Seasonal Abundance of the Ctenophore Mnemiopsis leidyi in
Relation to Water Temperature and Other Zooplankton in
the Thames River Estuary, Connecticut
Lucy S. Vlietstra
Northeastern Naturalist, Volume 21, Issue 3 (2014): 397–418
Full-text pdf (Accessible only to subscribers. To subscribe click here.)
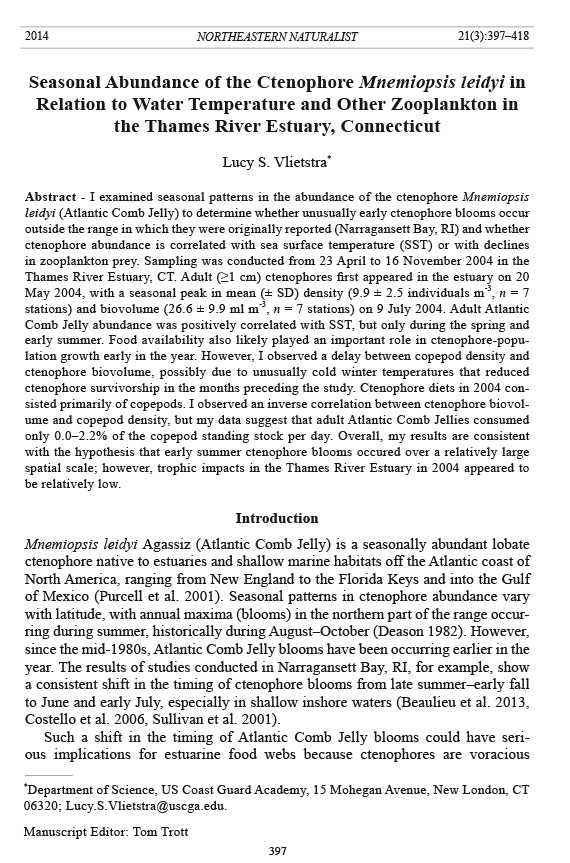
Access Journal Content
Open access browsing of table of contents and abstract pages. Full text pdfs available for download for subscribers.
Current Issue: Vol. 30 (3)
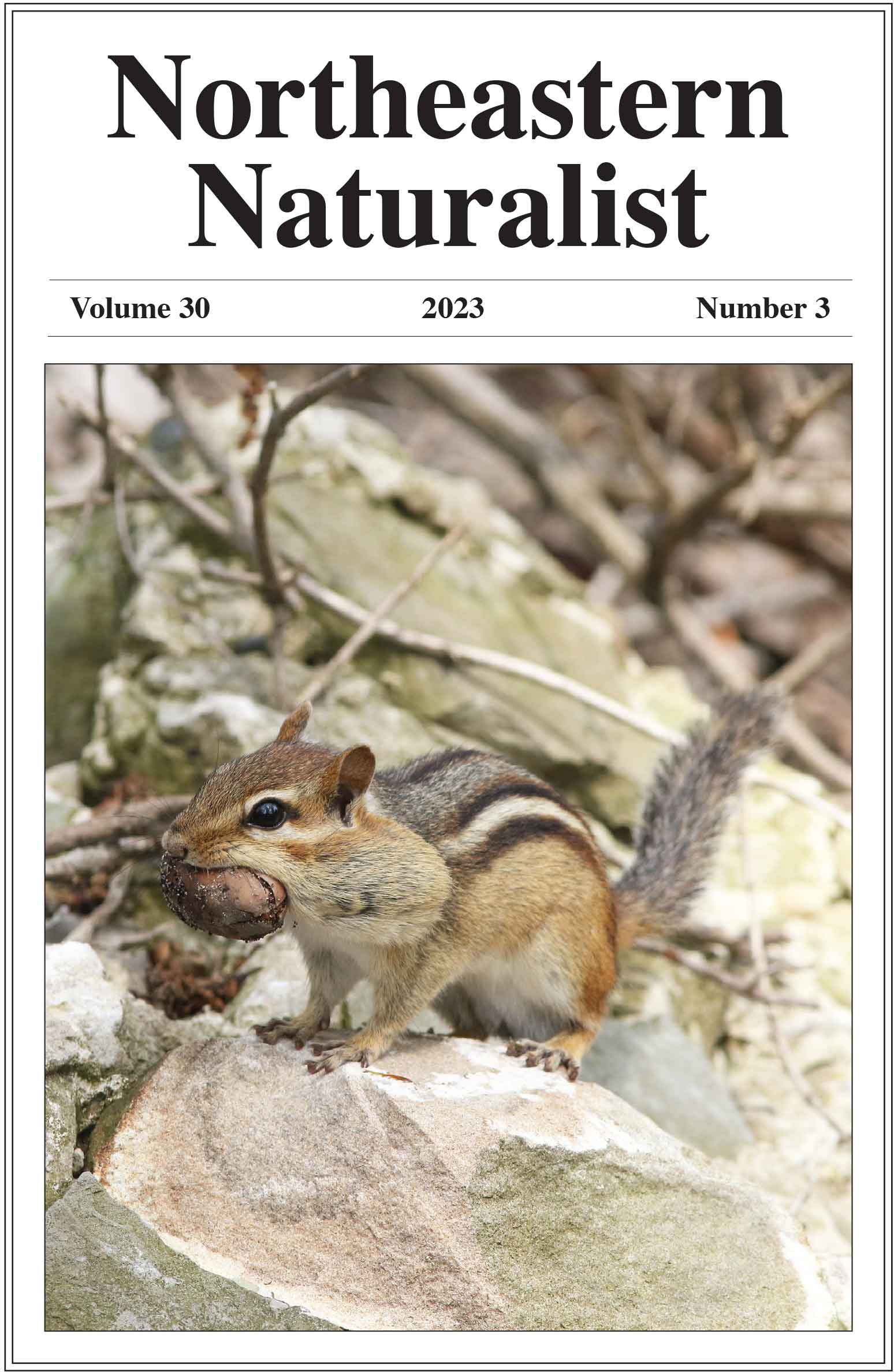
Check out NENA's latest Monograph:
Monograph 22
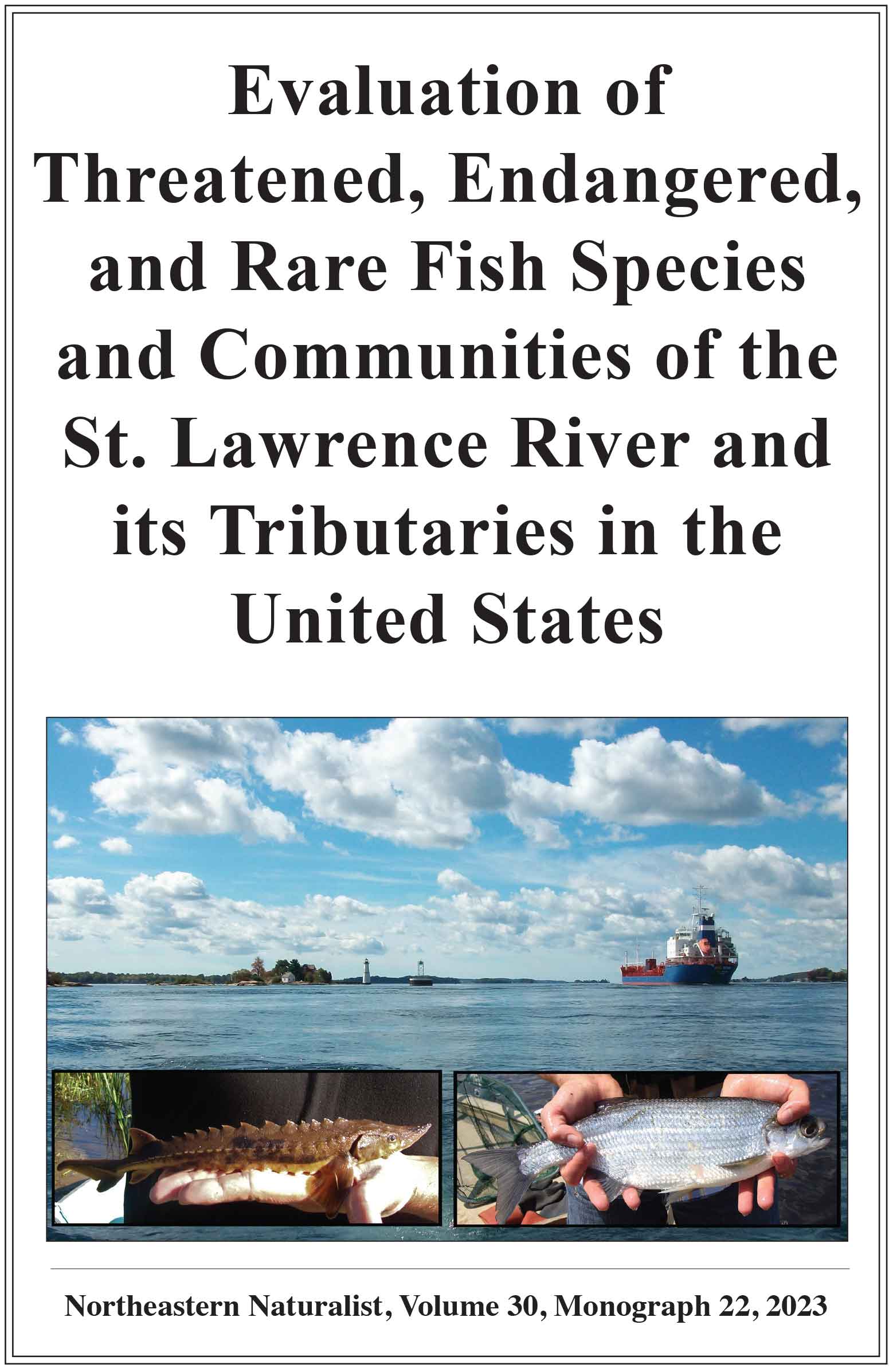
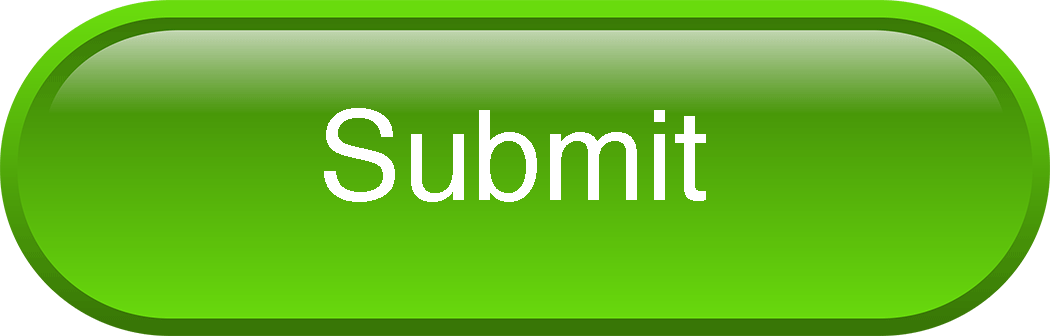
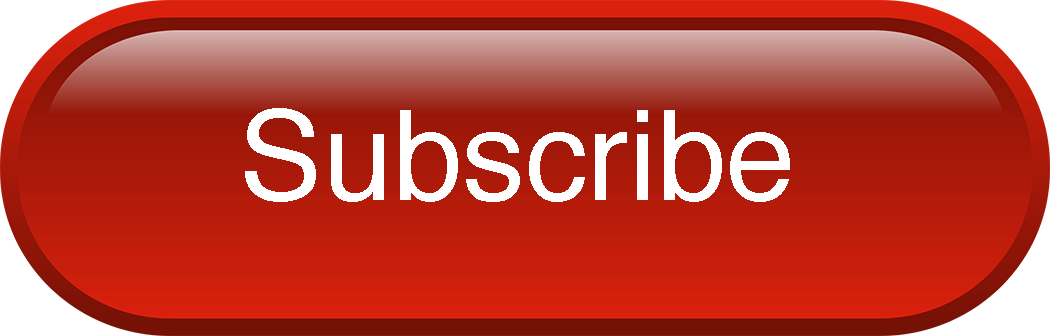
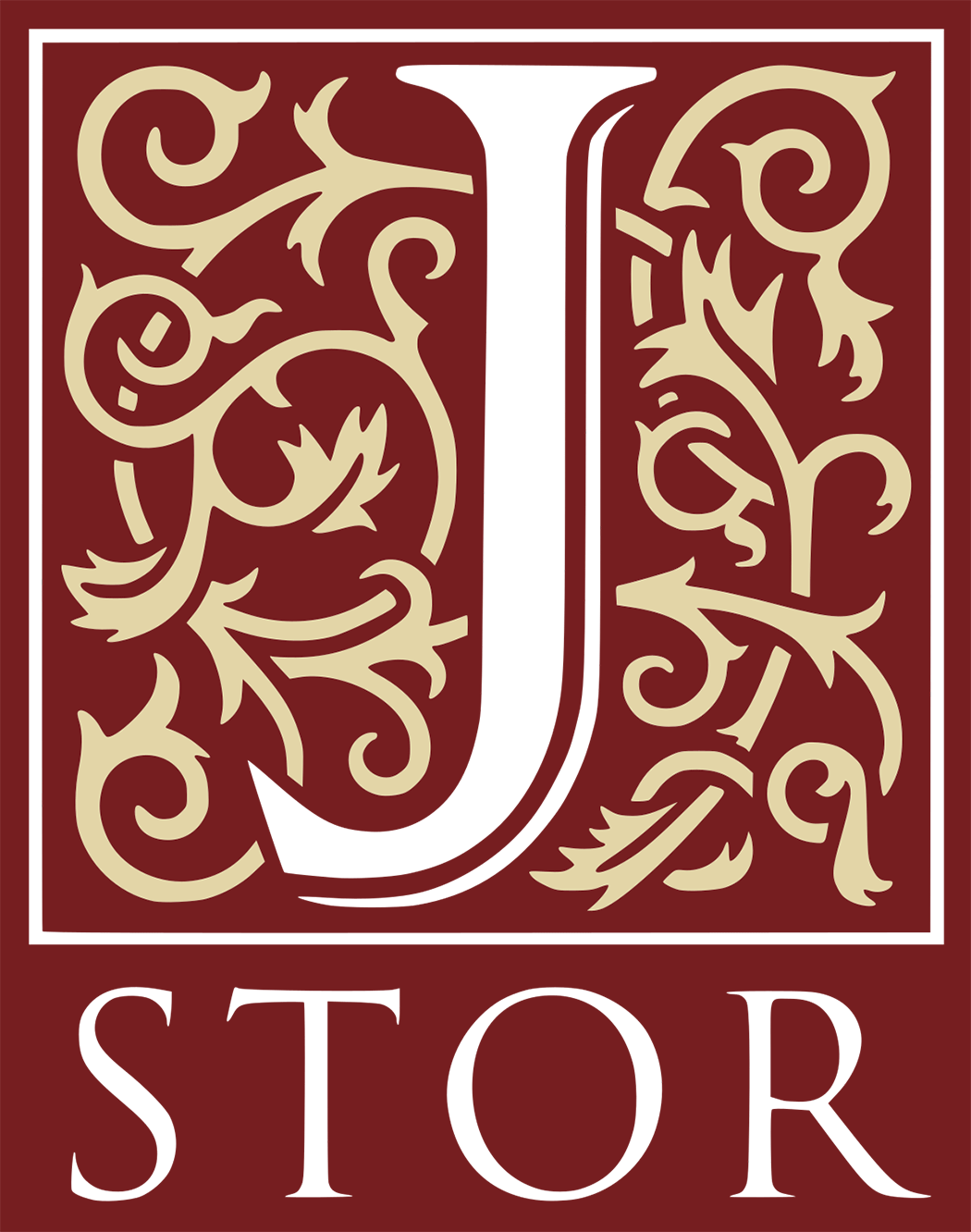


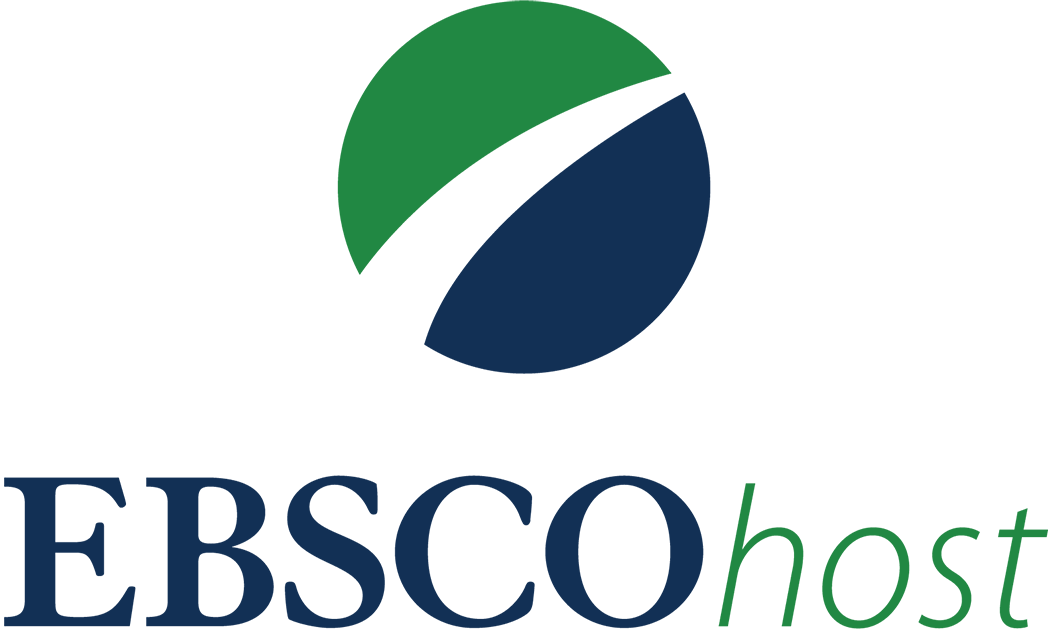

Northeastern Naturalist Vol. 21, No. 3
L.S. Vlietstra
2014
397
2014 NORTHEASTERN NATURALIST 21(3):397–418
Seasonal Abundance of the Ctenophore Mnemiopsis leidyi in
Relation to Water Temperature and Other Zooplankton in
the Thames River Estuary, Connecticut
Lucy S. Vlietstra*
Abstract - I examined seasonal patterns in the abundance of the ctenophore Mnemiopsis
leidyi (Atlantic Comb Jelly) to determine whether unusually early ctenophore blooms occur
outside the range in which they were originally reported (Narragansett Bay, RI) and whether
ctenophore abundance is correlated with sea surface temperature (SST) or with declines
in zooplankton prey. Sampling was conducted from 23 April to 16 November 2004 in the
Thames River Estuary, CT. Adult (≥1 cm) ctenophores first appeared in the estuary on 20
May 2004, with a seasonal peak in mean (± SD) density (9.9 ± 2.5 individuals m-3, n = 7
stations) and biovolume (26.6 ± 9.9 ml m-3, n = 7 stations) on 9 July 2004. Adult Atlantic
Comb Jelly abundance was positively correlated with SST, but only during the spring and
early summer. Food availability also likely played an important role in ctenophore-population
growth early in the year. However, I observed a delay between copepod density and
ctenophore biovolume, possibly due to unusually cold winter temperatures that reduced
ctenophore survivorship in the months preceding the study. Ctenophore diets in 2004 consisted
primarily of copepods. I observed an inverse correlation between ctenophore biovolume
and copepod density, but my data suggest that adult Atlantic Comb Jellies consumed
only 0.0–2.2% of the copepod standing stock per day. Overall, my results are consistent
with the hypothesis that early summer ctenophore blooms occured over a relatively large
spatial scale; however, trophic impacts in the Thames River Estuary in 2004 appeared to
be relatively low.
Introduction
Mnemiopsis leidyi Agassiz (Atlantic Comb Jelly) is a seasonally abundant lobate
ctenophore native to estuaries and shallow marine habitats off the Atlantic coast of
North America, ranging from New England to the Florida Keys and into the Gulf
