Interannual Survival of Myotis lucifugus (Chiroptera:
Vespertilionidae) near the Epicenter of White-Nose Syndrome
Jonathan D. Reichard, Nathan W. Fuller, Alyssa B. Bennett, Scott R. Darling, Marianne S. Moore, Kate E. Langwig, Emily D. Preston, Susi von Oettingen, Christopher S. Richardson, and D. Scott Reynolds
Northeastern Naturalist, Volume 21, Issue 4 (2014): N56–N59
Full-text pdf (Accessible only to subscribers. To subscribe click here.)
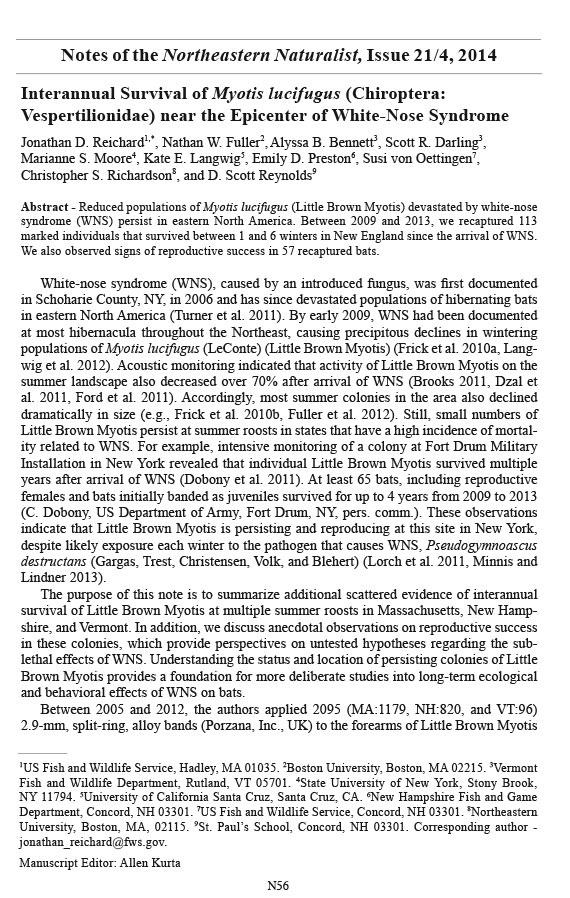
Access Journal Content
Open access browsing of table of contents and abstract pages. Full text pdfs available for download for subscribers.
Current Issue: Vol. 30 (3)
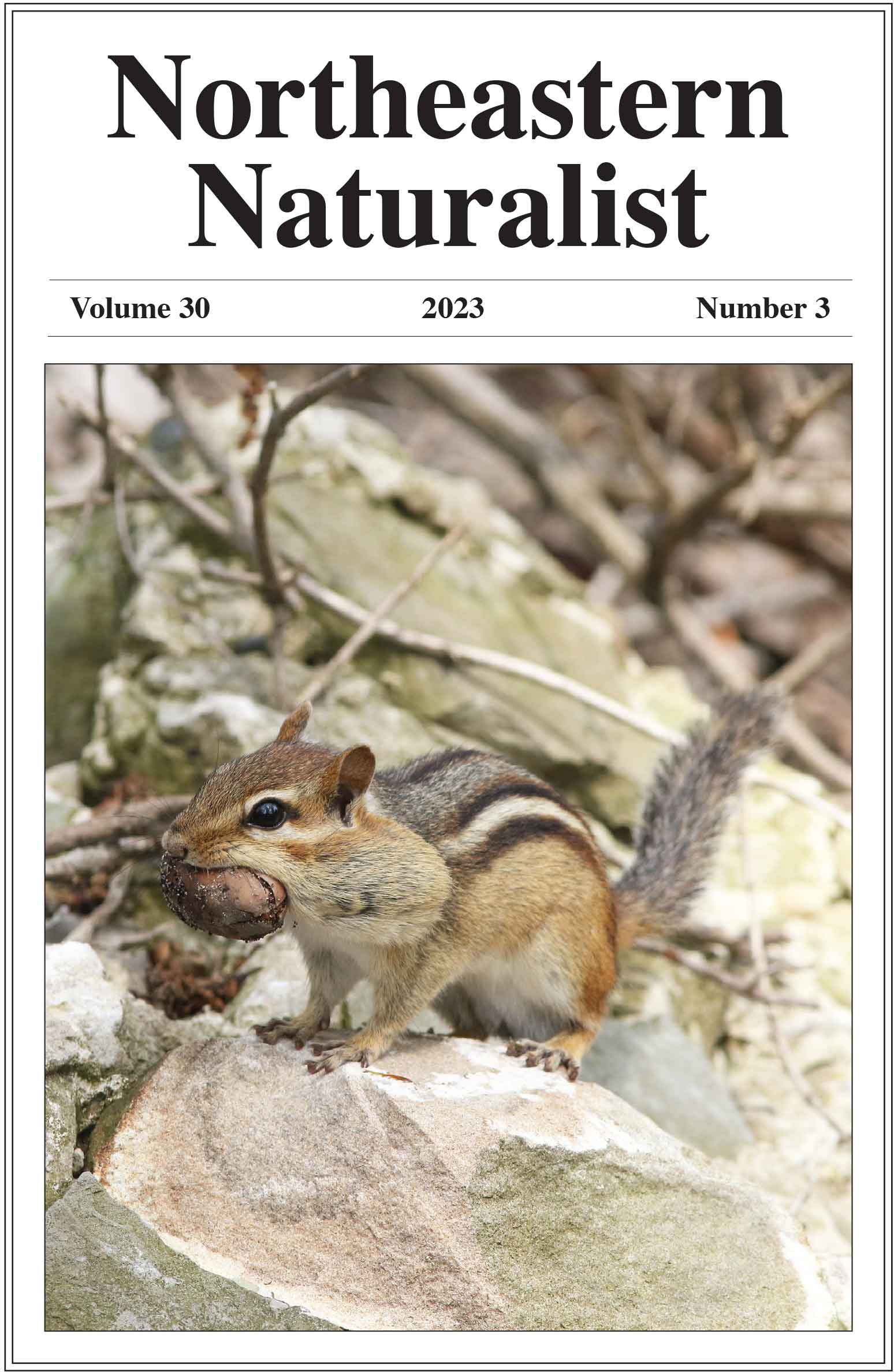
Check out NENA's latest Monograph:
Monograph 22
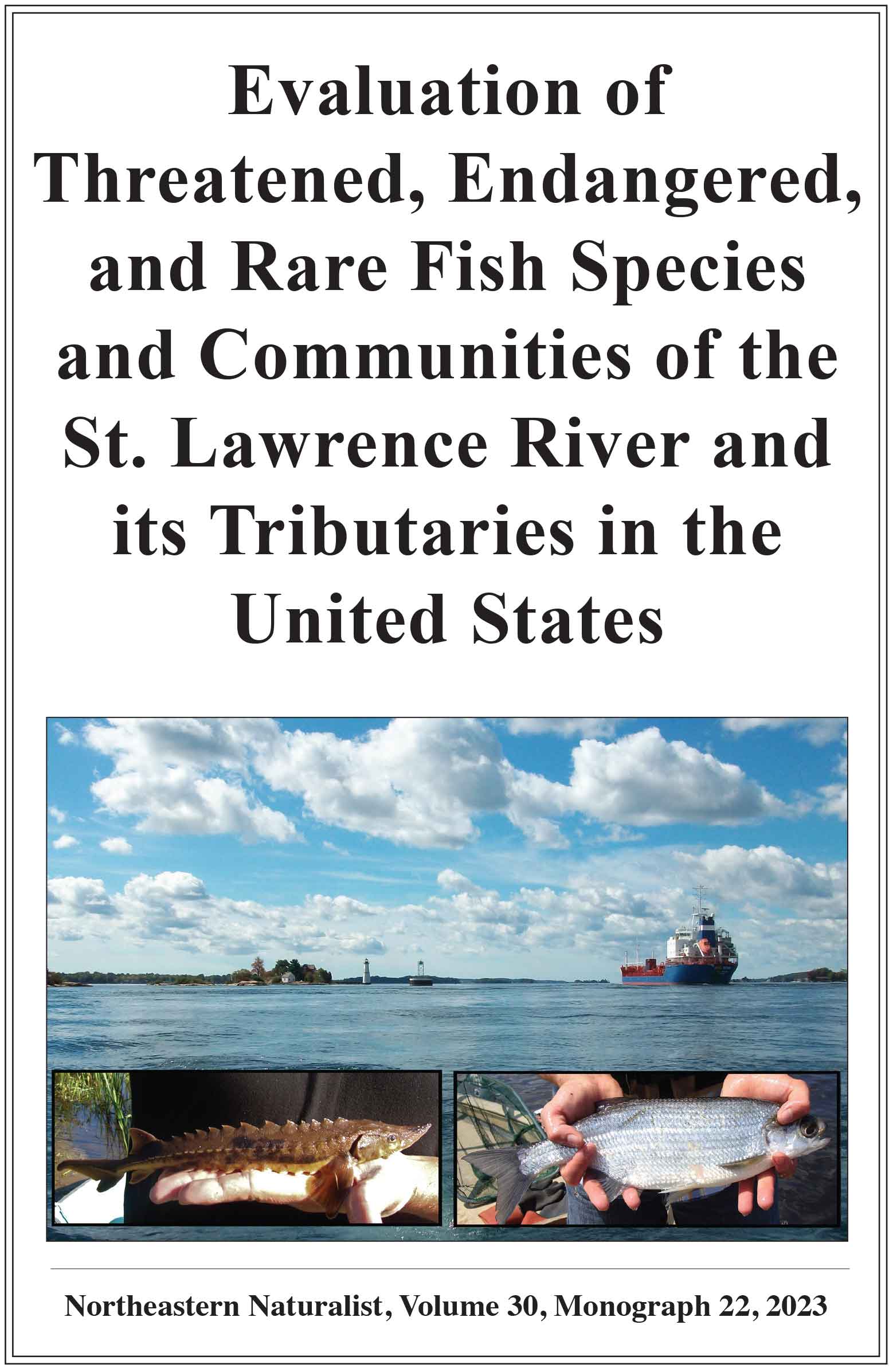
