Evaluation of Ailanthus altissima as a Bioindicator to Detect
Phytotoxic Levels of Ozone
Lauren K. Seiler, Dennis R. Decoteau, and Donald D. Davis
Northeastern Naturalist, Volume 21, Issue 4 (2014): 541–553
Full-text pdf (Accessible only to subscribers. To subscribe click here.)
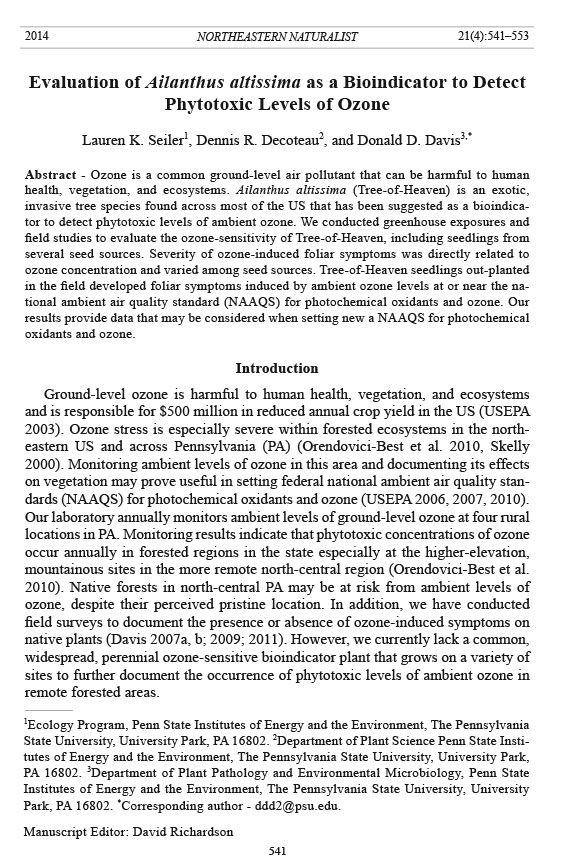
Access Journal Content
Open access browsing of table of contents and abstract pages. Full text pdfs available for download for subscribers.
Current Issue: Vol. 30 (3)
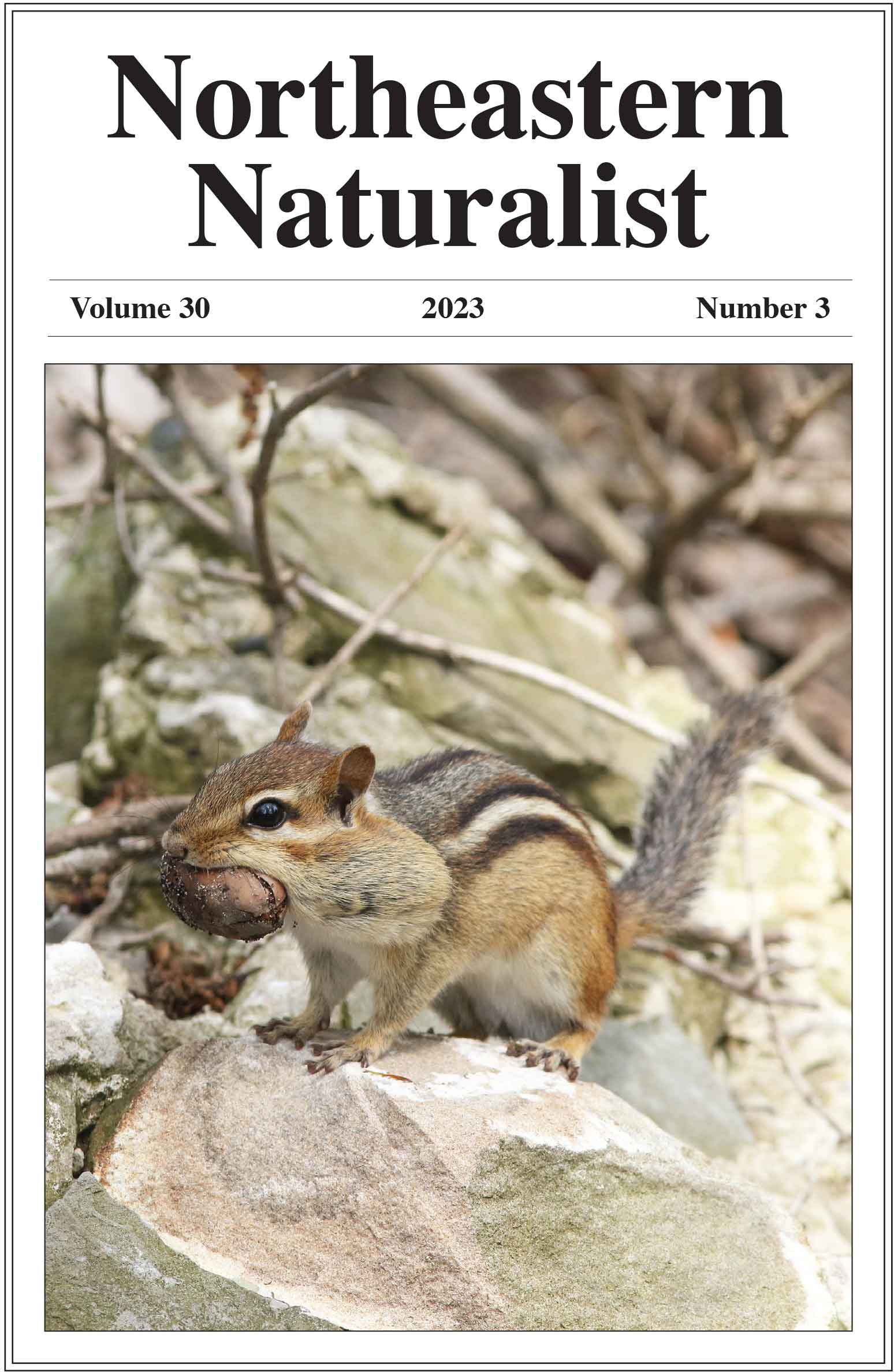
Check out NENA's latest Monograph:
Monograph 22
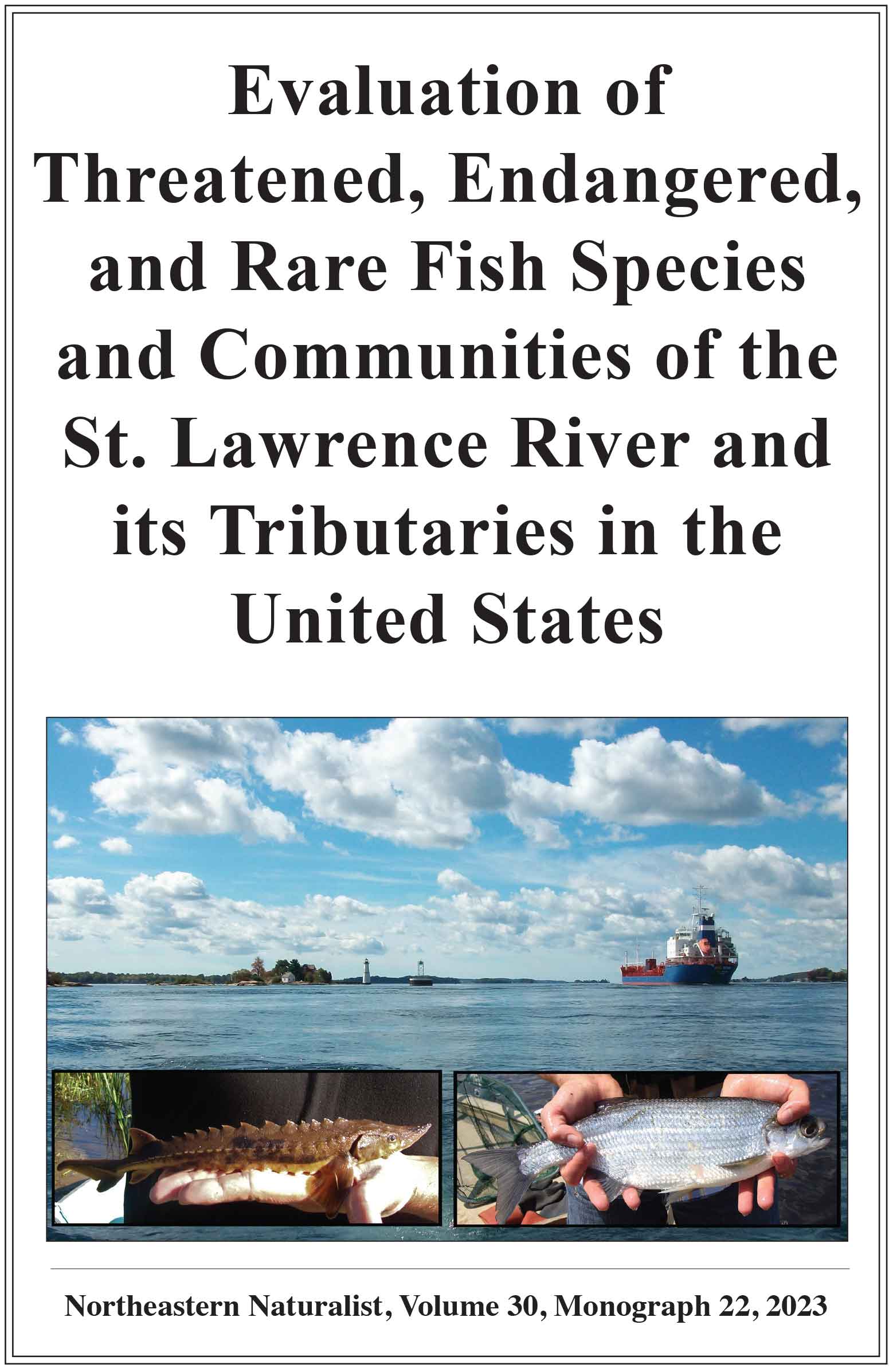
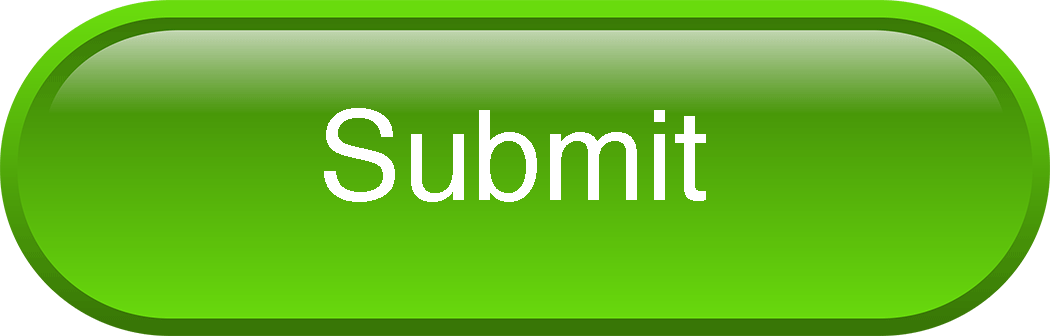
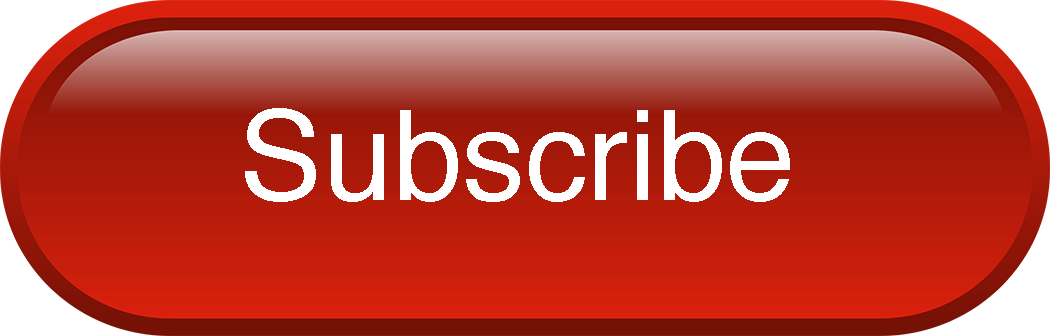
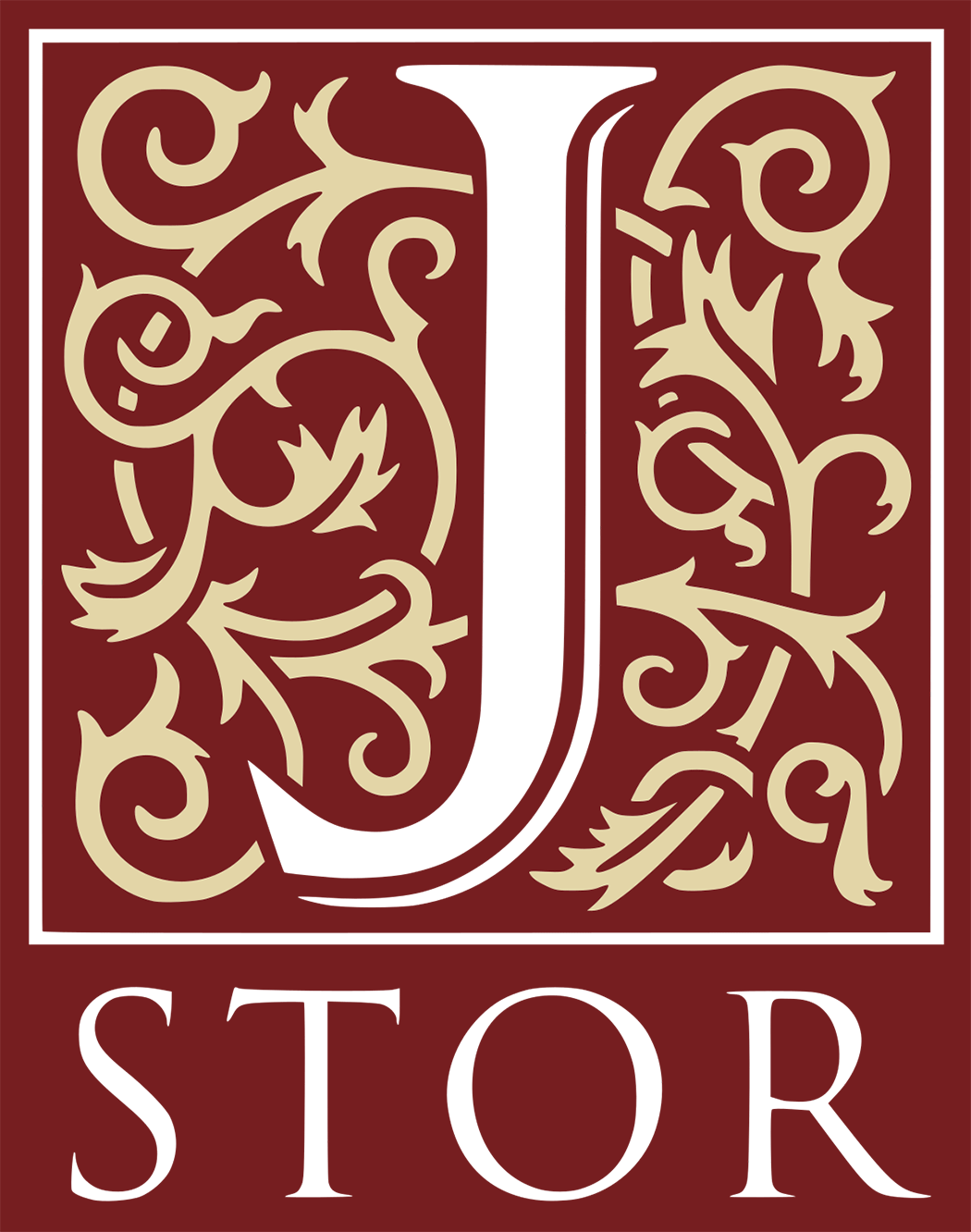


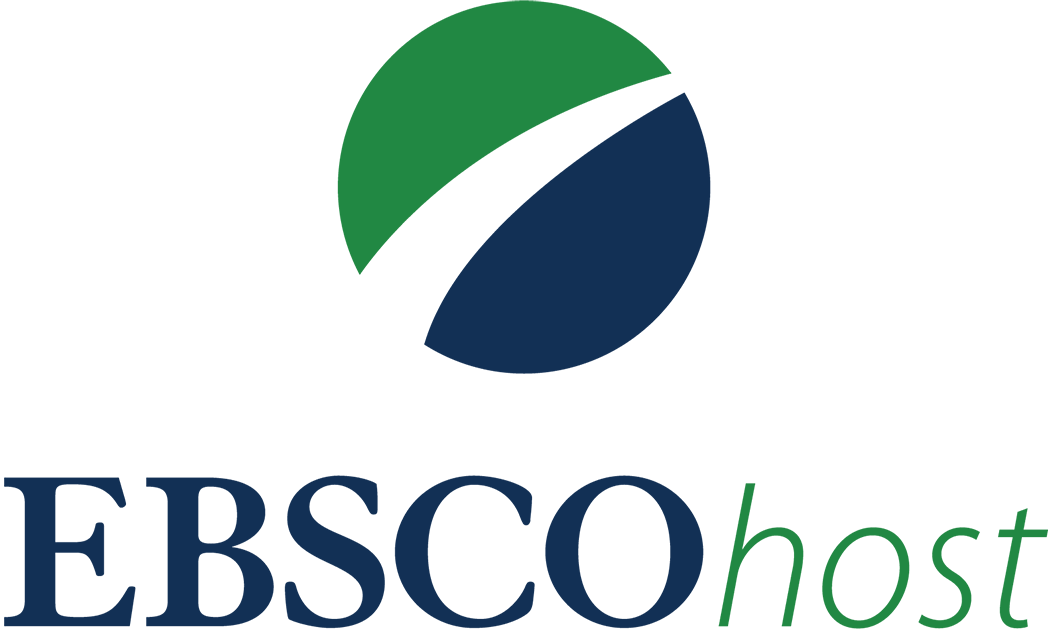

Northeastern Naturalist Vol. 21, No. 4
L.K. Seiler, D.R. Decoteau, and D.D. Davis
2014
541
2014 NORTHEASTERN NATURALIST 21(4):541–553
Evaluation of Ailanthus altissima as a Bioindicator to Detect
Phytotoxic Levels of Ozone
Lauren K. Seiler1, Dennis R. Decoteau2, and Donald D. Davis3,*
Abstract - Ozone is a common ground-level air pollutant that can be harmful to human
health, vegetation, and ecosystems. Ailanthus altissima (Tree-of-Heaven) is an exotic,
invasive tree species found across most of the US that has been suggested as a bioindicator
to detect phytotoxic levels of ambient ozone. We conducted greenhouse exposures and
