min over the life of each transmitter using an SRX-DL
data-logging receiver (Lotek Wireless, Inc., New Market, ON, Canada) at each site,
attached to a 6-m-tall omni-directional antenna and a 3-element directional antenna,
powered by a 12v deep-cycle battery and 2 Solaris 26 photovoltaic arrays (Brunton
Outdoor, Inc., Riverton, WY). We used data from radio-transmitters to assess if
bats were present at the site (i.e., within range of the data-logger) and whether they
Northeastern Naturalist Vol. 22, No. 1
P.R. Moosman, Jr., D.P. Warner, R.H. Hendren, and M.J. Hosler
2015
NENHC-5
were active or torpid. Data-loggers typically detected radio-transmitters of bats
roosting less than 100 m away; therefore, we assumed data were from bats on or near the
talus slopes. We defined torpor as any Tsk below the lowest active Tsk recorded at
emergence, as was the standard in previous studies (Cryan and Wolf 2006, Solick
and Barclay 2006). Active Tsk in our study ranged from 30.1 °C to 38.1 °C; thus, we
defined torpor as Tsk < 30 °C. Maximum Tsk while roosting (usually between noon
and emergence) also was recorded. Hourly air temperature (Ta) was recorded using
a Thermocron iButton (NexSens Technology, Inc., Fairborn, OH) placed 2 m above
the ground in a shaded location in the forest below each talus slope as a basis for
comparison. Work was conducted under a collecting permit (#048316) issued by
the Virginia Department of Game and Inland Fisheries, and approved by the Animal
Subjects Committee at the Virginia Military Institute.
We quantified the number of days between the first and last instance each bat
was detected by the data-logger, as well as the number of days bats spent absent
or out of range. We pooled data for each bat that was radio-tagged twice and then
assessed potential gender-differences between these variables using two-tailed
independent-sample t-tests. Seasonal variation in the above variables was assessed
using Pearson bivariate correlations, with date expressed as a Julian number. We
quantified patterns of Tsk using incidence of torpor (the ratio of days bats were
detected in torpor versus the number of days they were detected at active Tsk). To
ensure data were not collected from transmitters after they had been shed by bats,
we only considered bouts of torpor as valid if followed by an arousal to active Tsk.
We assessed effects of season (Julian day) on proportion of days bats were recorded
in torpor using a linear regression with incidence of torpor as the dependent variable
and start date of each bat’s monitoring period as the independent variable.
Statistical analyses were performed using SPSS with α of 0.05. All results are reported
as mean ± se, unless noted otherwise.
Results
Across sites, timed visual searches resulted in the discovery of an average
of 3.1 ± 1.1 bats per person-hour of searching between 5 June and 3 September
(Fig. 1). During this period, we documented bats in 100% of searches at Marbleyard
(6 visits), St. Mary’s (2 visits), and Sherando (2 visits). We documented
bats more sporadically between 20 September and 29 October (40%, or 2 of 5
visits at Marbleyard and Sherando combined). We discovered a total of 27 bats
in 23 roosts during timed visual searches, including: 20 roosts with solitary bats,
2 roosts with 2 bats, and 1 roost with 3 bats. Instances of bats roosting together
included a pair of adults of unknown sex on 5 June, a female roosting with a 3.5-
g juvenile on 16 July, and a trio composed of a lactating female with 2 volant
4.25-g juveniles on 18 July.
We encountered an additional 8 roosts by chance while setting up mist nets or
walking to quadrats to conduct population estimates, including: 5 encounters with
solitary bats, 1 with 2 adults, and 2 with maternity colonies. We discovered the first
colony on 15 June when bats were heard vocalizing from a series of crevices in a
Northeastern Naturalist
NENHC-6
P.R. Moosman, Jr., D.P. Warner, R.H. Hendren, and M.J. Hosler
2015 Vol. 22, No. 1
6-m-tall x 7-m-wide boulder. Size of this colony was unknown. Vocalizations lead
to the discovery of another maternity colony on 5 July, which had ~20 bats congregating
in direct sunlight at the mouth of a large vertical crevice in a >3-m-wide
boulder. In addition to finding Small-footed Bats in their roosts, we saw one (later
determined to be a post-lactating female) flying during the day .