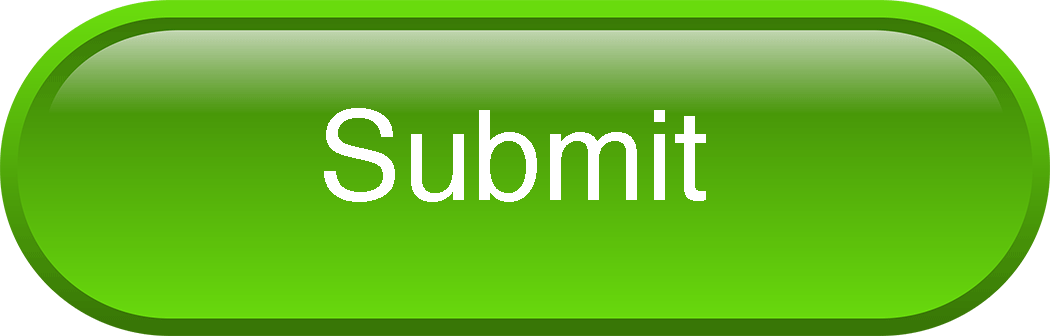
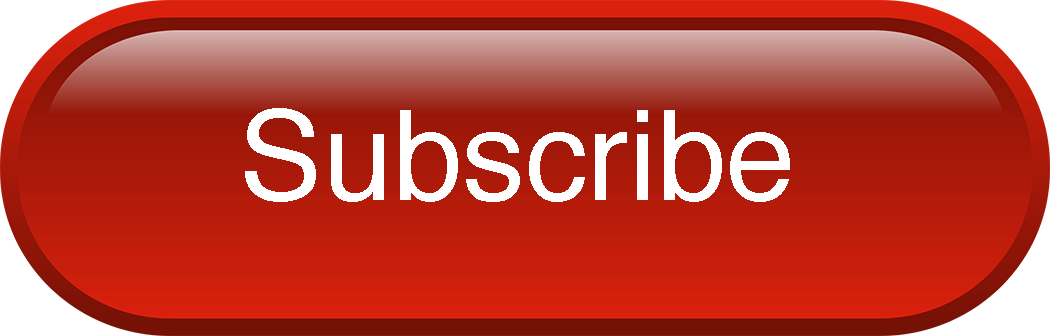
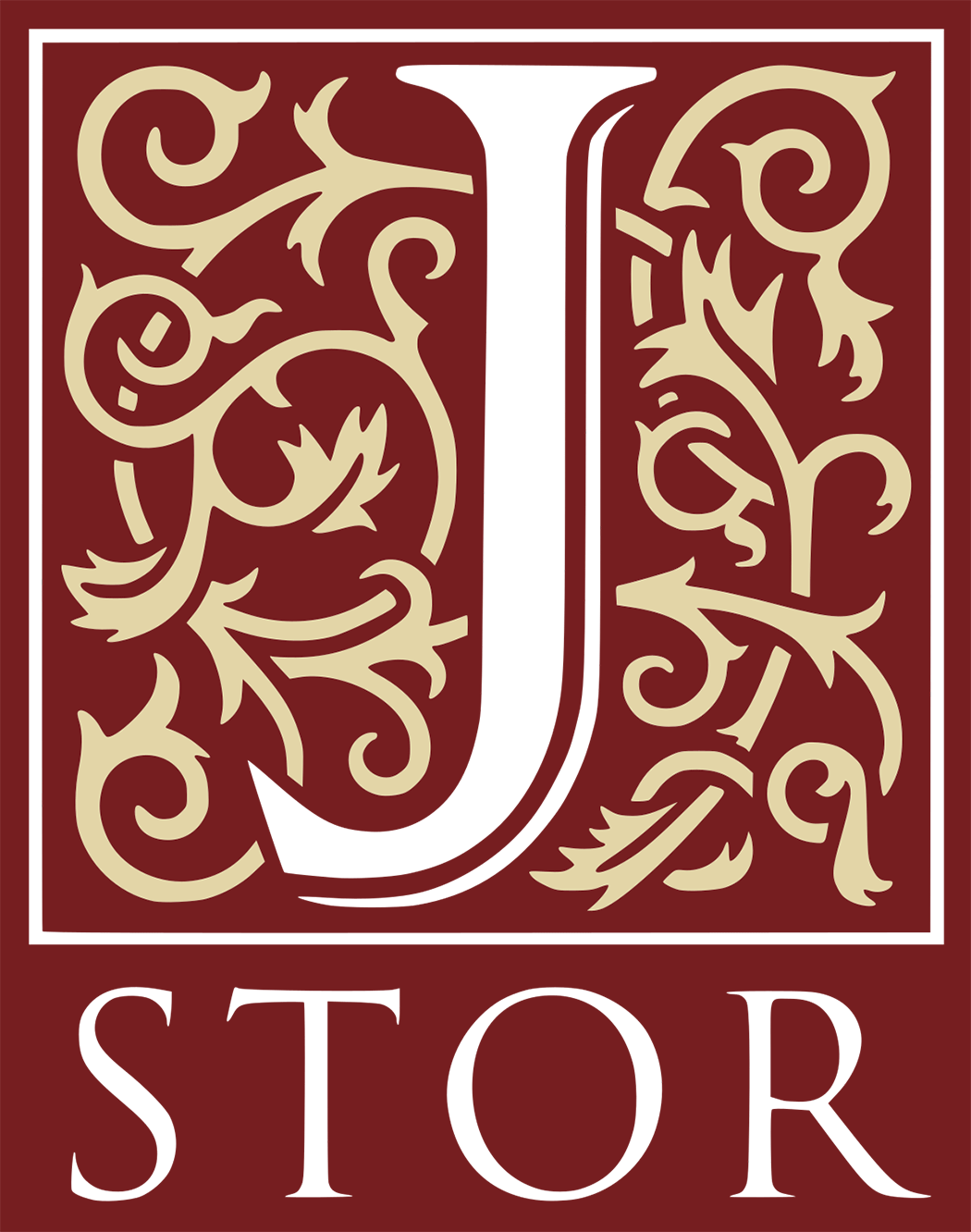


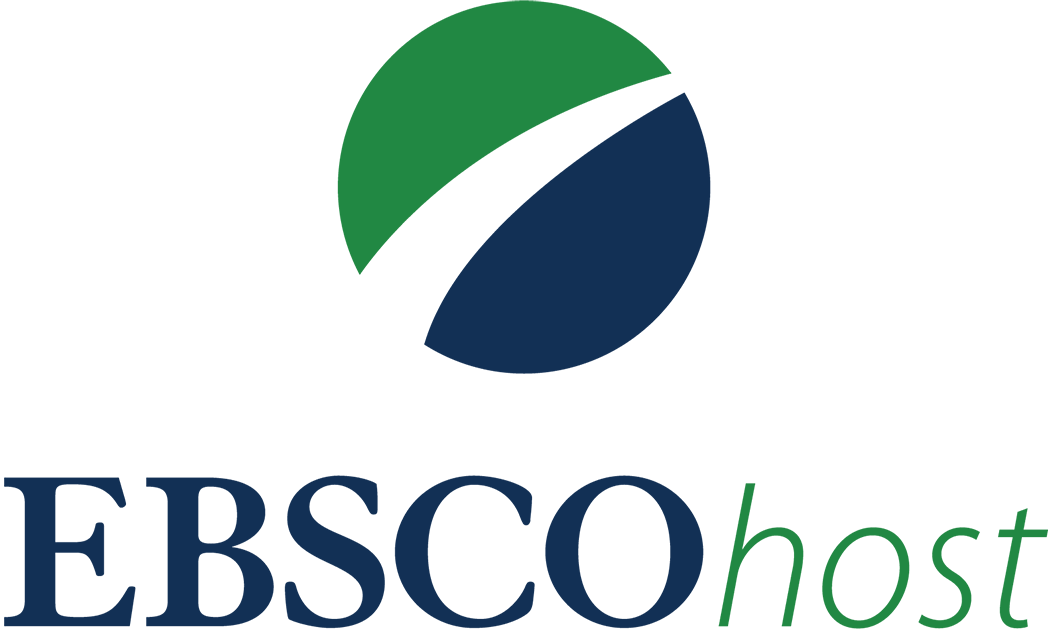

2014 Northeastern Naturalist Notes Vol. 21, No. 4
N56
J.D. Reichard, et al.
Interannual Survival of Myotis lucifugus (Chiroptera:
Vespertilionidae) near the Epicenter of White-Nose Syndrome
Jonathan D. Reichard1,*, Nathan W. Fuller2, Alyssa B. Bennett3, Scott R. Darling3,
Marianne S. Moore4, Kate E. Langwig5, Emily D. Preston6, Susi von Oettingen7,
Christopher S. Richardson8, and D. Scott Reynolds9
Abstract - Reduced populations of Myotis lucifugus (Little Brown Myotis) devastated by white-nose
syndrome (WNS) persist in eastern North America. Between 2009 and 2013, we recaptured 113