of Mexico (Purcell et al. 2001). Seasonal patterns in ctenophore abundance vary
with latitude, with annual maxima (blooms) in the northern part of the range occurring
during summer, historically during August–October (Deason 1982). However,
since the mid-1980s, Atlantic Comb Jelly blooms have been occurring earlier in the
year. The results of studies conducted in Narragansett Bay, RI, for example, show
a consistent shift in the timing of ctenophore blooms from late summer–early fall
to June and early July, especially in shallow inshore waters (Beaulieu et al. 2013,
Costello et al. 2006, Sullivan et al. 2001).
Such a shift in the timing of Atlantic Comb Jelly blooms could have serious
implications for estuarine food webs because ctenophores are voracious
*Department of Science, US Coast Guard Academy, 15 Mohegan Avenue, New London, CT
06320; Lucy.S.Vlietstra@uscga.edu.
Manuscript Editor: Tom Trott
Northeastern Naturalist
398
L.S. Vlietstra
2014 Vol. 21, No. 3
consumers of copepods, fish eggs, and possibly fish larvae (Cowan and Houde
1993, Kremer 1979, Purcell et al. 1994). Because fish production in New England
usually peaks during spring and early summer, high abundances of ctenophores
in early summer could reduce fish populations through predation on icthyoplankton
or competition with larval fishes (Purcell et al. 2001, Sullivan et al. 2001).
Evidence of ctenophores exerting top-down control in local food webs has been
documented in Narragansett Bay, RI, where high Atlantic Comb Jelly abundance
was followed by low copepod densities and high values of chlorophyll-a (Costello
et. al 2006, Deason and Smayda 1982).
One hypothesis for the cause of early ctenophore blooms involves warm spring
temperatures associated with positive phases of the North Atlantic Oscillation
(NAO). Hawk (1998) and Sullivan et al. (2001) described a warming trend in waters
off Rhode Island over the last several decades, during which time the NAO shifted
from a negative phase to a positive one. During positive phases, currents bring
relatively warm water to the northern Atlantic Coast of North America (Ottersen et
al. 2001). This situation could account for a recent shift in the timing of seasonal
ctenophore blooms because water temperature appears to be an important factor
limiting the onset of ctenophore blooms in New England and elsewhere along the
Atlantic Coast (Purcell et al. 2001, Sullivan et al. 2001).
If early summer Atlantic Comb Jelly blooms are associated with a large-scale
atmospheric–oceanographic coupling mechanism, such as the NAO, early blooms
would be expected in other estuaries in the northern native range. I conducted this
study to determine whether Atlantic Comb Jelly blooms also occured during early
summer in a small estuary located approximately 70 km west of Narragansett Bay,
on the northern shore of Long Island Sound. In addition, I sought to define the relationship,