field studies to evaluate the ozone-sensitivity of Tree-of-Heaven, including seedlings from
several seed sources. Severity of ozone-induced foliar symptoms was directly related to
ozone concentration and varied among seed sources. Tree-of-Heaven seedlings out-planted
in the field developed foliar symptoms induced by ambient ozone levels at or near the national
ambient air quality standard (NAAQS) for photochemical oxidants and ozone. Our
results provide data that may be considered when setting new a NAAQS for photochemical
oxidants and ozone.
Introduction
Ground-level ozone is harmful to human health, vegetation, and ecosystems
and is responsible for $500 million in reduced annual crop yield in the US (USEPA
2003). Ozone stress is especially severe within forested ecosystems in the northeastern
US and across Pennsylvania (PA) (Orendovici-Best et al. 2010, Skelly
2000). Monitoring ambient levels of ozone in this area and documenting its effects
on vegetation may prove useful in setting federal national ambient air quality standards
(NAAQS) for photochemical oxidants and ozone (USEPA 2006, 2007, 2010).
Our laboratory annually monitors ambient levels of ground-level ozone at four rural
locations in PA. Monitoring results indicate that phytotoxic concentrations of ozone
occur annually in forested regions in the state especially at the higher-elevation,
mountainous sites in the more remote north-central region (Orendovici-Best et al.
2010). Native forests in north-central PA may be at risk from ambient levels of
ozone, despite their perceived pristine location. In addition, we have conducted
field surveys to document the presence or absence of ozone-induced symptoms on
native plants (Davis 2007a, b; 2009; 2011). However, we currently lack a common,
widespread, perennial ozone-sensitive bioindicator plant that grows on a variety of
sites to further document the occurrence of phytotoxic levels of ambient ozone in
remote forested areas.
1Ecology Program, Penn State Institutes of Energy and the Environment, The Pennsylvania
State University, University Park, PA 16802. 2Department of Plant Science Penn State Institutes
of Energy and the Environment, The Pennsylvania State University, University Park,
PA 16802. 3Department of Plant Pathology and Environmental Microbiology, Penn State
Institutes of Energy and the Environment, The Pennsylvania State University, University
Park, PA 16802. *Corresponding author - ddd2@psu.edu.
Manuscript Editor: David Richardson
Northeastern Naturalist
542
L.K. Seiler, D.R. Decoteau, and D.D. Davis
2014 Vol. 21, No. 4
Manning and Federer (1980) advocated the use of bioindicators to yield a
general indication of presence or absence of phytotoxic levels of ambient ozone.