marked individuals that survived between 1 and 6 winters in New England since the arrival of WNS.
We also observed signs of reproductive success in 57 recaptured bats.
White-nose syndrome (WNS), caused by an introduced fungus, was first documented
in Schoharie County, NY, in 2006 and has since devastated populations of hibernating bats
in eastern North America (Turner et al. 2011). By early 2009, WNS had been documented
at most hibernacula throughout the Northeast, causing precipitous declines in wintering
populations of Myotis lucifugus (LeConte) (Little Brown Myotis) (Frick et al. 2010a, Langwig
et al. 2012). Acoustic monitoring indicated that activity of Little Brown Myotis on the
summer landscape also decreased over 70% after arrival of WNS (Brooks 2011, Dzal et
al. 2011, Ford et al. 2011). Accordingly, most summer colonies in the area also declined
dramatically in size (e.g., Frick et al. 2010b, Fuller et al. 2012). Still, small numbers of
Little Brown Myotis persist at summer roosts in states that have a high incidence of mortality
related to WNS. For example, intensive monitoring of a colony at Fort Drum Military
Installation in New York revealed that individual Little Brown Myotis survived multiple
years after arrival of WNS (Dobony et al. 2011). At least 65 bats, including reproductive
females and bats initially banded as juveniles survived for up to 4 years from 2009 to 2013
(C. Dobony, US Department of Army, Fort Drum, NY, pers. comm.). These observations
indicate that Little Brown Myotis is persisting and reproducing at this site in New York,
despite likely exposure each winter to the pathogen that causes WNS, Pseudogymnoascus
destructans (Gargas, Trest, Christensen, Volk, and Blehert) (Lorch et al. 2011, Minnis and
Lindner 2013).
The purpose of this note is to summarize additional scattered evidence of interannual
survival of Little Brown Myotis at multiple summer roosts in Massachusetts, New Hampshire,
and Vermont. In addition, we discuss anecdotal observations on reproductive success
in these colonies, which provide perspectives on untested hypotheses regarding the sublethal
effects of WNS. Understanding the status and location of persisting colonies of Little
Brown Myotis provides a foundation for more deliberate studies into long-term ecological
and behavioral effects of WNS on bats.