if any exists, between sea surface temperature (SST) and Atlantic Comb
Jelly abundance in the estuary as well as the potential impact of the ctenophore
bloom on the abundance of local prey populations, specifically copepods, fish eggs,
and fish larvae.
Field-Site Description
I conducted this study from 23 April to 16 November 2004 in the Thames River
Estuary, CT (41°21'14.8''N, 72°6'3.6''W; Fig. 1). The Thames River headwaters are
located at the junction of the Yantic and Shetucket Rivers in Norwich, CT, approximately
25 km upstream of Long Island Sound. I sampled in the lower reaches of the
estuary, where the Thames River is up to 15 m deep and 0.5–1.5 km wide and covers
an area of approximately 10.8 km2. Tides are semi-diurnal, and the water column
has a well-defined salt-wedge structure for most of the year. Surface and bottom
salinity typically range from 5 to 20 ppt (parts per thousand) and from 25 to 33 ppt,
respectively, with the pycnocline at 2–4 m depth.
The Thames River Estuary supports a wide variety of fishes, including Centropristis
striata L. (Black Sea Bass), Morone saxatilis (Walbaum) (Striped Sea Bass),
Stenotomus chrysops (L.) (Scup), Anchoa mitchilli (Valenciennes) (Bay Anchovies),
Peprilus triacanthus Peck (Butterfish), and several flounders, including both
Northeastern Naturalist Vol. 21, No. 3
L.S. Vlietstra
2014
399
Pseudopleuronectes americanus (Walbaum) (Winter Flounder) and Paralichthyes
dentatus (L.) (Summer Flounder) (K. Mrakovcich, US Coast Guard Academy, New
London, CT, unpubl. data).
Methods
I measured ctenophore abundance in the water column approximately every 2
weeks, with replicate (n = 2) vertical-net tows conducted at 7 fixed stations (stations
0–6; Fig. 1). Sampling was omitted at some stations in the first 2 weeks of
the study while the protocol was established and occasionally thereafter when
maritime traffic restricted access to portions of the estuary. Tows were made with
a 63-mm-mesh plankton net lowered to a depth of 6 m (after Sullivan et al. 2001).
Immediately following each tow, I recorded the number of Atlantic Comb Jellies
caught, and measured the oral-aboral diameter to the nearest 0.1 cm. When I collected
too many ctenophores for individual measurement, I visually assessed the
Figure 1. Map of Thames River
Estuary showing the location
of sampling stations 0–6 (●).
USCGA = campus of US Coast
Guard Academy.
Northeastern Naturalist
400
L.S. Vlietstra
2014 Vol. 21, No. 3
number in each size class (estimated to the nearest 0.1 cm). I gently transferred a
subsample of individuals representing the range of sizes observed to a separate container
for biovolume measurements in the laboratory. I measured the displacement
volume of individual ctenophores and developed an equation for the length–volume
relationship for the purpose of calculating biovolume of the total population. This
relationship was
y = 0.0011 x2.57,
where x was oral-aboral length (r2 = 0.90, n = 79).