Bioindicators offer an alternative to the use of chemical/mechanical air monitors
that directly measure ambient pollutant levels. Although plant bioindicators do not
yield quantitative air-quality data for specific pollutants, they have advantages over
mechanical/chemical means, including lower costs, less maintenance, and greater
flexibility with respect to expansion or relocation. In addition, because they are part
of the ecosystem, bioindicators are likely to be more directly correlated with the
health of the ecosystem than are human-made monitors.
Since the discovery that leaf stipple on Vitis sp. (grape) was caused by ozone
(Richards et al. 1958), adaxial dark stipple has been the classic foliar symptom used
to evaluate levels of ozone-induced symptoms on broadleaved bioindicators (Skelly
2000, Skelly et al. 1987). The presence of stippling has been utilized as the standard
broadleaf-plant response to ozone in many laboratory, greenhouse, and field studies
(Skelly 2000). In 1974, we reported that Tree-of-Heaven was sensitive to ozone
within a controlled environment chamber in our laboratory, with 74% of 35 exposed
seedlings developing dark stipples on the adaxial leaf surface, as well as adaxial
tan stipples, chlorosis, and premature defoliation (Davis and Coppolino 1974). In
addition to documented effects on seedlings, injury due to ambient ozone has been
observed on Tree-of-Heaven saplings and trees watered only by natural rainfall in
various areas in PA (D.D. Davis, pers. observ), as well as within a wildlife refuge
in New Jersey (Davis and Orendovici 2006). The predominant ozone-induced foliar
symptom on Tree-of-Heaven in these surveys was a dark adaxial stipple, but
the species also occasionally exhibited only adaxial tan stipple. Based on these
observations, we proposed that Tree-of-Heaven might be a useful ozone-sensitive
bioindicator (USDOI 2003). Likewise, Gravano et al. (2003) reported that potted,
watered Tree-of-Heaven root suckers were useful bioindicators in Italy, where
ambient ozone induced a dark adaxial leaf stipple. However, to the best of our
knowledge, no studies have been conducted under a combination of carefully controlled
exposures and field observations that included rigorous statistical analyses.
The main objective of this study was to more thoroughly evaluate the usefulness of
Tree-of-Heaven as an ozone-sensitive bioindicator.
Methods
We used two experimental approaches to evaluate the efficacy of Tree-of-Heaven
as a bioindicator of ozone pollution. First, potted Tree-of-Heaven seedlings were
exposed to ozone within continuously stirred tank reactor (CSTR) chambers (Heck
et al. 1975) in a greenhouse during 2010 and 2011 to establish the relationship
between controlled ozone-exposure concentrations and induced foliar stipple and
to determine if response to ozone varied among seedlings originating from several
seed sources across the country. Second, we planted Tree-of-Heaven seedlings in a
central PA field in 2011 to observe the species’ response to ambient ozone, as measured
at two nearby Environmental Protection Agency (EPA) monitoring sites.
Northeastern Naturalist Vol. 21, No. 4
L.K. Seiler, D.R. Decoteau, and D.D. Davis
2014
543
2010 greenhouse exposures
As part of a related study (Kasson et al. 2013), we acquired Tree-of-Heaven seed
from six locations across the US: Bloomington, IN; Corvallis, OR; Far Rockaway,
NY; Mineral, VA; Reno, NV; and State College, PA (M.T. Kasson, Penn State, State
College, PA, pers. comm.). We placed one hundred Tree-of-Heaven seeds from
each source in a refrigerator maintained at approximately 2 °C. Two weeks later, we
placed the seeds on flats containing a general potting mix, moved them to a greenhouse,
and watered all flats. The greenhouse, located on The Pennsylvania State
University campus, had been modified to pass incoming air through activated-charcoal
filters, which reduced the ambient ozone concentration within the greenhouse to
less than 8 ppb ozone. Upon germination, we planted 64 Tree-of-Heaven seedlings from each
seed source in the greenhouse in individual 2-L pots containing SUN GRO Metro-
Mix 200 growing medium (Sun Gro Horticulture Canada Ltd., Bellevue, WA) and
fertilized them with ~5g Osmocote Plus® (15N:9P:12K) fertilizer (The Scotts Company
LLC, Marysville, OH). We randomly arranged potted seedlings on greenhouse
benches, where they received charcoal-filtered air, and watered them as needed. Prior
to ozone exposure, four seedlings from each seed source were randomly assigned to
one of the 16 CSTR chambers and to a specific location within the chamber.
We initiated ozone exposure on 9 August 2010. Although all Tree-of-Heaven
seedlings were of similar age (~6–7 weeks), height varied among seedlings originating
from the various seed sources. Mean initial height (cm) of seedlings from
each seed source was 22.2 (NY), 18.3 (NV), 17.9 (VA), 17.6 (PA), 13.9 (IN), and
12.1 (OR). Potted Tree-of-Heaven seedlings were exposed to 75 or 120 ppb ozone
in a square-wave exposure for 8 hr/day, 5 days/week (Monday–Friday) for 3 weeks.
Daily exposures began at 0900 hr and ended at 1700 hr. Seven of the 16 CSTR
chambers were maintained at a target concentration of 75 ppb ozone, which was
near the NAAQS for ozone (USEPA 2006), and seven chambers were set at a target
of 120 ppb, a higher level intended to induce foliar symptoms in the event that
conditions were not conducive for symptom development. Two control chambers
received the ambient greenhouse charcoal-filtered air (less than 8 ppb ozone daily hourly
average). We monitored ozone concentrations (ppb) in each chamber for 1.5 min
at 12-min intervals during each exposure and sampled ozone through Teflon tubing
using a solenoid-driven sampling system connected to a calibrated TECO Model 49
photometric ozone analyzer (Thermo Environmental Corp., Franklin, MA). Ozone
and environmental data were input to a data-logger connected to a PC computer.
During non-exposure hours, all plants remained in the CSTR chambers with the
chamber doors open and were exposed to internal greenhouse environmental conditions,
including charcoal-filtered air. Mean relative humidity was ~72% and mean
temperature was ~29 oC in the chambers. We performed routine quality-control
measures on monitoring equipment throughout the study.
We visually rated Tree-of-Heaven leaves for ozone-induced symptoms at the
end of each exposure week (on Sunday), using a modified Horsfall-Barratt classification
scale (Horsfall and Barratt 1945). We rated each plant from 0–5 for amount
(AMT) and severity (SEV) of ozone-induced foliar symptoms. The rating values
Northeastern Naturalist
544
L.K. Seiler, D.R. Decoteau, and D.D. Davis
2014 Vol. 21, No. 4
corresponded to the following percentages: 0 = 0%, 1 = 1–6%, 2 = 7–25%, 3 = 26–
50%, 4 = 51–75%, and 5 = 76–100%. AMT described percentage of symptomatic
leaves on a plant, whereas SEV described percentage of adaxial leaf surface that
was symptomatic on affected leaves. We also made subjective observations, such as
color of symptoms, but we did not include them in our statistical analyses. We converted
AMT and SEV ratings for each plant to percentage values representing the
midpoint of each injury class as follows: 0 = 0%, 1 = 3.5%, 2 = 16%, 3 = 38%, 4 =
63%, and 5 = 88%. We used AMT*SEV to represent whole plant injury, INJP. Mean
INJP values for seedlings from each seed source, INJSS, were calculated and transformed
using a log10 data transformation to attain equal variances. We employed a
2-way ANOVA to test for significant differences (P = 0.05) among transformed final
(week 3) injury values for each seed source per treatment, and a Tukey HSD test to
identify the significant differences (P = 0.05) (SAS 2011).