Between 2005 and 2012, the authors applied 2095 (MA:1179, NH:820, and VT:96)
2.9-mm, split-ring, alloy bands (Porzana, Inc., UK) to the forearms of Little Brown Myotis
1US Fish and Wildlife Service, Hadley, MA 01035. 2Boston University, Boston, MA 02215. 3Vermont
Fish and Wildlife Department, Rutland, VT 05701. 4State University of New York, Stony Brook,
NY 11794. 5University of California Santa Cruz, Santa Cruz, CA. 6New Hampshire Fish and Game
Department, Concord, NH 03301. 7US Fish and Wildlife Service, Concord, NH 03301. 8Northeastern
University, Boston, MA, 02115. 9St. Paul’s School, Concord, NH 03301. Corresponding author -
jonathan_reichard@fws.gov.
Manuscript Editor: Allen Kurta
Notes of the Northeastern Naturalist, Issue 21/4, 2014
N57
2014 Northeastern Naturalist Notes Vol. 21, No. 4
J.D. Reichard, et al.
captured during various research and management activities at 8 summer roosts (MA:3,
NH:3, and VT:2). All sites were within the putative migratory range of Little Brown Myotis
from WNS-infected hibernacula in New England (Davis and Hitchcock 1965). Because of
the nearly ubiquitous occurrence of P. destructans in affected areas (Lorch et al. 2013), we
assumed that any bats captured in this area in summer 2009 or later represented individuals
that hibernated in sites where P. destructans was likely present and have somehow remained
unexposed to, resisted, or recovered from WNS.
We captured 113 of the 2095 previously banded Little Brown Myotis in subsequent
summers between 2009 and 2013 (Table 1). Although our recapture rate is much lower than
recapture rates reported in other studies (Frick et al. 2010b, Keen and Hitchcock 1980),
we caution that the varied methods that produced these observations were not sufficient to
make inferences from these recapture rates. In total, 20 bats survived ≥4 years after being
banded. Two of these bats were captured on 3 June 2013 in Princeton, MA, 5 years after being
banded there, and two others were recaptured on 20 July 2012 in Cornwall, VT, 6 years
after being banded at that location. We recaptured bats in various stages of reproduction
(Table 2), thus documenting successful ovulation, fertilization, gestation, and parturition in
these colonies. Of the 113 recaptured bats, 15 were initially banded as young-of-the-year
and were recaptured up to 4 years later. Nine of these 15 bats exhibited signs of pregnancy
or lactation when recaptured, suggesting that they also produced offspring.
Although WNS predominantly affects bats during winter, factors associated with their
summer habitats and behavior may influence their response to the disease. Variation in
interannual survival and recruitment among maternity colonies may signify potential
behavioral, environmental, and/or inherited physiological factors relevant to surviving
Table 1. Number of recaptured Little Brown Myotis in Massachusetts, New Hampshire, and Vermont
during summer 2009–2013. Numbers in parentheses represent bats that were originally banded as
young-of-the-year. Unless noted, all bats reported were female.
Number of Summer in which the bat was last recovered
winters survived 2009 2010 2011 2012 2013 Total
1 34 (3) - - 21 (2) 7 (1) 62 (6)
2 - - 9* (2) - 6 (2) 15 (4)
3 - - 3** (3) 13 (1) - 16 (4)
4 2 - - 14 (1) - 16 (1)
5 - - - - 2 2
6 - - - 2 - 2
*Includes 1 adult male recaptured in Framingham, MA, on 12 July 2011.
**Includes 1 adult male recaptured in Milford, NH, on 17 July 2012.
Table 2. Reproductive conditions at time of recapture of Little Brown Myotis recaptured 1–6 years
after being banded in Massachusetts, New Hampshire, and Vermont during summer 2006–2013. Numbers
in parentheses represent bats that were originally banded as young-of-the-year.
Number of winters survived
Condition 1 2 3 4 5 6 Total
Pregnant 15 (2) 3 (3) 1 (1) 2 1 - 22 (6)
Lactating 9 (2) 1 5 5 1 - 21 (2)
Postlactating 3 - 2 7 (1) - 2 14 (1)
2014 Northeastern Naturalist Notes Vol. 21, No. 4
N58
J.D. Reichard, et al.
infection with WNS. Because female Little Brown Myotis exhibit high fidelity to natal
roosts (Dixon 2011, Humphrey and Cope 1976), maternally heritable factors (e.g., immunocompetence,
metabolism) that could contribute to a bat’s ability to survive WNS may be
traceable to certain summer roosts and have the potential to perpetuate in persisting populations.