Mist nets on the talus slope captured 13 Small-footed Bats, with an average of
4.3 ± 2.1 bats per net-night (or 3.8 ± 1.8 per hour of sampling), not including recaptures.
We captured all of these bats less than 10 m from the forest edge, on the up-slope
side of nets an average of 24 ± 2 min after sunset (range = 14–48 min after sunset).
Bats captured in mist-nets on the talus slope included 9 non-scrotal adult males and
4 adult females (2 pregnant, 1 lactating, 1 post-lactating, and 1 with no evidence
of reproduction). We recaptured 2 bats (a male first caught on 3 June and a female
first caught on 26 June) on our final mist-netting attempt. One scrotal adult male
Small-footed Bat was captured in mist-nets at Sherando on 13 September.
Data from mist-nets and visual searches combined resulted in the following
observations (first and last dates): pregnancy (4–26 June), lactation (26 June–18
July), maternity colonies and small mother-pup groups (15 June–18 July), nonreproductive
adult females (29 August 2013–11 March 2014), and scrotal males
(3 September–29 October). Additionally, we made single observations of a postlactating
female on 16 July and two 4.25-g volant juveniles on 18 July. The last date
we documented bats in autumn was 29 October. We failed to detect bats during 6
searches between 6 November and 2 March. Encounters with bats resumed on 11
and 23 March. Surveys of random quadrats at Marbleyard resulted in the discovery
of 6 bats out of 337 crevices searched. We estimated that the entire talus slope
had 8873–11,018 suitable crevices, and calculated the size of the population to be
48–343 bats when we used the crude estimate and 158–196 bats using the ecological
estimate (95% CI).
Figure 1. Phenology of Eastern Small-footed Bats on talus slopes in Virginia, inferred from
visual searches for roosting bats and bats captured in mist-nets. Bars represent the number
of bats observed relative to visual search effort.
Northeastern Naturalist Vol. 22, No. 1
P.R. Moosman, Jr., D.P. Warner, R.H. Hendren, and M.J. Hosler
2015
NENHC-7
Of the 16 bats radio-tagged, 11 were detected by the data-logger every day, 3
went undetected for 2–7 days (3.3 ± 1.5 days), and 2 were never detected. The time
elapsed between the first and last instances bats were detected (5.1 ± 0.9 days per
radio-transmitter) and the number of days they went undetected (1.0 ± 0.5 days
per radio-transmitter) was similar between sexes (P = 0.36, P = 0.73, respectively)
and showed no discernable seasonal pattern (P = 0.14 and P = 1.0, respectively).
Time of year had a significant negative effect on incidence of torpor, with use of
torpor greatest in spring and lowest in autumn (F1,15 = 10.28, P = 0.006; Fig. 2).
Maximum active Tsk was 35.7 ± 1.0 °C, whereas Tsk when roosting reached 1.9 ± 0.6
°C above active Tsk. Bats typically exhibited a daily Tsk pattern from June to October
characterized by torpor while in the roost, during which Tsk mimicked Ta. That is, Tsk
gradually rose from a minimum Tsk near dawn to a maximal Tsk after noon, before
declining until bats aroused and emerged from the roost at sunset (Fig. 3). In contrast,
bats in March of 2014 remained in a steady state of relatively deep torpor
(mean Tsk = 13.8 ± 0.3 °C) for >2 days (perhaps >4 days), interrupted by ~1 h-long
periods of arousal to active Tsk (Fig. 3). During the same period, mean Ta was 3.8 ±
2.3 °C, with daily minima ranging from 1.5 to -6.0 °C
Figure 2. Seasonal changes in the incidence of torpor, expressed as the ratio of the number
of days in which torpor was documented versus the number of days in which bats exhibited
active skin temperature. Data were from 8 males (hollow symbols) and 7 females (solid
symbols) from summer to autumn of 2013 and spring of 2014. The center line represents
the trend, and the upper and lower lines represent the 95% confi dence intervals.