I characterized ctenophore abundance in terms of density (individuals m-3) and
biovolume (ml m-3), and calculated density from the total number of individuals
collected in each tow divided by the volume of water (m3) sampled by the tow. The
volume (V) was calculated with the equation,
V = π r2 * z,
where r was the radius of the net, and z was the distance of the tow. Biovolume
was calculated from the total volume of ctenophore biomass (ml) in each tow
divided by V. I averaged density and biovolume across replicate tows at each
station. To derive abundance estimates for the study site as a whole, I averaged
mean ctenophore density and biovolume station-1 across sampling stations. This
yielded a grand mean (± standard error [SE]) ctenophore abundance at the study
site on each sampling date, with standard error representing variance among
sampling-station means.
Immediately after collecting samples, I inspected them to detect ctenophores
with intact gut contents. I removed these individuals from the sample and stored
them in a separate container for a period of 0.5–1.5 h until I could get them to the
laboratory, where I inspected prey items inside the gut with a dissecting microscope
and identified each item to the lowest possible taxonomic level. Because some prey
species were likely digested or expelled during transport (Granhag et al. 2011),
these measures were not intended for quantitative analysis but to qualitatively identify
important prey species. I ranked each species by its frequency of occurrence
(FO) in gut samples to determine relative dietary importance.
After sorting Atlantic Comb Jellies in the field, I preserved the remaining plankton
sample in 80% ethyl alcohol. Beginning on 7 May 2004, I used a Folsom splitter
to subsample preserved samples from Stations 0, 2, 3, 5, and 6, and transferred them
to a counting wheel. I identified every member of the mesoplankton (0.2–2.0 mm)
and macroplankton (2–20 mm) in the subsample, including holoplankton and meroplankton,
to the lowest possible taxonomic level. The total number of individuals
of each taxon was back-calculated from the number of splits used to derive the
subsample. I determined seasonal patterns in non-gelatinous zooplankton density
(individuals m-3) by dividing the number of individuals of each species in whole
samples by V and averaging replicate tows per station. Those values were averaged
across all stations to obtain a grand mean (± SE) zooplankton abundance for the
study site as a whole, with standard error representing variance among samplingstation
means.
Northeastern Naturalist Vol. 21, No. 3
L.S. Vlietstra
2014
401
I characterized impacts of Atlantic Comb Jelly consumption on lower trophic
levels by the correlation, if any existed, between ctenophore biovolume and the
ambient density of the single species most frequently observed in ctenophore diets,
which was copepods (see Results). I used ctenophore biovolume for this analysis
because prey consumption has been shown to vary with ctenophore length (Granhag
et al. 2011), making biovolume a better indicator of consumption potential than
ctenophore density. I performed correlation analysis on raw values of ctenophore
biovolume (larvae and adults combined) and copepod density collected in the same
tow. Because neither parameter fit a normal distribution with equal variance even
after log-transformation was applied, the correlation was examined with a Spearman
rank test.
I further characterized trophic impacts by estimating the percentage of copepod
standing stock consumed by Atlantic Comb Jellies on a daily basis using the relationship
between copepod density and copepod-consumption rate by adult comb
jellies in air-saturated water as defined by Decker et al. (2004): y = 0.05x - 0.07,
where x is ambient copepod density (mean number of copepods L-1) and y is the
number of copepods consumed per unit time (number of copepods h-1 ml ctenophore-
1). Values of y were multiplied by adult ctenophore biovolume, divided by
mean copepod density, and multiplied by 100 to derive the % copepod standing
stock consumed by ctenophores at each station per day. I made calculations for
each station sampled on a given day and averaged these values to estimate the
mean percent (± SE) copepod standing stock consumed in the estuary as a whole.
Because copepods likely have a patchy distribution in the water column, individual
ctenophores may encounter densities much different from mean values used for x
(i.e., mean ambient copepod density). However, because consumption rates were
calculated over a relatively large spatial scale, x was a useful estimate of ctenophore-
prey availability. Prey consumption by larval ctenophores, which specialize
on microphytoplankton, microzooplankton, and copepod nauplii (Sullivan and Gifford
2004, Waggett and Sullivan 2006), were not included in the analysis for the
purpose of comparison with other studies.
I acquired surface and mid-water temperature (°C) and salinity (ppt) for the
period 23 April–November 2004 from a mooring station maintained by the University
of Connecticut (http://sounddata.uconn.edu/) adjacent to station 3 (Fig. 1).
Surface temperatures were measured at 0.3 m depth; mid-water temperatures were
measured at 4.4 m depth. Following Sullivan et al. (2001), I derived daily water
temperatures by averaging hourly data. Water temperature and salinity were not
available from the mooring on the final sampling date, 16 November 2004, so I
measured hydrographic parameters at midday with a hand-held YSI 6500 sonde.
Correlations between ctenophore abundance (both density and biovolume) at
station 3 and SST were examined with a Spearman rank test. In addition, water
column stability (E) was calculated according to Knauss (1997):
E = α (Ts - Tb) / D + β (Ss - Sb) / D,
where α is 1.5; β is -7.6; Ts is surface temperature, Tb is mid-water temperature, Ss
Northeastern Naturalist
402
L.S. Vlietstra
2014 Vol. 21, No. 3
is surface salinity, Sb is mid-water salinity, and D is depth. Following Costello et al.
(2006), the water column was considered mixed when E > 2 x 10-4 m-1.
Because winter SST may influence overwintering survivorship in ctenophores,
and therefore population growth in the spring (Costello et al. 2006, Shiganova et
al. 2001), I evaluated winter conditions prior to the study to determine whether
they were unusually warm or cold. Data for this comparison were obtained from
the National Oceanic and Atmospheric Administration (NOAA) weather station located
on the east bank of the Thames River, adjacent to station 2 (station #461490,
41°21'6.0''N, 72°5'3.8''W, http://tidesandcurrents.noaa.gov). To characterize daily
SST leading up to the study, I averaged hourly data every 24 h from 1 November
2003 to 31 March 2004. I also used this method to calculate daily mean SST during
1 November–31 March in each of the 8 previous winters (1995–1996 to 2002–
2003). Long-term (1995–2003) means ± 95% CI were plotted on the same graph as
daily SST in winter 2003–2004 for visual comparison.
Results
I sampled in the Thames River Estuary every 10 ± 5 days (mean ± SD) for a total
of 21 days during the study. On most days, I made replicate tows (n = 2) at each of
the 7 stations; exceptions are noted in Table 1.
I first observed Atlantic Comb Jelly larvae (<1 cm) and adults (≥1 cm) in the
estuary on 12 May and 20 May 2004, respectively. Adult densities remained relatively
low (0.0–0.2 individuals m-3) until 16 June 2004, when abundance began to
rise sharply, peaking on 9 July 2004 (9.9 ± 1.0 ind m-3, n = 7 stations). I observed a
smaller peak (8.3 ± 2.0 individuals m-3, n = 7 stations) on 5 September 2004 (Fig.
2a). Adult biovolume also showed a bimodal pattern, with a maximum value (26.6
± 3.7 ml m-3, n = 7 stations) observed on 9 July 2004 and a smaller peak (17.2 ± 4.7
ml m-3, n = 7 stations) on 5 September 2004 (Fig. 2b). Seasonal patterns in adult
ctenophore abundance were generally consistent among sampling stations, with
two peaks evident at each station during the study (Figs. 3d–f, 4d–f).
Larvae were generally 2–3 times more abundant than adults in the estuary, with
peak densities measured on 28 October 2004 (77.3 ± 33.4 ind m-3, n = 7 stations,
Fig. 2a). On that date, ctenophore larvae were 16 times more abundant than adults,
with the highest densities at downstream stations (stations 0–2; Fig. 3a). Despite
their high densities, larvae usually accounted for only 5–25% of total Atlantic
Comb Jelly biovolume, with the greatest larval biovolume (2.2 ± 0.4 ml m-3, n = 7
stations) observed on 30 June 2004 (Fig. 2b).
Ctenophore diet
I examined gut contents from 21 Atlantic Comb Jellies collected 20 May–16
July 2004; individuals were 0.4–5.7 cm in length. I found copepods (all life stages)
in 87% of the samples (FO = 0.87; Table 1), making them the most widespread
prey item found in ctenophore diets. Most adult copepods were members of Order
Calanoida, mainly Acartia (Acanthacartia) tonsa Dana (FO = 0.74), but Centropages
spp. (FO = 0.09) and Temora longicornis (O.F. Müller) (FO = 0.04) were also
Northeastern Naturalist Vol. 21, No. 3
L.S. Vlietstra
2014
403
Table 1. Mean ± SE abundance of adult and larval Atlantic Comb Jellies and copepods (all age classes) in the Thames River Estuary, 2004. Sample size
(n) is number of stations sampled day-1. Sampling was not conducted at stations 0, 5, and 6 on April 23; stations 0, 1, 5, and 6 on 7 May; stations 0 and 6
on 12 May; station 2 on 22 July; and station 0 on 16 October. We conducted only 1 tow at stations 1–4 on 23 April, stations 2–4 on 7 May, station 1 on 16
October, and station 2 on 28 October and 16 November. Copepods were not sampled on 16 October.