2011 greenhouse exposures
In 2011, we followed the same protocol as in 2010. However, initial results of
the 2010 study prompted several improvements in the 2011 methodology. In 2010,
the potted Tree-of-Heaven seedlings from the OR seed source were more sensitive
to ozone than seedlings from other seed sources. Therefore, to increase the possibility
that the ozone would induce visible symptoms, we exposed only seedlings from
the OR seed source in 2011. In addition, at the conclusion of the 2010 study, some
CSTR chambers (e.g., on the west side of the greenhouse) appeared to be slightly
warmer than others. Therefore, we monitored the pre-exposure temperature in all
16 chambers for 24 hr/day for 10 days prior to start of the 2011 exposures. We
used the overall 10-day mean temperature for each chamber to group the exposure
chambers into three blocks (five chambers/block) designated as low (mean = 24.6
ºC), medium (mean = 25.2 ºC), and high (mean = 25.8 ºC) temperature regimes.
The 2010 results also suggested that measuring more ozone levels would allow
better delineation of the relationship between ozone concentration and plant injury.
Therefore, we used five target ozone concentrations (40, 60, 80, 100, and 120 ppb)
in the CSTR chambers during 2011. Within each temperature block, we randomly
assigned each individual chamber to one of the five target ozone-concentrations.
One chamber served as the ozone control (less than 8 ppb ozone). We initiated ozone exposures
at 0900 h on 11 July 2011, at which time seedlings were ~9 weeks old, and
conducted the treatments 8 hrs/day, 5 d/wk (Monday–Friday) for 4 weeks. During
exposures, mean relative humidity was ~80% and mean temperature was 25–26
ºC, as described above. Each Sunday, we rated the incidence and severity of ozone
injury on each plant, as described for the 2010 studies. We converted ozone-induced
foliar-injury ratings into percentages, and calculated AMT * SEV for each plant to
represent whole-plant injury (INJP). A multiple regression analysis was performed
using the mean final (week 4) INJP values, mean ozone concentration, mean initial
plant height (cm), and mean temperature (ºC) for each chamber. Two interaction
terms were used in the regression analysis: temperature x ozone (teoz) and height x
ozone (heoz). We used SAS statistical software (SAS 2011) to analyze our data.
Northeastern Naturalist Vol. 21, No. 4
L.K. Seiler, D.R. Decoteau, and D.D. Davis
2014
545
2011 field studies
We germinated and grew Tree-of-Heaven seeds from one seed source (OR) in
the greenhouse as in 2010. On 13 June 2011, we planted the thirty-six resultant
seedlings (mean height = 24 cm) in a mowed field at Penn State’s Russell E. Larson
Agricultural Research Center, Rock Springs, PA, located ~16 km west of the CSTR
greenhouse facility. During dry weather, we watered seedlings as needed. We made
weekly AMT and SEV ratings for foliar injury induced by ambient ozone on the
Tree-of-Heaven seedlings for 9 weeks (18 July–13 September 2011), converted the
ozone-induced foliar injury ratings into percentages, and calculated AMT*SEV for
each plant to represent whole-plant injury (INJP).
We downloaded hourly ambient ozone concentrations for the 2011 growing season
from EPA AIRS site #42-027-9991, located ~1.1 km northeast of the field site.
We calculated two indices, SUM60 and W126, from the downloaded ozone datasets
and selected them as metrics of ambient ozone levels to determine if ambient ozone
levels were related to the level of ozone injury on the bioindicator plants. SUM60 is
the sum of mean hourly ozone averages ≥60 ppb, expressed in ppb.hrs. We selected
this index because ambient SUM60 ozone has been correlated with ozone injury
within eastern US forests (Hildebrand et al. 1996). We calculated the W126 index
by multiplying hourly ozone averages between 0800 and 2000 hrs as a sigmoidal
weighted function 1/(1 + 4403 * e[-126 * C]), where C is the hourly ozone concentration
in ppb, which is added cumulatively over the growing season. The sum of these values
(expressed in ppb.hrs) during the greatest consecutive 3-month period averaged
over 3 years is calculated to determine the W126 ozone index. We selected the W126
index because the EPA has considered this index in calculations of a national secondary
NAAQS for ambient ground-level ozone (USEPA 2007, 2010).
Results
2010 greenhouse exposures
The two-way ANOVA indicated that ozone concentration and seed source had
significant (P < 0.0001) effects on plant injury in 2010. Tukey’s test (P = 0.05)
results revealed that seedlings from the OR seed source exhibited significantly
greater INJss values than seedlings from the other sources. Injury values for the
other sources were statistically similar, with the exception of seedlings from the VA
source, which exhibited significantly greater ozone injury than those from the NY
seed source (Table 1). Seedlings from the NY source had the lowest INJss values of
all seed sources, but injury values were statistically different only from the OR and
VA seed sources. Control plants remained asymptomatic throughout the study.
In both the 75 and 120 ppb treatments during 2010, seedlings from all seed
sources except NY exhibited ozone injury after only 1 week of exposure to ozone
(Fig. 1). At 75 ppb ozone, mean INJss was directly related to exposure duration for
all six seed sources, but only seedlings from the OR seed source exhibited INJss
values that were significantly different from the other seed sources. At 120 ppb
ozone, mean INJss values were greater than those at 75 ppb. Injury levels increased
with exposure duration at 120 ppb, except for seedlings from the VA seed source,
Northeastern Naturalist
546
L.K. Seiler, D.R. Decoteau, and D.D. Davis
2014 Vol. 21, No. 4
which exhibited significantly greater INJss values at week 2, as compared to all seed
sources except OR. Over the duration of both exposure concentrations, seedlings
from the OR seed source exhibited the greatest INJss values, and seedlings from the
NY source displayed the lowest values (Table 1, Fig. 1).
2011 greenhouse exposures
Tree-of-Heaven seedlings in the control chamber, as well as those exposed to 40
ppb ozone, did not exhibit symptoms during the 2011 studies (data not shown). At
60–120 ppb ozone, the level of foliar injury was directly related to concentration
and duration of ozone exposure (Fig. 2). Seedlings exposed to 60 ppb ozone did
not exhibit foliar injury symptoms during weeks 1–2, but one of 12 plants (8.3%)
exhibited foliar injury following weeks 3 and 4. Seedlings in the 80 ppb ozone treatment
exhibited slight symptoms during weeks 1–3 of exposure, and greater injury
at week 4. Plants in the 100 ppb ozone treatment exhibited levels of foliar-injury
symptoms similar to those in the 80 ppb treatment during the first week of exposure,
and symptoms then increased until week 3, at which time they leveled off. The temporal
pattern of symptoms in the 120 ppb treatment was generally similar to those
in the 100 ppb treatment, but more severe.