Northeastern Naturalist
NENHC-8
P.R. Moosman, Jr., D.P. Warner, R.H. Hendren, and M.J. Hosler
2015 Vol. 22, No. 1
Discussion
Our results suggest that surveying for Eastern Small-footed Bats on talus slopes
is highly effective. Bats were efficiently encountered in visual searches and by
placing mist nets directly on talus slopes during summer. The efficiency of both
methods suggests either is preferable over the customary means of placing mistnets
on roads or permanent bodies of water (unless perhaps such features occur
particularly close to suitable rock outcrops). The next-best published capture success
was only ~1 Small-footed Bat per net-night (or 0.25 bats per hour of sampling)
using mist-nets placed on forest edges and roads 10–500 m from a boulder-covered
dam where bats roosted (Moosman et al. 2013). Francl et al. (2012) reported much
lower rates of capture (less than 0.1 Small-footed Bat per net-night) using standard mist-net
Figure 3. Skin temperature of 2 Eastern Small-footed Bats (solid symbols) illustrating differences
between patterns in summer (A) and those in early spring (B). Air temperature in
the shade near the talus slope (hollow symbols) is shown for comparison. Isolated solid
symbols are erroneous readings by the data-logger.
Northeastern Naturalist Vol. 22, No. 1
P.R. Moosman, Jr., D.P. Warner, R.H. Hendren, and M.J. Hosler
2015
NENHC-9
protocols that do not target rock outcrops, i.e., those designed for Myotis sodalis
Miller and Allen (Indiana Bat). We note that in our study, bats were caught immediately
after sunset, apparently as they traveled down the edge of the talus slope.
Therefore, researchers planning to use the technique we outline here should ensure
nets are deployed before dusk and attempt to place them perpendicular to the forest
edge or other terrain features likely to funnel bats.
Mist-nets placed on the talus slope resulted in the greatest number of bats captured
per hour of sampling (not including time to set up nets); thus, we preferred
this method when attempting to acquire several bats to radio-tag. However, visual
searches were preferable for simply confirming that bats were present at a site. The
effort needed to visually locate Small-footed Bats during the present study was
similar to that reported by Whitby et al. (2013) at rock-outcrops in Illinois, except
we rarely needed to move rocks in order to search crevices. It should be noted
that search effort needed to locate bats appeared to vary between talus slopes as a
function of boulder size. Sherando had the smallest boulders (therefore the greatest
number of crevices) and required the most time to survey. Visual searches also
generated some novel observations, e.g., bats making brief flights between roosts
during the day, and bats apparently basking in sunlight outside the entrances to
roosts. Basking of course is a common behavior in many species of animals, but it
has been rarely documented in bats (see Slough 2009).
The population estimates we derived from random surveys are particularly
promising. Although the technique should be refined, we present these data to
stimulate a much needed discussion. Both of our estimates indicate the population
at Marbleyard was small compared to summer populations of other bat species,
but still greater than the largest numbers of Small-footed Bats reported from hibernacula
surveys (Johnson and Gates 2008, Turner et al. 2011). We suspect the
true population size at Marbleyard was between the totals which we derived using
crude and ecological estimates. Differences between the two methods of estimating
population size are important to consider. The crude method of estimation did not
take into account variation in availability of potential crevices and it was negatively
affected by the fact that many quadrats contained no bats (i.e., quadrat data were
zero-inflated). The ecological estimate accounted for variation in availability of
suitable crevices, and likely was less affected by zero-inflation, but it unrealistically
assumed no variation in occupancy rates. These findings suggests population
estimates can be improved by (1) quantifying variation in occupancy rates and
(2) reducing zero-inflation (e.g., by increasing quadrat size and conducting surveys
during parts of the year when females roost alone). Other ways to improve modeling
of error also are possible and should be explored (Dupuis et al. 2011).