Larvae (<1 cm) Adults (≥1 cm) Copepods
Date n Density (individuals m-3) Biovolume (ml m-3) Density (individuals m-3) Biovolume (ml m-3) n Density (individuals m-3)
23 April 4 0.0 ± 0.0 0.0 ± 0.0 0.0 ± 0.0 0.0 ± 0.0 0 −
7 May 3 0.0 ± 0.0 0.0 ± 0.0 0.0 ± 0.0 0.0 ± 0.0 2 1335 ± 97
12 May 5 0.2 ± 0.2 <0.1 ± <0.1 0.0 ± 0.0 0.0 ± 0.0 3 8576 ± 5322
20 May 7 0.2 ± 0.5 <0.1 ± <0.1 0.2 ± 0.2 0.7 ± 0.7 5 20,386 ± 5300
26 May 7 0.2 ± 0.2 <0.1 ± <0.1 0.0 ± 0.0 0.0 ± 0.0 5 12,478 ± 2399
3 Jun 7 0.3 ± 0.3 <0.1 ± <0.1 0.2 ± 0.2 0.2 ± 0.2 5 14,037 ± 1654
10 Jun 7 0.2 ± 0.2 <0.1 ± <0.1 0.0 ± 0.0 0.0 ± 0.0 5 9374 ± 1245
16 Jun 7 2.5 ± 1.3 0.2 ± 0.1 1.2 ± 0.5 6.3 ± 5.0 5 12,624 ± 1793
24 Jun 7 5.7 ± 1.6 0.5 ± 0.2 2.0 ± 0.8 7.0 ± 6.1 5 17,080 ± 5388
30 Jun 7 24.9 ± 3.3 2.2 ± 0.4 7.6 ± 1.3 9.5 ± 2.5 5 11,497 ± 1030
9 Jul 7 21.1 ± 2.4 2.2 ± 0.5 9.9 ± 1.0 26.6 ± 3.7 5 10,433 ± 3598
16 Jul 7 16.0 ± 4.2 1.7 ± 0.4 6.6 ± 1.7 9.4 ± 3.1 5 9791 ± 1374
22 Jul 6 6.1 ± 2.5 0.8 ± 0.3 5.5 ± 2.2 10.5 ± 5.4 4 7742 ± 1409
6 Aug 7 1.9 ± 0.9 0.2 ± 0.1 4.2 ± 1.2 12.2 ± 4.0 5 12,090 ± 3264
17 Aug 7 5.9 ± 1.2 0.4 ± 0.1 4.6 ± 0.9 17.0 ± 7.2 5 3842 ± 1056
28 Aug 7 11.1 ± 2.1 1.4 ± 0.4 4.0 ± 1.6 13.2 ± 7.1 5 5616 ± 1397
5 Sep 7 22.8 ± 8.8 1.6 ± 0.7 8.3 ± 2.0 17.2 ± 4.8 5 6480 ± 1365
25 Sep 7 43.2 ± 16.1 1.9 ± 0.2 5.5 ± 1.9 6.8 ± 3.0 5 8428 ± 690
16 Oct 6 8.6 ± 2.5 1.2 ± 0.5 3.4 ± 0.9 4.7 ± 2.9 0 −
28 Oct 7 77.3 ± 33.4 1.8 ± 0.4 4.9 ± 1.6 4.9 ± 1.9 5 2084 ± 411
16 Nov 7 34.7 ± 15.3 0.4 ± 0.1 1.2 ± 0.4 5.6 ± 2.5 5 1706 ± 341
Northeastern Naturalist
404
L.S. Vlietstra
2014 Vol. 21, No. 3
common. Copepod prey also included adult harpacticoid copepods (FO = 0.13) and
unidentified copepidites and nauplii (FO = 0.52). Other common prey items were:
barnacle nauplii (FO = 0.70), bivalve veligers (FO = 0.48), and cladocerans (FO =
0.48) (Table 2). Less common were mysid shrimp, crab zoea, and tunicate larvae
(Table 2). I observed neither fish eggs nor fish larvae in gut samp les.
Non-gelatinous zooplankton
Non-gelatinous mesozooplankton and macrozooplankton in the water column
consisted mainly of crustaceans (Fig. 5), which represented 81% of all
Figure 2. Mean (± SE) (a) density and (b) biovolume of adult (≥1 cm) and larval (<1 cm)
Atlantic Comb Jellies in the Thames River Estuary, 23 April-16 November 2004. Standard
error bars represent variation among mean ctenophore abundance at each sampling station.
Sample sizes are shown in Table 1.
Northeastern Naturalist Vol. 21, No. 3
L.S. Vlietstra
2014
405
non-gelatinous zooplankton collected in nets. The most abundant crustaceans were
copepods and barnacle larvae (15% and 81% of crustaceans, respectively). Molluscs
(12% overall) were also relatively abundant, specifically bivalve veligers
(76% of molluscs), as were polychaete larvae (6% overall). Icthyoplankton represented
0.1% (by number) of all zooplankton counted. Fish eggs were collected
sporadically during 7 May–28 August, with a maximum density of 37 ± 29 eggs m-3
Figure 3. Mean (± SE) density of larval (<1 cm) Atlantic Comb Jellies collected in replicate
(n = 2) tows conducted at (a) stations 0-2, (b) stations 3 and 4, and (c) stations 5 and
6. Mean (± SE) density of adult (≥1 cm) Atlantic Comb Jellies collected in replicate tows
at (d) stations 0–2; (e) stations 3 and 4, and (f) stations 5 and 6. Note the different y-axis
scales used for larvae and adults.
Northeastern Naturalist
406
L.S. Vlietstra
2014 Vol. 21, No. 3
(n = 5 stations) on 3 June. I observed fish larvae in samples collected 26 May–30
June, with a maximum density of 15 ± 10 individuals m-3 (n = 5 stations) on 24 June.
Densities of non-gelatinous zooplankton (all species) were greatest during 20
May–30 June 2004 (Fig. 5a). Copepods, specifically, were most abundant on 20
May 2004 (20,384 ± 5300 individuals m-3, n = 5 stations), after which time they
declined to 12,478 ± 2399 individuals m-3 (n = 5 stations, Fig. 6). Copepod densities
Figure 4. Mean (± SE) biovolume of larval (<1 cm) Atlantic Comb Jellies collected in replicate
(n = 2) tows conducted at (a) stations 0–2, (b) stations 3 and 4, and (c) stations 5 and
6. Mean (± SE) biovolume of adult (≥1 cm) Atlantic Comb Jellies collected in replicate tows
at (d) stations 0-2, (e) stations 3 and 4, and (f) stations 5 and 6. Note the different y-axis
scales used for larvae and adults.
Northeastern Naturalist Vol. 21, No. 3
L.S. Vlietstra
2014
407
remained relatively stable until 17 August 2004, when they declined to 3842 ±
1046 individuals m-3 (n = 5 stations). On 25 September 2004, densities increased to
8428 ± 690 individuals m-3 (n = 5 stations) before decreasing again in late October
(Fig. 6). Overall, I detected a negative correlation between Atlantic Comb Jelly
biovolume (all sizes) and copepod density measured in the same tow (rs = -0.31,
df = 145, P < 0.001).
I estimated that, on average, adult Atlantic Comb Jellies in the Thames River
Estuary consumed 0.0–2.8% of the standing stock of copepods day-1 (n = 19 days;
Fig. 7). Prey-consumption rates were highest during the ctenophore bloom on 9
July 2004, when adult Atlantic Comb Jellies consumed between 2.8 ± 0.5% (n = 5
stations) of the copepod standing stock (Fig. 7), with the greatest portion (4.1%)
consumed at station 5.
Correlation with water temperature
During the study, SST at station 3 was 9.7–22.8 °C and surface salinity was
6–24 ppt (Fig. 8a, b). Mid-water temperature and salinity ranged from 7 °C to 21
°C and from 28 ppt to 30 ppt, respectively (Fig. 8a, b). Water column stability (E)
exceeded the threshold for stratification for most of the study, which is consistent
with the salt-wedge structure of the water column. Exceptions occurred on 18 and
29 September 2004, when E < 2 x 10-4 m-1, which indicated that the water column
had become mixed to a depth of at least 4.4 m (Fig. 8c).