Results of multiple regression analysis revealed the following significant (P =
0.0006, R2 = 0.8543) relationship between independent variables and final injury:
(INJP = -34992 + [424 * temp] + [705 * ozone] + [76 * height] - [8 * teoz] - [2 *
heoz]). The influence of each variable was significant in the regression equation.
Ozone concentration had the greatest influence on INJP, followed by teoz, heoz,
temperature, and height, respectively.
2011 field studies
Tree-of-Heaven seedlings planted in the field displayed ozone-induced foliar
injury symptoms when exposed to ambient ozone concentrations (Fig. 3). Seedlings
displayed black stipple in the beginning of the monitoring period, but black stipple
transitioned to tan stipple on 31 of 36 plants (86%) by the conclusion of the field
survey. Tree-of-Heaven first exhibited ozone injury on 8 August. The level of injury
increased in a non-linear fashion for the duration of the survey, except during 15–22
August, when ratings decreased. On the last day of evaluation, the final mean plantinjury
value (INJP) was 177.5.
Table 1. Seed sources and number of Tree-of-Heaven seedlings exposed to 75 and 120 ppb ozone in
2010. Mean final INJSS ± SE values represent average ozone-induced foliar injury per seed source for
all ozone treatments at the end of the exposure period (week 3). Means followed by the same letter
are not significantly different (P = 0.05) according to Tukey’s test.
Seed source Number of plants Mean final INJSSA ± SE
Corvallis, OR 64 1674.68 ± 215.61 c
Mineral, VA 64 437.92 ± 88.44 b
Reno, NV 64 359.15 ± 73.20 ab
Bloomington, IN 64 311.08 ± 84.32 ab
State College, PA 63 298.51 ± 58.44 ab
Far Rockaway, NY 61 128.80 ± 35.78 a
Northeastern Naturalist Vol. 21, No. 4
L.K. Seiler, D.R. Decoteau, and D.D. Davis
2014
547
Ambient levels of ozone first increased to 60 ppb (the first value to be included
in SUM60 considerations) on 11 May (Fig. 3). SUM60 values continued to increase
via a series of high ozone events over the course of the field study. The
highest ozone-concentration events occurred during 8–10 June and 17–23 July.
Figure 1. Mean foliar injury (INJSS ± SE) on Tree-of-Heaven seedlings from six seed sources
following exposure to 75 ppb (upper graph) or 120 ppb (lower graph) ozone for 1, 2, or 3
weeks in 2010. Ozone-induced foliar injury was not observed on seedlings in the control
(less than 8 ppb ozone).
Northeastern Naturalist
548
L.K. Seiler, D.R. Decoteau, and D.D. Davis
2014 Vol. 21, No. 4
Figure 2. Mean foliar injury (INJP ± SE) on Tree-of-Heaven seedlings from the OR seed
source exposed to 60, 80, 100, or 120 ppb ozone after 1, 2, 3, or 4 weeks in 2011. Ozoneinduced
foliar injury was not observed on seedlings in the control (less than 8 ppb ozone) or 40 ppb
ozone treatment.
Figure 3. Cumulative ozone concentrations of 60 ppb or greater for the 2011 growing
season (SUM60), cumulative weighted hourly ozone concentrations from 0800–2000 hrs
for the 2011 growing season (W126), and mean plant injury values (INJP) for out-planted
Tree-of-Heaven seedlings from the OR seed source per sampling date in survey. Error bars
represent ± SE.
Northeastern Naturalist Vol. 21, No. 4
L.K. Seiler, D.R. Decoteau, and D.D. Davis
2014
549
There were also periods of lower ozone-concentration events from 1–17 August and
3–13 September. However, ozone-induced foliar injury increased in an accumulative
pattern even during periods of (relatively) low ambient ozone concentration.
Ozone-induced foliar injury was observed on most Tree-of-Heaven seedlings when
the SUM60 reached approximately 14,000 ppb.hrs on about 8 August. SUM60 at
the conclusion of the survey was 15,232 ppb.hrs.
The cumulative sum of weighted hourly concentrations from 800–2000 hr daily
(W126 index) increased more steadily over the course of the growing season than
the SUM60 index (Fig. 3). Patterns of high- and low-concentration ozone events
indicated by the SUM60 index also occurred in the W126 index values. The final
W126 ozone index value for central PA in 2011 was 11,600 ppb.hrs.
Discussion
Our results suggest a range in ozone susceptibility among Tree-of-Heaven
populations. Tree-of-Heaven seedlings from the OR seed source were significantly
more sensitive to ozone than seedlings from the other seed sources (Table 1, Fig. 1).
This finding might have been related to unknown environmental factors that govern
ozone sensitivity (Bennett et al. 2006, Hildebrand et al. 1996), but also might have
been related to the evolution of tolerance, or lack thereof, to ambient ozone among
the various plant populations (Berrang et al. 1989, 1991). However, long-term ambient
ozone-monitoring data is not available to test the latter hypothesis.
The OR seedlings were smaller compared to seedlings from other seed sources.
Ozone susceptibility in plants is governed by a complex set of factors, ranging from
stomatal uptake/flux at the whole-plant level (Orendovici et al. 2007) to activity/efficiency
of antioxidants at the cell-membrane level (Krupa 1997, Krupa et al. 2001).
Small plants, such as the OR seedlings, may have fewer or less efficient antioxidants,
making them less able to repair ozone injury as compared to larger, healthier
plants (Krupa et al. 2001). Similarly, Tree-of-Heaven seedlings were smaller (mean
initial height = 12.1 cm) at the start of the 2010 ozone exposure, than those in the
2011 exposure experiment (mean initial height = 23.4 cm). After 3 weeks of exposure
to 120 ppb ozone in 2010, the initially smaller Tree-of-Heaven seedlings had
a mean INJP of 2705 compared to a mean of 627 for the larger seedlings used in
2011. This result might be related to plant size, but it is confounded because the
2010 exposure also occurred later in the growing season when plants are generally
more sensitive to ozone. In addition, differences in unknown environmental factors
between 2010 and 2011 may have influenced the different results from the 2 years.
Tree-of-Heaven seedlings exposed to ozone in the greenhouse chambers displayed
greater foliar injury with increasing ozone concentration, except seedlings
exposed to 40 ppb ozone, which did not differ from the control. The regression
model from the greenhouse studies indicated a positive linear relationship between
foliar injury and ozone concentration, suggesting that ozone-induced foliar
injury is indicative of the relative concentration of ozone in the area, which suggests
that a bioindicator may be useful as a biomonitor for ozone damage (Manning
and Federer 1980).
Northeastern Naturalist
550
L.K. Seiler, D.R. Decoteau, and D.D. Davis
2014 Vol. 21, No. 4
Foliar ozone injury exhibited a significantly negative linear relationship with
exposure temperature. Davis and Wood (1973) reported a similar strong negative
linear relationship between exposure temperature and ozone-induced plant injury.