Combined observations indicate both sexes were present on the talus slopes
throughout the study. Maternity season occurred from early June to mid-July, and
scrotal males were observed from early September to mid-October, perhaps suggesting
mating occurs on talus slopes. Visual surveys from November through the
winter failed to detect bats, indicating they either migrated to hibernacula elsewhere
or moved into microsites on the talus slopes that we could not survey effectively
Northeastern Naturalist
NENHC-10
P.R. Moosman, Jr., D.P. Warner, R.H. Hendren, and M.J. Hosler
2015 Vol. 22, No. 1
(i.e., deep crevices or rip-rap caves). Bats appeared to have returned to crevices
near the surface by early March. These data generally agree with what little information
about the phenology of Small-footed Bats has been published (see Best and
Jennings 1997, Johnson and Gates 2008).
We were surprised that the incidence of torpor in bats we studied was similar
between sexes and that use of torpor declined from spring through autumn. Studies
of other bats species in temperate regions have reported differences in thermoregulatory
patterns based on sex or reproductive status (Cryan and Wolf 2003, Solick
and Barclay 2006), and more frequent use of torpor in spring and autumn than in
summer (Becker et al. 2013, Wojciechowski et al. 2006). These discrepancies might
be resolved with additional data, including for bats later into autumn, but it is also
possible the thermal properties of talus slopes allow unique thermoregulatory opportunities
for Small-footed Bats. The talus slopes we studied provided particularly
warm microclimates, relative to Ta. During summer and autumn daily Tsk often began
~3 °C or more above Ta in the morning and rose to 11 °C or more above Ta in the
warmest part of the afternoon. Furthermore, Tsk during the day sometimes reached
40 °C and typically exceeded that recorded at night when bats were presumably
flying. Roosting in such conditions could minimize the need for active arousals, but
perhaps also increase costs from evaporative water loss. Indeed, other authors have
suggested Small-footed Bats select sites near water (Johnson et al. 2011).
Although we did not observe bats at our study sites in winter (including during
searches of 3 rip-rap caves), the question of whether Small-footed Bats hibernate
in talus slopes remains unanswered. Skin temperatures of 3 bats captured from particularly
deep crevices in March resembled those of hibernating bats. We captured
these bats after brief warm spells. Two were torpid when discovered and a 3rd bat
was sunning itself outside its roost but crawled back inside as we approached. Two
cold fronts arrived less than 24 h after bats were released, bringing low temperatures of
-6 °C and covering the talus slope with snow. Skin temperatures were remarkably
stable during this time despite drastic fluctuations in Ta and they were within the
range of temperatures expected in caves in eastern North America during winter,
including those used by Small-footed Bats (Moore and Sullivan 1978, Swezey and
Garrity 2009, Webb et al. 1996). The bats in our study only aroused to active Tsk
for ~1-h long periods in March, a pattern that was distinct from the daily pattern of
torpor and arousals demonstrated during summer and autumn. We suspect bats in
March remained in the crevices from which they were captured, but it is also possible
they moved to other crevices during arousals.
In conclusion, Eastern Small-footed Bats are more accessible than has commonly
been assumed, and monitoring their populations on talus slopes is one
likely solution to the uncertainty about population trends described in the USFWS
(2013) finding. Talus slopes are not the only habitats the species occupies,
but they are abundant in the Appalachian Mountains and they are detectable in
remote-sensing imagery (O’Keefe and LaVoie 2010, Whitby et al. 2013). Use of
thermal refugia on talus slopes in spring further supports the notion that Eastern
Small-footed Bats could hibernate outside of caves and mines. This situation
Northeastern Naturalist Vol. 22, No. 1
P.R. Moosman, Jr., D.P. Warner, R.H. Hendren, and M.J. Hosler
2015
NENHC-11
would support the hypothesis that Small-footed Bats are at lower risk of mortality
from WNS (Langwig et al. 2012), but it also would raise doubts about the
efficacy of using cave and mine surveys to monitor their populations. Identifying
techniques to monitor populations of Eastern Small-footed Bats should be a major
conservation priority. In particular, the methods described herein should be tested
at suitable habitats in other parts of the Eastern Small-footed Bat’s range and, if
determined to be reliable, used to begin monitoring.