Table 2. Frequency of occurrence (FO) of prey items found in the guts of Atlantic Comb Jellies (n =
21) collected in the Thames River Estuary, May–June 2004.
Specimen FO
Phylum Annelida
Polycheate larvae (spinoid) 0.35
Phylum Mollusca
Gastropod larvae 0.35
Bivalve larvae (veliger) 0.48
Phylum Arthropoda
Copepoda 0.87
Calanoida (adult) 0.74
Acartia spp. 0.74
Centropages spp. 0.09
Temoral longicornis 0.04
Harpacticoida 0.13
Unid. copepidites and nauplii 0.52
Cladocera 0.48
Podon spp. 0.48
Evadne spp. 0.09
Mysida 0.13
Decapoda (crab zoea) 0.17
Cirripedia (barnacle nauplii and cypris) 0.70
Phylum Chordata
Actinula larvae 0.09
Northeastern Naturalist
408
L.S. Vlietstra
2014 Vol. 21, No. 3
At station 3, both density and biovolume of adult Atlantic Comb Jellies were
positively correlated with SST (density: rs = 0.36, df = 38, P = 0.021; biovolume:
rs = 0.40, df = 38, P = 0.010). However, the correlation was not always strong;
SST increased from 14 °C to 17 °C during April-early May, but Atlantic Comb
Jelly density remained equal or close to 0.0 individuals m-3 until mid-June. In
addition, ctenophore density declined when SSTs reached their highest values in
mid-summer (16 Jul–17 Aug 2004). Because factors controlling population growth
may differ from factors controlling population decline (e.g., temperature vs. food),
ctenophore abundance and SST measured prior to the 9 July bloom (23 April–9 July
Figure 5. Mean (± SE) density of all non-gelatinous meso- and macrozooplankton collected
in vertical-net tows, 7 May–16 November 2004. Standard error bars represent variance
among sampling stations. Note differences in scale between (a) and (b).
Northeastern Naturalist Vol. 21, No. 3
L.S. Vlietstra
2014
409
Figure 6. Mean (± SE) copepod density and mean (± SE) Atlantic Comb Jelly biovolume
(all sizes) among sampling stations in the Thames River Estuary, 2004. Sample sizes are
given in Table 1.
Figure 7. Mean (± SE) % of copepod (all life stages) standing stock consumed by Atlantic
Comb Jellies in the Thames River Estuary, 7 May–16 November 2004. Zeros represent days
when adult ctenophores were not collected in net tows. Sample sizes are provided in Table 1.
Northeastern Naturalist
410
L.S. Vlietstra
2014 Vol. 21, No. 3
Figure 8. Daily (a) sea surface temperature (°C), (b) salinity (ppt), and (c) water column
stability index (E) in the Thames River Estuary, 23 April–16 November 2004.
Northeastern Naturalist Vol. 21, No. 3
L.S. Vlietstra
2014
411
2004) were analyzed (post hoc) separately from those measured after the bloom (16
July–16 November 2004). Correlations were still positive for the periods before
the bloom (density: rs = 0.59, df = 18, P = 0.006; biovolume: rs = 0.56, df = 18,
P = 0.010) but not afterwards (density: rs = -0.10, df = 18, P = 0.677; biovolume:
rs = 0.003, df = 18, P = 0.990) (Fig 9).
Likewise, larval ctenophore abundance at station 3 was not correlated with SST
during the study (density: rs = 0.18, df = 38, P = 0.267; biovolume: rs = 0.25, df
= 38, P = 0.125) unless I analyzed values prior to 9 July separately. In that case,
larval abundance was positively correlated with SST (density: rs = 0.57, df = 18,
P = 0.009; biovolume: rs = 0.60, df = 18, P = 0.005; Fig. 9). Larval abundance was
not correlated with SST after the bloom (density: rs = -0.25, df = 18, P = 0.285;
biovolume: rs = -0.15, df = 18, P = 0.516; Fig. 9).
Figure 9. Sea surface temperature (SST) at station 3 and the (a) density and (b) biovolume
of adult Atlantic Comb Jellies at station 3 on and before 9 July 2004 (closed circles) and
after 9 July 2004 (open circles). SST at station 3 and the (c) density and (d) biovolume of
larval Atlantic Comb Jellies at station 3 on and before 9 July 2004 (closed circles) and after
9 July 2004 (open circles). Trend lines show significant (P < 0.05) correlations.
Northeastern Naturalist
412
L.S. Vlietstra
2014 Vol. 21, No. 3
During the winter months preceding the study, November 2003–March 2004,
SST in the Thames River Estuary ranged from 0.9 °C to 14.4 °C (Fig. 10). Overall,
temperatures were cool relative to average winter temperatures during 1995–
2003. The December-February period was especially cold; daily SST was up to
3.9 °C below the long-term mean. Occasionally, SST exceeded average winter
temperatures for periods lasting a few days, but below-average temperatures were
more common.
Discussion
The seasonal patterns I observed in adult Atlantic Comb Jelly abundance in the
Thames River Estuary are consistent with the hypothesis that early summer ctenophore
blooms occur outside the range in which they were originally reported in the
northeast. Both their date of first appearance (20 May 2004) and peak abundance
(9 Jul 2004) coincide with early blooms previously described in Narragansett Bay,
RI (Beaulieu et al. 2013; Sullivan et al. 2001, 2008). Similar phenological shifts in
the northern part of the range have since been described by McNamara et al. (2010),
who found that Atlantic Comb Jelly blooms in two Long Island estuaries occurred
2–3 months earlier in 2006 than blooms in the 1970s and 1980s. In addition, Condon
and Steinberg (2008) found that blooms in the York River Estuary, Chesapeake
Bay, VA occurred 1 month earlier than they did in the late 1960s. Without historical
records from the Thames River Estuary, I could not determine whether the early
Figure 10. Mean daily SST in the Thames River Estuary, November-March from 1995–96 to
2002–03 (solid line, n = 8) and in 2003–04 (white circles). Dotted lines represent the 95%
confidence interval around the long-term (1995–2003) mean.
Northeastern Naturalist Vol. 21, No. 3
L.S. Vlietstra
2014
413
July bloom I observed represents a temporal shift compared to past decades, but
the pattern supports the idea that mechanisms promoting early summer ctenophore
blooms operate on a relatively large spatial scale.
In general, factors driving the onset of ctenophore blooms are not fully understood,
but the results of this study and others suggest that water temperature plays
an important role. Water temperature appears to influence overwintering survivorship
in ctenophores as well as metabolic rates and egg production (Condon and
Steinberg 2008; Costello et al. 2006; Kremer 1979, 1994). Overall, both adult
and larval Atlantic Comb Jelly abundances in the Thames River Estuary were positively
correlated with SST during periods of population growth in early summer,
before the first adult bloom, suggesting that rising water temperature stimulates
reproduction. However, the relationship between temperature and ctenophore abundance
was not always linear. For example, when adult Atlantic Comb Jellies first
appeared in the estuary on 20 May 2004, both adults and larvae were scarce until
16 June 2004 despite an 8 °C (from 14 to 22 °C) increase in SST during that time.
Because the minimum temperature threshold for ctenophore egg production in native
habitats is 10 °C (Costello et al. 2006) and larvae hatch 20–24 h after eggs are
produced (Purcell et al. 2001), the lack of population growth in the spring probably
did not reflect unfavorable temperatures for reproduction.
Laboratory studies have shown that egg production in Atlantic Comb Jelly
is highly sensitive to prey density, and adults stop producing eggs after only a
few days without food (Purcell et al. 2001, Reeve et al. 1989). In the Thames
River Estuary, the main food of Atlantic Comb Jellies was copepods and other
crustaceans, which were not locally abundant until 20 May 2004. This date occurred
3 weeks after SSTs exceeded the minimum threshold for egg production
and coincided with the first appearance of adult ctenophores in the estuary. This
observation is important because it suggests that both SST and food availability
must be adequate before habitats become suitable for ctenophores in the spring.
However, if water temperature and food availability were the only factors limiting
spring population growth in ctenophores, one would expect a larger increase in
ctenophores following the peak in copepod abundance. Instead, ctenophores remained
scarce for the next 4 weeks. Such a delay may be due, in part, to the time
required for foraging adult ctenophores to produce eggs, and for eggs to mature
into larvae and eventually into adults. However, that idea is not consistent with
the fact that adult Atlantic Comb Jellies typically experience high fecundity, and
larvae reach sexual maturity at a relatively young age (14 days; Reeve and Walter
1978 reviewed by Costello et al. 2012).
Alternatively, delayed ctenophore production in the spring of 2004 may be
related to the unusually low surface temperatures observed in the winter months
preceding the study. Shiganova et al. (2001) found that Atlantic Comb Jellies in the
Black Sea were smaller and less abundant in spring months following cold winters.