Environmental and social variables during postnatal development can also influence
many aspects of the lives of neonates, such as their symbiotic microbial fauna (Altizer et al.
2003), migratory behavior (Fleming and Eby 2003), exposure to contaminants (Bayat et al.
2014, Yates et al. 2013), availability of prey (Clare et al. 2011), and exposure to pathogens,
potentially including P. destructans. Ecology and biology of WNS-affected bats during
summer may be an important factor influencing the impact of the disease, and this area of
study deserves continued attention.
The hibernacula used by the bats reported in this study are largely unknown, although
recapturing banded individuals at sites other than where they are banded is generally rare
(Griffin 1970). During our study, only 1 individual was banded at a maternity colony and
subsequently recaptured at a hibernaculum more than 1 year after initial capture. This bat,
a female, was banded in Milford, NH, in summer 2008, and was subsequently observed at
Eagle Cave in the central Adirondack Mountains, NY, in March 2009 and again in March 2013
(R. von Linden, New York Department of Environmental Conservation, Albany, NY, pers.
comm.). Notably, the same bat was also observed at the summer roost in Milford in 2012.
The data reported in this note represent opportunistic records of multi-year survival
at summer roosts and thus corroborate results from Fort Drum, NY, with data from additional
sites in Massachusetts, Vermont, and New Hampshire. Still, the current population
of Little Brown Myotis in the area most heavily affected by WNS appears strikingly low
compared to past censuses. With limited resources and dwindling populations of bats,
conservation agencies may need to consolidate research and management and focus on
protecting summer colonies with efforts to shield healthier bats from other potential
threats to their survival. Understanding the current distribution, behavior, ecology, and
genetics of surviving bats may be critical to conserving these populations and developing
effective recovery strategies.
Acknowledgments. We thank numerous landowners for access to roosts and their continued
stewardship of bat colonies. We appreciate contributions and early comments on
this note from R. von Linden, C. Dobony, and J. Coleman. Assistance in the field and with
data collection was provided by T. Murtha, L. Le, A. Greco, A. Banks, S. Fellows, A. Irwin,
M. Nabhan, S. Miller-Fellows, A. Luken, S. MacKay, A. Hunt, J. Collins, and C. Kocer.
The authors and field assistants were supported by funding from the US Fish and Wildlife
Service (E.D. Preston, J.D. Reichard, M.S. Moore), the National Science Foundation (K.E.
Langwig, M.S. Moore), Bat Conservation International (K.E. Langwig, M.S. Moore, N.W.
Fuller), Biodiversity Research Institute (M.S. Moore), and National Speleological Society
(M.S. Moore, N.W. Fuller). M.S. Moore is currently supported by the National Institute of
General Medical Science of the National Institutes of Health, Bethesda, MD, under award
number K12GM102778.
Literature Cited
Altizer, S., C.L. Nunn, P.H. Thrall, J.L. Gittleman, J. Antonovics, A.A. Cunningham, A.P. Dobson, V.
Ezenwa, K.E. Jones, A.B. Pedersen, M. Poss, and J.R.C. Pulliam. 2003. Social organization and
parasite risk in mammals: Integrating theory and empirical studies. Annual Reviews in Ecology,
Evolution, and Systematics 34:517–547.
Bayat, S., F. Geiser, P. Kristiansen, and S.C. Wilson. 2014. Organic contaminants in bats: Trends and
new issues. Environment International 63:40–52.
N59
2014 Northeastern Naturalist Notes Vol. 21, No. 4
J.D. Reichard, et al.
Brooks, R.T. 2011. Declines in summer bat activity in central New England 4 years following the
initial detection of white-nose syndrome. Biodiversity and Conservation 20:2537–2541.
Clare, E.L., B.R. Barber, B.W. Sweeney, P.D.N. Hebert, and M.B. Fenton. 2011. Eating local:
Influences of habitat on the diet of Little Brown Bats (Myotis lucifugus). Molecular Ecology
20:1772–1780.
Davis, W.H., and H.B. Hitchcock. 1965. Biology and migration of the bat, Myotis lucifugus, in New
England. Journal of Mammalogy 46:296–313.
Dixon, M.D. 2011. Population genetic structure and natal philopatry in the widespread American bat
Myotis lucifugus. Journal of Mammalogy 92:1343–1351.