Acknowledgments
Funding was provided by the Virginia Department of Game and Inland Fisheries through
a Wildlife Restoration Grant from the US Fish and Wildlife Service, and the Virginia Military
Institute’s Center for Undergraduate Research. We thank the US Forest Service for allowing
us to conduct this study. Additionally, we are indebted to R. Reynolds, K. Powers,
R. Humston, D. Wright, K. Simms, H. Thomas, J. Huth, E. Hill, P. Burke, and W. Ford for
the help they provided with various aspects of the research.
Literature Cited
Becker, N.I., M. Tschapka, E.K.V. Kalko, and J.A. Encarnação. 2013. Balancing the energy
budget in free-ranging male Myotis daubentonii bats. Physiological and Biochemical
Zoology 86(3):361–369.
Best, T.L., and J.B. Jennings. 1997. Myotis leibii. Mammalian Species 547:1–6.
Brooks, R.T. 2011. Declines in summer bat activity in central New England 4 years following
the initial detection of White-nose Syndrome. Biodiversity Conservation
20:2537–2541.
Chapin, B.R. 2007. Eastern Small-footed Myotis: Myotis leibii (Audubon and Bachman,
1942). Pp. 189–192, In M.K. Trani, W.M. Ford, and B.R. Chapman (Eds.). The Land
Manager’s Guide to Mammals of the South. The Nature Conservancy, Durham, NC,
546 pp.
Cryan, P.M., and B.O. Wolf. 2006. Sex differences in the thermoregulation and evaporative
water loss of a heterothermic bat, Lasiurus cinereus, during its spring migration. Journal
of Experimental Biology 206:3381–3390.
Dupuis, J.A., F. Bled, and J. Joachim. 2011. Estimating the occupancy rate of spatially rare
or hard-to-detect species: A conditional approach. Biometrics 67:290–298.
Dzal, Y., L.P. McGuire, N. Veselka, and M.B. Fenton. 2011. Going, going, gone: The impact
of White-nose Syndrome on the summer activity of Little Brown Bat (Myotis lucifugus).
Biology Letters 7:392–394.
Ford, W.M., E.R. Britzke, C.A. Dobony, J.L. Rodrigue, and J.B. Johnson. 2011. Patterns
of acoustical activity on bats prior to and following White-nose Syndrome occurrence.
Journal of Fish and Wildlife Management 2:125–134.
Francl, K.E., W.M. Ford, D.W. Sparks, and V.E. Brack, Jr. 2012. Capture and reproductive
trends in summer bat communities in West Virginia: Assessing the impact of White-nose
Syndrome. Journal of Fish and Wildlife Management 3:33–42.
Johnson, J.B., and J.E. Gates. 2008. Spring migration and roost selection of female Myotis
leibii in Maryland. Northeastern Naturalist 15:453–460.
Johnson, J.S., J.D. Kiser, K.S. Watrous, and T.S. Peterson. 2011. Day-roosts of Myotis
leibii in the Appalachian Ridge and Valley of West Virginia. Northeastern Naturalist
18:95–106.
Northeastern Naturalist
NENHC-12
P.R. Moosman, Jr., D.P. Warner, R.H. Hendren, and M.J. Hosler
2015 Vol. 22, No. 1
Krutzsch, P H. 1966. Remarks on Silver-haired and Leib’s bats in eastern United States.
Journal of Mammalogy 47:121.
Langwig, K.E., W.F. Frick, J.T. Bried, A.C. Hicks, T.H. Kunz, and A.M. Kilpatrick. 2012.
Sociality, density-dependence, and microclimates determine the persistence of populations
suffering from a novel fungal disease, White-nose Syndrome. Ecology Letters
15:1050–1057.
Mengkak, M.T., and S.B. Castleberry. 2008. Influence of acorn mast on Allegheny Woodrat
population trends in Virginia. Northeastern Naturalist 15:475–484.
Moore, G.W., and G.N. Sullivan. 1978. Speleology, the Study of Caves. 2nd Edition. Zephyrus
Press, Teaneck, NJ. 150 pp.