In addition, individuals were practically absent from inshore waters, where winter
temperatures had reached their lowest levels. SSTs in the Thames River Estuary
during the months preceding the study were below average for most of the winter,
Northeastern Naturalist
414
L.S. Vlietstra
2014 Vol. 21, No. 3
on some days dropping to 0–4 °C, temperatures at which Atlantic Comb Jellies do
not survive (Costello et al. 2012, Shiganova et al. 2001).
If ctenophore populations in the Thames River Estuary were reduced by low
temperatures during the winter of 2003–2004, time may be required for ctenophores
to disperse into the estuary from surrounding areas. Such source–sink dynamics
appear to influence the timing of Atlantic Comb Jelly population growth and abundance
in nearby Narragansett Bay, RI (Beaulieu et al. 2013, Costello et al. 2006).
Ctenophores there overwinter in inshore areas, where water warms rapidly in the
spring, favoring early reproduction. Such sites serve as source regions, supplying
adults to cooler regions of the bay where advective currents are stronger and ctenophore
densities are reduced. For comparison, the annual range of SST in the Thames
River Estuary in 2004 (1–24 °C) more closely resembled temperatures in sink regions
of Narragansett Bay during 2003 (Fox Island, Dutch Island: 2–24 °C) than
they did temperatures in the source region (Greenwich Cove: -1–27 °C; Costello et
al. 2006). I did not measure tidal currents in this study, but ctenophore abundance
could be further inhibited if strong currents in the spring flushed individuals out of
the estuary and into Long Island Sound.
In addition to the Atlantic Comb Jelly bloom in early July, a smaller abundance
peak occurred on 5 September 2004. Ctenophore blooms during August–October
were typical in New England prior to the mid-1980s (Sullivan et al. 2001), and
blooms are still observed during this time in the central waters of Narragansett Bay,
RI (Beaulieu et al. 2013). It is possible that local biophysical processes independent
of large-scale currents continue to operate in the Thames River Estuary leading
to a fall spike in ctenophore production. For example, a weakened thermocline in
autumn coupled with wind mixing may bring nutrients to surface waters, promoting
primary production and energy transfer to organisms at higher trophic levels,
including copepods, which, in turn, are consumed by ctenophores. This idea is
consistent with the observation that the water-column stability index (E) decreased
in late August and September 2004, indicating intermittent mixing of the water
column, while copepod and Atlantic Comb Jelly densities increased. In addition,
preliminary results from research conducted at the study site in 2003 and 2011
indicate that surface concentrations of chlorophyll-a and net primary production
peaked during mid-August and early September (S. Wainright, US Coast Guard
Academy, New London, CT, unpubl. data).
Seasonal abundance of larval ctenophores followed a different pattern than that
of adults. Larval densities were variable during the study, with exceptionally high
densities on 28 October 2004. At that time, SST was around 13 °C, dropping to <10
°C within two weeks. Sullivan et al. (2001) also reported a marked pulse in larval
ctenophore density during October in Narragansett Bay, RI. Because the pulse in
larval abundance occured just before water temperatures dropped below levels required
to sustain population growth, the reason for an autumn pulse in ctenophore
reproduction is unclear. One possibility is that reproduction during late autumn is an
adaptive strategy for ensuring genetic representation in overwintering populations
that inhabit shallow areas in estuarine habitats. Overwintering populations may be
Northeastern Naturalist Vol. 21, No. 3
L.S. Vlietstra
2014
415
an important source of individuals initiating production in the spring (Costello et
al. 2006).
Potential Ecological Impacts
Concern over early-summer Atlantic Comb Jelly blooms in New England
largely involves the potential for ctenophores to reduce fish production by consuming
large amounts of ichthyoplankton and outcompeting young fish for copepod
prey (Purcell et al. 2001). Such potential is clearly evident in Chesapeake Bay, VA,
where Atlantic Comb Jelly blooms coincide with spawning in Anchoa mitchilli Valenciennes
(Bay Anchovies ) and where ctenophores consume 20–100% of the eggs
and larvae produced (Cowen and Houde 1993, Purcell et al. 2001). Using similar
calculations based on laboratory-derived consumption rates (Monteleone and Duguay
1988) and observed ctenophore densities (300 individuals m-3), Sullivan et al.
(2001) estimated that in some parts of Narragansett Bay, RI, Atlantic Comb Jellies
consume as much as 111–1500% of fish eggs present. Where ctenophore densities
were lower (7.5 individuals m-3), consumptions rates ranged between 2.5 and 35%
of eggs present per day. However, we found no evidence in the Thames River Estuary
to suggest that Atlantic Comb Jellies were a major consumer of icthyoplankton
during 2004. Our net tows yielded fish eggs only occasionally during May-August,
and our May-June samples contained fish larvae at least 4–8 weeks before adult Atlantic
Comb Jelly became locally abundant. In addition, I found no ichthyoplankton
in Atlantic Comb Jelly gut contents.
In contrast, copepods were an important food source for Atlantic Comb Jelly in
the Thames River Estuary during 2004, having been consumed by 87% of ctenophores
examined. Yet it remains unclear whether ctenophore predation depressed
copepod populations to an appreciable extent. The inverse correlation between
copepod density and ctenophore biovolume suggests that ctenophores could reduce
prey availability to other consumers, such as local fishes. However, research by
Kremer (1979) serves to emphasize that correlations may not indicate cause-effect
relationships. Specifically, Kremer (1979) observed a similar correlation but calculated
that during the 1970s, ctenophores in Narragansett Bay, RI, consumed 5–10%
of the copepod standing stock per day. This level of consumption could not account
for the 20–25% day-1 copepod decline observed in late summer. Instead, predation
pressure from other estuarine consumers, such as fish and other zooplankton, and
possibly reduced phytoplankton concentrations were also thought to play a role in
the decline (Kremer 1979).
In the Thames River Estuary in 2004, my calculations suggest that ctenophores
also consumed a relatively small portion (0.0–2.2%) of copepods on a daily basis.
This figure is much lower than some estimates of copepod consumption by ctenophores
in Narragansett Bay, RI. The findings from a different study from the 1970s
estimated that Atlantic Comb Jellies consumed approximately 20% of the copepod
standing stock per day and noted that ctenophore blooms were followed by rapid
declines in zooplankton (Deason 1982, Deason and Smayda 1982). By the 2000s,
ctenophore densities in Narragansett Bay had increased from earlier decades, and
Northeastern Naturalist
416
L.S. Vlietstra
2014 Vol. 21, No. 3
Atlantic Comb Jellies were considered responsible for drastically reducing the
dominant copepod, Acartia tonsa, during blooms (as cited in Costello et al. 2006).
Although ctenophores in the Thames River Estuary appeared to consume a relatively
small portion of the copepod standing stock in 2004, caution should be used
when drawing conclusions about consumption rates calculated at too fine a resolution.
Recent studies based on ctenophore-gut contents observed in the field suggest
that laboratory-derived feeding rates could underestimate feeding activity in nature
by up to 300–400% (Madsen and Riisgård 2010, Purcell 2009).
One observation supporting the idea that ctenophores had a relatively small impact
on copepod densities in the Thames River Estuary in 2004 is that ctenophore
densities there were much lower than in other estuaries where copepod densities
were comparable but trophic impacts were more pronounced. For example, the
maximum density of adult Atlantic Comb Jellies in the Thames River Estuary was
9.7 individuals m-3, whereas maximum densities in Narragansett Bay, RI during
2002–2003 were 350–450 individuals m-3 (Costello et al. 2006). In Chesapeake
Bay, VA, maximum densities during 2003–2006 were >100 to >400 individuals m-3
(Condon and Steinberg 2008). In addition, copepod densities in the Thames River
Estuary increased over a period of 6 weeks in August–September concurrent with
ctenophore abundance reaching a second annual peak. This pattern suggests that,
while ctenophore predation may have dampened copepod growth rates during this
time, predation pressure was not strong enough to prevent copepods from achieving
positive population growth.
I did not specifically investigate the reasons for low ctenophore densities in
the Thames River Estuary during 2004, but the situation could have involved
several ecological factors, such as prey availability, predation pressure, and water
conditions, including the effects of low winter temperatures previously described
(Costello et al. 2006, Purcell et al. 2001, Purcell and Decker 2005). Perhaps ctenophore
densities were higher and trophic impacts greater in previous or subsequent
years. Comparative studies of ctenophore-population dynamics in the same estuary
in additional years and in estuaries of varying size, hydrographic conditions, and
food-web structure could be useful in identifying factors that influence geographic
variation in ctenophore density, and thus the potential for early ctenophore blooms
to impact local fish production.