Dobony, C.A., A.C. Hicks, K.E. Langwig, R.I. von Linden, J.C. Okoniewski, and R.E. Rainbolt.
2011. Little Brown Myotis persist despite exposure to white-nose syndrome. Journal of Fish and
Wildlife Management 2:190–195.
Dzal, Y., L.P. Mcguire, N. Veselka, M.B. Fenton. 2011. Going, going, gone: The impact of white-nose
syndrome on the summer activity of the Little Brown Bat (Myotis lucifugus). Biology Letters
7:392–394.
Fleming, T.H., and P. Eby. 2003. Ecology of bat migration. Pp. 156–208, In T.H. Kunz and M.B.
Fenton (Eds.). Bat Ecology. University of Chicago Press, Chicago, IL. 779 pp.
Ford, W.M., E.R. Britzke, C.A. Dobony, J.L. Rodrigue, and J.B. Johnson. 2011. Patterns of acoustical
activity of bats prior to and following white-nose syndrome occurrence. Journal of Fish and
Wildlife Management 2:125–134.
Frick, W.F., J.F. Pollock, A.C. Hicks, K.E. Langwig, D.S. Reynolds, G.G. Turner, C.M. Butchkoski,
and T.H. Kunz. 2010a. An emerging disease causes regional population collapse of a common
North American bat species. Science 329:679–682.
Frick, W.F., D.S. Reynolds, and T.H. Kunz. 2010b. Influence of climate and reproductive timing on
demography of Little Brown Myotis Myotis lucifugus. Journal of Animal Ecology 79:128–136.
Fuller, N.W., J.D. Reichard, M.L. Nabhan, S.R. Fellows, L.C. Pepin, and T.H. Kunz. 2011. Freeranging
Little Brown Myotis (Myotis lucifugus) heal from wing damage associated with whitenose
syndrome. EcoHealth 2:154–162.
Griffin, D.R. 1970. Migration and homing of bats. Pp. 233–264, In W.A. Wimsatt (Ed.). Biology of
Bats. Vol. 1. Academic Press, NY. 406 pp.
Humphrey, S.R., and J.B. Cope. 1976. Population ecology of the Little Brown Bat, Myotis lucifugus,
in Indiana and north-central Kentucky. Special Publication of the American Society of Mammalogists
4:1–81.
Keen, R., and H.B. Hitchcock. 1980. Survival and longevity of the Little Brown Bat (Myotis lucifugus)
in southeastern Ontario. Journal of Mammalogy 61:1–7.
Langwig, K.E., W.F. Frick, J.T. Bried, A.C. Hicks, T.H. Kunz, and A.M. Kilpatrick. 2012. Sociality,
density-dependence, and microclimates determine the persistence of populations suffering from a
novel fungal disease, white-nose syndrome. Ecology Letters 15:1050–1057.
Lorch, J.M., C.U. Meteyer, M.J. Behr, J.G. Boyles, P.M. Cryan, A.C. Hicks, A.E. Ballmann, J.T.H.
Coleman, D.N. Redell, D.M. Reeder, and D.S. Blehert. 2011. Experimental infection of bats with
Geomyces destructans causes white-nose syndrome. Nature 480:376–379.
Lorch, J.M., L.K. Muller, R.E. Russell, M. O’Connor, D.L. Lindner, and D.S. Blehert. 2013. Distribution
and environmental persistence of the causative agent of white-nose syndrome, Geomyces
destructans, in bat hibernacula of the eastern United States. Applied and Environmental Microbiology
79:1293–1301.
Minnis, A.M., and D.L. Lindner. 2013. Phylogenetic evaluation of Geomyces and allies reveals no
close relatives of Pseudogymnoascus destructans, comb. nov., in bat hibernacula of eastern North
America. Fungal Biology 117:638–649.
Turner, G.G., D.M. Reeder and J.T.H. Coleman. 2011. A five-year assessment of mortality and
geographic spread of white-nose syndrome in North American bats and a lok to the future. Bat
Research News 52:13–27.
Yates, D.E., E.M. Adams, S.E. Angelo, D.C. Evers, J. Schmerfeld, M.S. Moore, T.H. Kunz, T. Divoll,
S.T. Edmonds, C. Perkins, R. Taylor, and N.J. O’Driscoll. 2013. Mercury in bats from the northeastern
United States. Ecotoxicology 23:45–55.