Moosman, P.R., Jr., J.P. Veilleux, G.W. Pelton, and H.H. Thomas. 2013. Changes in capture
rates in a community of bats in New Hampshire during the progression of White-nose
Syndrome. Northeastern Naturalist 20:552–558.
Neubaum, D.J., T.J. O’Shea, and K.R. Wilson. 2006. Autumn migration and selection of
rock crevices as hibernacula by Big Brown Bats in Colorado. Journal of Mammalogy
87:470–479.
O’Keefe, J.M., and M. LaVoie. 2010. Maternity colony of Eastern Small-footed Myotis
(Myotis leibii) in a historic building. Southeastern Naturalist 10:381–383.
O’Shea, T.J., and M.A. Bogan (Eds.). 2003. Monitoring trends in bat populations of the
United States and territories: Problems and prospects. US Geological Survey, Biological
Resources Discipline, Information and Technology, Fort Collins, CO. Report USGS/
BRD/ITR-2003-0003. 274 pp.
Roble, S.M. 2004. Notes on an autumn roost of an Eastern Small-footed Bat (Myotis leibii).
Banisteria 23:42–44.
Saugey, D.A., V.R. McDaniel, D.R. England, M.C. Rowe, L.R. Chandler-Mozisek, and
B.G. Cochran. 1993. Arkansas range extensions of the Eastern Small-footed Bat (Myotis
leibii) and Northern Long-eared Bat (Myotis septentrionalis), and additional county
records for the Silver-haired Bat (Lasionycteris noctivagans), Hoary Bat (Lasiurus
cinereus), and Rafinesque’s Big-eared Bat (Plecotus rafinesquii). Proceedings of the
Arkansas Academy of Science 47:102–106.
Sikes, R.S., W.L. Gannon, and the Animal Care and Use Committee of the American Society
of Mammalogists. 2011. Guidelines of the American Society of Mammalogists for
the use of wild mammals in research. Journal of Mammalogy 92:235–253.
Slough, B.G. 2009. Behavioural thermoregulation by a maternity colony of Little Brown
Bats in the Yukon. Northwestern Naturalist 90:47–51.
Solick, D.I., and R.M.R. Barclay. 2006. Thermoregulation and roosting behavior of reproductive
and nonreproductive female Western Long-eared Bats (Myotis evotis) in the
Rocky Mountains of Alberta. Canadian Journal of Zoology 84:589–599.
Swezey, C.S., and C.P. Garrity. 2009. Geographical and geological data from caves and
mines infected with White-nose Syndrome (WNS) before September 2009 in the eastern
United States. Journal of Cave and Karst Studies 73:125–157.
Turner, G.G., D.M. Reeder, and J.T.H. Coleman. 2011. A five-year assessment of mortality
and geographic spread of White nose Syndrome in North American bats and a look into
the future. Bat Research News 52:13–27.
US Fish and Wildlife Service (USFWS). 2013. 12-Month finding on a petition to list the
Eastern Small-footed Bat and the Northern Long-eared Bat as endangered or threatened
species. Federal Register 78:61,046–61,080.
Northeastern Naturalist Vol. 22, No. 1
P.R. Moosman, Jr., D.P. Warner, R.H. Hendren, and M.J. Hosler
2015
NENHC-13
Webb, P.I., J.R. Speakman, and P.A. Racey. 1996. How hot is a hibernaculum? A review
of the temperatures at which bats hibernate. Canadian Journal of Zoology 74:761–765.
Whitby, M., S. Bergeson, T. Carter, S. Rutan, and R. McClanahan. 2013. The discovery of
a reproductive population of Eastern Small-footed Bat, Myotis leibii, in southern Illinois
using a novel survey method. American Midland Naturalist 169:229–233.
Wojciechowski, M.S., M. Jefimow, and E. Tęgowska. 2006. Environmental conditions,
rather than season, determine torpor use and temperature selection in large Mouse-eared
Bats (Myotis myotis). Comparative Biochemistry and Physiology 147:828–840.
Zar, J.H. 1999. Biostatistical Analysis, 4th Edition. Prentice Hall, Upper Saddle River, NJ.
663 pp.