However, both studies were conduced in chambers utilizing watered potted seedlings,
which may not reflect the complicated environmental relationships found
in nature. That is, ozone enters the plant during gas uptake through leaf stomata
(USEPA 2006), which usually close during times of moisture stress (Reynolds-
Henne 2010). In the field, moisture stress is induced by low rainfall and high ambient
temperatures, resulting in stomatal closure and reduced ozone uptake (Grulke et
al. 2003, Matyssek et al. 2006, Panek 2004), even in the presence of high ambient
ozone levels (Davis and Oredovici 2006, Showman 1991, Yuska et al. 2003). Therefore,
the regression equation derived from chamber data may have limited utility in
predicting outcomes for field-grown plants.
In the field, Tree-of-Heaven seedlings displayed ozone-induced black stipple
early in the monitoring period, but displayed a tan stipple later in the survey
period. In contrast, seedlings exposed to ozone within CSTR chambers under
greenhouse conditions displayed only tan stipple. The two ozone-induced responses
have been observed previously on Tree-of-Heaven in both the laboratory
(Davis and Coppolino 1974) and field setting (Davis and Orendovici 2006). Treeof-
Heaven seedlings, saplings, and trees exposed to ambient ozone in the field
most commonly exhibit the classic black stipple, which may occasionally change
to tan by late summer (D.D. Davis, pers. observ.). In contrast, the potted Tree-of-
Heaven seedlings exposed to ozone in CSTR chambers in this study displayed
only tan stipple. The mechanism for induction of two different symptom types on
Tree-of-Heaven is unknown, but may be influenced by ozone dose, leaf maturity,
or environmental factors, such as light intensity. Whatever the reason, when using
Tree-of-Heaven as an ozone bioindicator, field evaluations must recognize that
ozone can induce both symptom types.
The EPA current secondary standard for ozone pollution, which was developed
to protect crops and vegetation against injury, states that the 3-year mean of the
4th-highest daily maximum 8-hr average ozone concentrations measured at each
monitor within an area should not exceed 75 ppb. Ambient ozone was monitored at
two locations near our field-study site. The ozone monitor located ~1.1 km from our
study site revealed a 3-year mean of the 4th-highest daily maximum 8-hr-average
ozone concentrations of 71.5 ppb for 2009–2011, which is less than EPA’s NAAQS
for ozone pollution. This result suggests that the current NAAQS for ozone may not
be sufficient to protect sensitive vegetation from ozone-induced injury in central
PA. However, ozone levels were relatively high in 2011, and the monitor yielded a
1-year 4th-highest daily maximum 8-hr average ozone concentration of 76 ppb. If
ozone concentrations remain at this level or increase, our study region will be in
violation of the NAAQS for ozone. For comparison, data from EPA AIRS Site #42-
027-0100, located 16.8 km northeast of our study site, revealed a 1-year 4th-highest
daily maximum 8-hr average ozone concentration of 73 ppb for 2011, which is less
than the NAAQS for ozone. Also, it should be noted that potted Tree-of-Heaven
Northeastern Naturalist Vol. 21, No. 4
L.K. Seiler, D.R. Decoteau, and D.D. Davis
2014
551
seedlings in the greenhouse developed symptoms following exposure to 60 ppb and
75 ppb ozone, indicating that the species is inherently sensitive to ozone.
We did not observe ozone-induced foliar injury on most Tree-of-Heaven plants
in the field until the ambient SUM60 ozone values reached approximately 14,000
ppb.hrs. This finding implies that a SUM60 value of 14,000 ppb.hrs may be near
the threshold at which Tree-of-Heaven seedlings initiate foliar symptoms, at least
under the environmental conditions of central PA present in 2011 and considering
the fact that the seedlings were watered occasionally and thus were probably
not moisture-stressed. This threshold is slightly greater than other estimates. For
example, SUM60 ozone needed to cause foliar symptoms on other species of
ozone-sensitive plants was estimated at approximately 9000 ppb.hrs or less than 10,000
ppb.hrs in Missouri and South Carolina, respectively (Davis 2009, Davis 2011).
However, we also observed that ozone-induced foliar injury increased even in periods
of low ambient ozone. Therefore, there may be lag time between an increase
in SUM60 ozone and development of ozone injury in sensitive plants, possibly
influenced by environmental conditions such as soil moisture (Davis and Oredovici
2006, Showman 1991, Yuska et al. 2003).
Ozone injury was more severe as the growing season progressed, which is a
result supported by earlier research in our lab (Davis and Coppolino 1974). However,
this observation may not always be related to ozone concentration, because
older leaves are often more affected by ozone than younger leaves (Skelly 2000).
Thus, the increasing sensitivity to ozone over time, as observed in our survey, may
be related to the interaction of leaf age and ozone concentration. Alternatively, as
autumn approaches, deciduous trees begin to shut down, shed their leaves, and
terminate physiological functions; a process that likely makes the leaves more
vulnerable to ozone injury. Further research is needed to identify the important
factors that determine the incidence and severity of ozone-induced foliar damage,
such as mean, peak, or cumulative ozone concentration; exposure duration; species
sensitivity; plant or leaf maturity; and various environmental factors.
Results of this study contribute to our knowledge of exposure/response relationships
between ozone levels and foliar symptoms on ozone-sensitive plant
species, and provide data that can inform the process of setting NAAQS in the US
for photochemical oxidants and ozone (USEPA 2006, 2007, 2010). The EPA has
considered an ozone W126 value secondary standard of 7000 to 15,000 ppb.hrs
(USEPA 2007, 2010). The 2011 W126 value for our study area was 11,600 ppb.hrs.
According to our field observations, a secondary standard of approximately 11,000
ppb.hrs may not be sufficient to protect sensitive vegetation from ozone-induced
foliar damage under moist conditions. Therefore, if a W126 secondary standard is
adopted, a value near or lower than 10,000 ppb.hrs might be best. However, Vander
Heyden et al. (2000) suggested that a value of 10,000 ppb.hrs might be too high to
protect ozone-sensitive vegetation. Similarly, Gravano et al. (2003) reported that
ozone injury on a watered Tree-of-Heaven clone was initiated at only 4000 to 5000
ppb.hrs in central Italy. Clearly, additional field research is needed to accurately
determine a realistic NAAQS for photochemical oxidants and ozone to protect
ozone-sensitive plant species in the United States.
Northeastern Naturalist
552
L.K. Seiler, D.R. Decoteau, and D.D. Davis
2014 Vol. 21, No. 4
Acknowledgments
We thank M. Kaye, J. Savage, J. Ferdinand, A. Hoffman, K. Webber, C. Fitzpatrick, and
J. Millican for their help. We are also grateful for funding from the Pennsylvania Department
of Environmental Protection, Bureau of Air Quality, Harrisburg, PA.
Literature Cited
Bennett, J.P., E.A. Jepsen, and J.A. Roth. 2006. Field responses of Prunus serotina and
Asclepias syriaca to ozone around southern Lake Michigan. Environmental Pollution
142:354–366.
Berrang, P., D.F. Karnosky, and J.P. Bennett. 1989. Natural selection for ozone tolerance
in Populus tremuloides: Field verification. Canadian Journal of Forestry Research
19:519–522.