In conclusion, early ctenophore blooms in New England and the mid-Atlantic
appear to take place on a relatively large spatial scale, a pattern consistent with
the hypothesis that phenological shifts in ctenophore production are due to largescale
physical processes, such as the NAO, affecting SST along the North Atlantic
coast. However, SST accounted for relatively little seasonal variation in ctenophore
abundance, and further research into both physical and biological factors limiting
the seasonal onset of ctenophore production is needed to better understand reasons
behind seasonal patterns. In the Thames River Estuary, ctenophore densities in
2004 appeared low compared to other, larger estuaries where impacts on organisms
at lower trophic levels, including copepods, appeared more substantial. However,
increases in the abundance of gelatinous consumers concurrent with large-scale
Northeastern Naturalist Vol. 21, No. 3
L.S. Vlietstra
2014
417
climate change have been widely documented in bays, coasts, and oceans worldwide
(Mills 2001, Richardson et al. 2009). If similar increases in ctenophore
biomass develop in small New England estuaries, the ecological impacts of Atlantic
Comb Jelly blooms in the Thames River Estuary may become more pronounced.
Acknowledgments
This study was funded by a grant from the Quebec–Labrador Foundation Atlantic Center
for the Environment and by a postdoctoral teaching fellowship from the United States Coast
Guard Academy (USCGA). I thank the University of Connecticut for granting permission
to use their mooring data, and Laura Gibbons (USCGA ’09) for assisting with field-work. I
am also grateful for assistance with mapping from Sabrina Bateman, and for valuable feedback
on the manuscript provided by Sam Wainright, Mark Patrick, Thomas Trott, and two
anonymous reviewers.
Literature Cited
Beaulieu, W.T., J.H. Costello, G. Klein-Macphee, and B.K. Sullivan. 2013. Seasonality of
the ctenophore Mnemiopsis leidyi in Narragansett Bay, Rhode Island. Journal of Plankton
Research 35:785–791.
Condon, R.H., and D.K. Steinberg. 2008. Development, biological regulation, and fate of
ctenophore blooms in the York River Estuary, Chesapeake Bay. Marine Ecology Progress
Series 369:153–168.
Costello, J.H., B,K. Sullivan, D.J. Gifford, D. van Keuren, and L.J. Sullivan. 2006. Seasonal
refugia, shoreward thermal amplification, and metapopulation dynamics of the
ctenophore, Mnemiopsis leidyi in Narragansett Bay, Rhode Island. Limnology and
Oceanography 51:1819–1831.
Costello, J.H., K.M. Bayha, H.W. Mianzan, T.A. Shiganova, and J.E. Purcell. 2012. Transitions
of Mnemiopsis leidyi (Ctenophore: Lobata) from a native to an exotic species: A
review. Hydrobiologia 690:21–46.
Cowan, J.H., Jr., and E.D. Houde. 1993. Relative predation potential of scyphomedusae,
ctenophores, and planktivorous fish on icthyoplankton in Chesapeake Bay. Marine Ecology
Progress Series 95:55–65.
Deason, E.E. 1982. Mnemiopsis leidyi (Ctenophora) in Narragansett Bay, 1975–1979:
Abundance, size composition and estimation of grazing. Estuarine and Coastal Shelf
Science 15:121–134.
Deason, E.E., and T.J. Smayda. 1982. Ctenophore–zooplankton–phytoplankton interactions
in Narragansett Bay, Rhode Island. Journal of Plankton Research 4:219–236.
Decker, M.B., D.L. Breitburg, and J.E. Purcell. 2004. Effects of low dissolved oxygen on
zooplankton predation by the ctenophore Mnemiopsis leidyi. Marine Ecology Progress
Series 280:163–172.
Granhag, L., L.F. Møller, and L.J. Hansson. 2011. Size-specific clearance rates of the
ctenophore Mnemiopsis leidyi based on in situ gut-content analysis. Journal of Plankton
Research 33:1043–1052.
Hawk, J.D. 1998. The role of the North Atlantic Oscillation in winter climate variability as
it relates to the winter–spring bloom in Narragansett Bay. M.Sc. Thesis. University of
Rhode Island, Kingston, RI. 148 pp.
Knauss, J.A. 1997. Introduction to Physical Oceanography, Second Edition. Prentice Hall,
Inc., NJ. 309 pp.
Northeastern Naturalist
418
L.S. Vlietstra
2014 Vol. 21, No. 3
Kremer, P. 1979. Predation by the ctenophore Mnemiopsis leidyi in Narragansett Bay,
Rhode Island. Estuaries 2:97–105.
Kremer, P. 1994. Patterns of abundance for Mnemiopsis in US coastal waters: A comparative
overview. ICES Journal of Marine Science 51:347–354.
Madsen, C.V., and H.U. Riisgård. 2010. Ingestion-rate method for measurement of clearance
rates of the ctenophore Mnemiopsis leidyi. Aquatic Invasions 5:357–361.
McNamara, M.E., D.J. Lonsdale, and R.M. Cerrato. 2010. Shifting abundance of the
ctenophore Mnemiopsis leidyi and the implications for larval bivalve mortality. Marine
Biology 157:401–412.
Mills, C. 2001. Jellyfish blooms: Are populations increasing globally in response to changing
ocean conditions? Developments in Hydrobiology 155:55–68.
Monteleon, D.M., and L.E. Duguay. 1988. Laboratory studies of predation by the ctenophore
Mnemiopsis leidyi on the early stages in the life history of the Bay Anchovy,
Anchoa mitchilli. Journal of Plankton Research 10:359–372.
Ottersen, G., B. Planque, A. Belgrano, E. Post, P.C. Reid, and N.C. Stenseth. 2001. Ecological
effects of the North Atlantic Oscillation. Oecologia 128:1–14.
Purcell, J.E. 2009. Extension of methods for jellyfish and ctenophore trophic ecology to
large-scale research. Hydrobiologia 616:23–50.
Purcell, J.E., and M.B. Decker. 2005. Effects of climate on relative predation by scyphomedusae
and ctenophores on copepods in Chesapeake Bay during 1987–2000. Limnology
and Oceanography 50:376–387.
Purcell, J.E., D.A. Nemazie, S.E. Dorsey, E.D. Houde, and J.C. Gamble. 1994. Predation
mortality of Bay Anchovy, Anchoa mitchilli, eggs and larvae due to scyphomedusae and
ctenophores in Chesapeake Bay. Marine Ecology Progress Series 114:47–58.
Purcell, J.E., T.A. Shiganova, M. B. Decker, and E.D. Houde. 2001. The ctenophore Mnemiopsis
in native and exotic habitats: US estuaries versus the Black Sea basin. Hydrobiologia
451:145–176.
Reeve, M.R., and M.A. Walter. 1978. Nutritional ecology of ctenophores: A review of recent
research. Advances in Marine Biology 15:249–287.
Reeve, M.R., M.A. Syms, and P. Kremer. 1989. Growth dynamics of a ctenophore (Mnemiopsis)
in relation to variable food supply. I. Carbon biomass, feeding, egg production,
growth and assimilation efficiency. Journal of Plankton Research 11:535–552.
Richardson, A.J., A. Bakun, G.C. Hays, and M.J. Gibbons. 2009. The jellyfish joyride:
Causes, consequences and management responses to a more gelatinous future. Trends in
Ecology and Evolution 24:312-322.
Shiganova, T.A., Z.A. Mirzoyan, E.A. Studenikina, S.P. Volovik, I. Siokou-Frangou, S. Zervoudaki,
E.D. Christou, A.Y. Skirta, and H.J. Dumont. 2001. Population development
of the invader ctenophore Mnemiopsis leidyi, in the Black Sea and in other seas of the
Mediterranean basin. Marine Biology 139:431–445.
Sullivan, L.J., and J. Gifford. 2004. Diet of the larval ctenophore, Mnemiopsis leidyi A. Aggassiz
(Ctenophora, Lobata). Journal of Plankton Research 26:417–431.
Sullivan, B.K., D. Van Keuren, and M. Clancy. 2001. Timing and size of blooms of the
ctenophore Mnemiopsis leidyi in relation to temperature in Narragansett Bay, RI. Hydrobiologia
451:113–120.
Sullivan, B.K., D. J. Gifford, J.H. Costello, and J.R. Graff. 2008. Narragansett Bay ctenophore–
zooplankton–phytoplankton dynamics in a changing climate. Pp. 485–498, In A.
Desbonnet and B.A. Costa-Pierce (Eds.). Science for Ecosystem-based Management,
Springer, New York, NY. 570 pp.
Waggett, R.J., and L.J. Sullivan. 2006. Feeding efficiency of the larval ctenophore Mnemiopsis
leidyi A. Agassiz (Ctenophora, Lobata). Journal of Plankton Research 28:719–723.