Berrang, P., D.F. Karnosky, and J.P. Bennett. 1991. Natural selection for ozone tolerance in
Populus tremuloides: An evaluation of nationwide trends. Canadian Journal of Forestry
Research 21:1091–1097.
Davis, D.D. 2007a. Ozone-induced symptoms on vegetation within the Moosehorn National
Wildlife Refuge in Maine. Northeastern Naturalist 14:403–414.
Davis, D.D. 2007b. Ozone injury to vegetation within the Seney National Wildlife Refuge
in the Upper Peninsula of Michigan. Northeastern Naturalist 14:415–424.
Davis, D.D. 2009. Ozone-induced stipple on plants in the Cape Romain National Wildlife
Refuge, South Carolina. Southeastern Naturalist 8:471–478.
Davis, D.D. 2011. Ozone-induced leaf symptoms on vegetation in the Mingo National Wildlife
Refuge Missouri. Northeastern Naturalist 18:115–122.
Davis, D.D., and J.B. Coppolino. 1974. Relative ozone susceptibility of selected woody
ornamentals. HortScience 9:537–539.
Davis, D.D., and T. Orendovici. 2006. Incidence of ozone symptoms on vegetation within
a national wildlife refuge in New Jersey, USA. Environmental Pollution 143:555–564.
Davis, D.D., and F.A. Wood. 1973. The influence of environmental factors on the sensitivity
of Virginia Pine to ozone. Phytopathology 63:371–376.
Gravano, E., V. Giulietti, R. Desotgiu, F. Bussotti, P. Grossoni, G. Gerosa, and C. Tani.
2003. Foliar response of an Ailanthus altissima clone in two sites with different levels
of ozone-pollution. Environmental Pollution 121:137–146.
Grulke, N.E., R. Johnson, A. Esperanza, D. Jones, T. Nguyen, S. Posch, and M. Tausz. 2003.
Canopy transpiration of Jeffrey Pine in mesic and xeric microsites: O3 uptake and injury
response. Trees-Structure and Function 17:292–298.
Heck, W.W., R.B. Philbeck, and J.A. Dunning. 1975. A continuous stirred tank reactor
(CSTR) system for exposing plants to gaseous contaminants: Principles, specifications,
construction and operation. USDA-ARS Pub. No. ARS-S-181, New Orleans, LA.
Hildebrand, E., J.M. Skelly, and T.S. Fredericksen. 1996. Foliar response of ozone-sensitive
hardwood-tree species from 1991 to 1993 in the Shenandoah National Park, VA. Canadian
Journal of Forestry Research 26:658–669.
Horsfall, J.G., and R.W. Barratt. 1945. An improved grading system for measuring plant
disease. Phytopathology 35:655.
Kasson, M.T., M.D. Davis, and D.D. Davis. 2013. The invasive Ailanthus altissima in
Pennsylvania: History, distribution, and growth patterns. Northeastern Naturalist 20
(Monograph No. 10):1–60.
Krupa, S.V. 1997. Air Pollution, People, and Plants. The American Phytopathological Society,
St. Paul, MN, 197 pp.
Northeastern Naturalist Vol. 21, No. 4
L.K. Seiler, D.R. Decoteau, and D.D. Davis
2014
553
Krupa, S.V., M.T. McGrath, C.P. Andersen, F.L. Booker, K.O. Burkey, A.H. Chappelka,
B.I. Chevone, E.J. Pell, and B.A. Zilinskas. 2001. Ambient ozone and plant health. The
American Phytopathological Society 85:4–12.
Manning, W.J., and W.A. Federer. 1980. Biomonitoring Air Pollutants with Plants. Applied
Science Publishers, UK. 142 pp.
Matyssek, R., D. LeThiec, M. Low, P. Dizengremel, A.J. Nunn, and K.H. Haberle. 2006.
Interactions between drought and O3 stress in forest trees. Plant Biology 1:11–17.
Orendovici, T., J.M. Skelly, D.D. Davis, J.A. Ferdinand, J.E. Savage, and R.E. Stevenson.
2007. Ozone uptake (flux) as it relates to ozone-induced foliar symptoms of Prunus
serotina and Populus maximowizii x trichocarpa. Environmental Pollution 151:79–92.
Orendovici-Best, T., J.M. Skelly, and D.D. Davis. 2010. Spatial and temporal patterns of
ground-level ozone within north-central Pennsylvania forests. Northeastern Naturalist
17:247–260.
Panek, J.A. 2004. Ozone uptake, water loss and carbon-exchange dynamics in annually
drought-stressed Pinus ponderosa forests: Measured trends and parameters for uptake
modeling. Tree Physiology 24:277–290.
Reynolds-Henne, C.E., A. Langenegger, J. Mani, N. Schenk, A. Zumsteg, and U. Feller.
2010. Interactions between temperature, drought, and stomatal opening in legumes.
Environmental and Experimental Botany 68:37–43.
Richards, B.L., J.T. Middleton, and W.B. Hewitt. 1958. Air pollution with relation to agronomic
crops. V. Oxidant stipple of grape. Agronomy Journal 50:559–561.
SAS Institute. 2011. SAS software, Version 9.1 of the SAS System for Unix. Cary, NC.
Showman, R.E. 1991. A comparison of ozone injury to vegetation during moist and drought
years. Journal of Air and Waste Management Association 41:63–64.
Skelly, J.M. 2000. Tropospheric ozone and its importance to forest and natural plant communities
of the northeastern United States. Northeastern Naturalist 7:221–236.
Skelly, J.M., D.D. Davis, W. Merrill, E.A. Cameron, H.D. Brown, D.B. Drummond, and
L.S. Dochinger. 1987. Diagnosing injury to eastern forest trees. USDA Forest Service,
Forest Pest Management, Atlanta, GA, and The Pennsylvania State University, College
of Agricultural Sciences, Department of Plant Pathology, University Park, PA, 22 pp.
United States Department of the Interior (USDOI). 2003. Ozone-sensitive plant species on
National Park Service and US Fish and Wildlife Service lands: Results of a 24–25 June
2003 workshop, Baltimore, MD. NPS/NRARD/NRR-2003/0, Denver, CO, 21 pp.
United States Environmental Protection Agency (USEPA). 2003. Ozone: Good up high, bad
nearby. EPA-451/K-03-001, Washington, DC.
USEPA. 2006. Air quality criteria for ozone and related photochemical oxidants, volume
III of III. EPA 600/R-05/004cF, Research Triangle Park, NC.
USEPA. 2007. Review of the national ambient air quality standards for ozone: Policy assessment
of scientific and technical information. EPA-452/R-07-007, Research Triangle
Park, NC.
USEPA. 2010. Proposed rule, national ambient air quality standards for ozone. Federal
Register/Vol. 75, No. 11/Tuesday, 19 January 2010, Research Triangle Park, NC.
Yuska, D.E., J.M. Skelly, J.A. Ferdinand, R.E. Stevenson, J.E. Savage, J.D. Mulik, and
A. Hines. 2003. Use of bioindicators and passive sampling devices to evaluate ambient
ozone concentrations in north central Pennsylvania. Environmental Pollution
125:71–80.