A Case Study of Urban and Peri-urban Mammal
Communities: Implications for the Management of National
Park Service Areas
Adam D. Chupp, Amy M. Roder, Loretta L. Battaglia, and John F. Pagels
Northeastern Naturalist, Volume 20, Issue 4 (2013): 631–654
Full-text pdf (Accessible only to subscribers. To subscribe click here.)
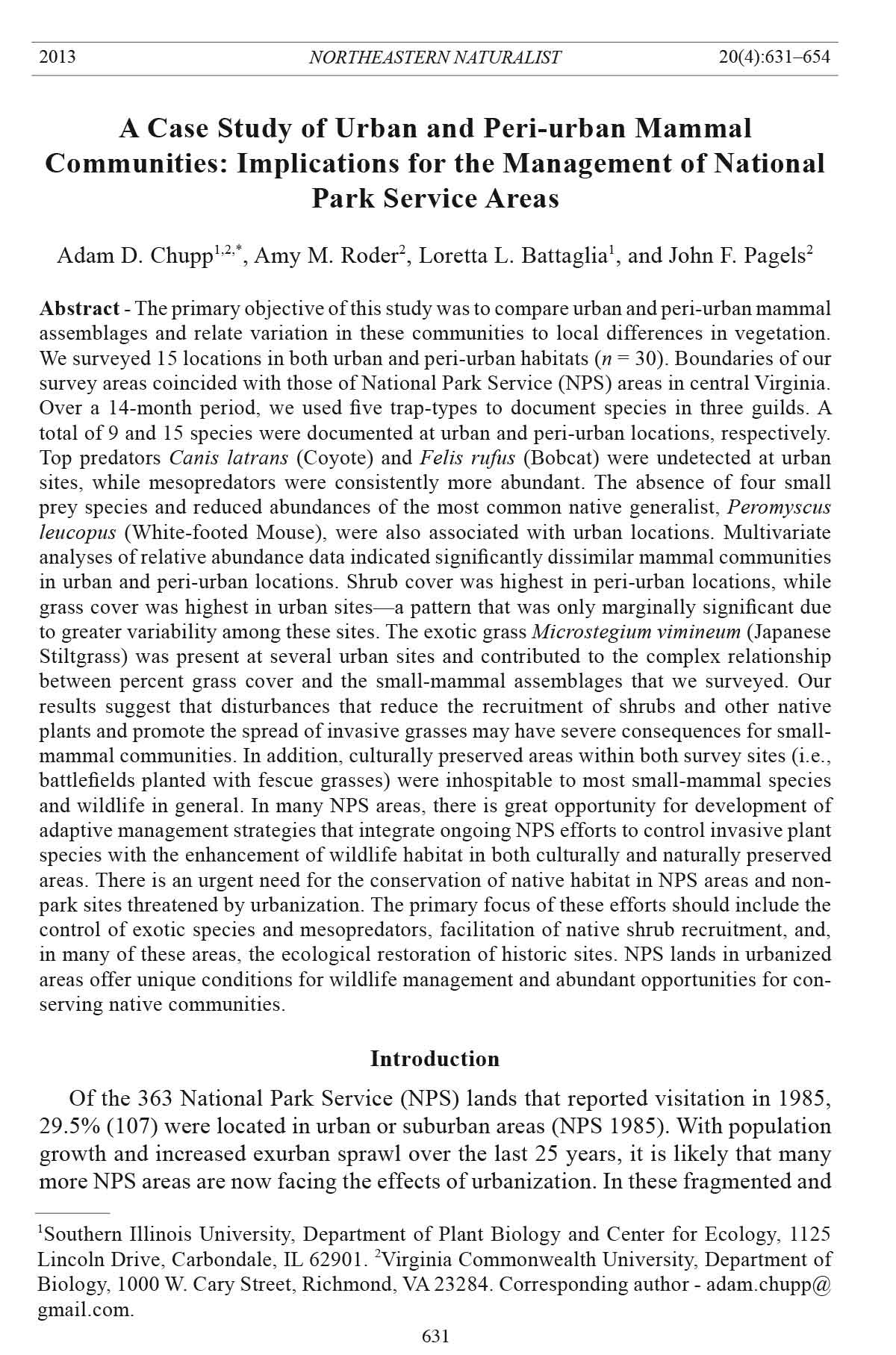
Access Journal Content
Open access browsing of table of contents and abstract pages. Full text pdfs available for download for subscribers.
Current Issue: Vol. 30 (3)
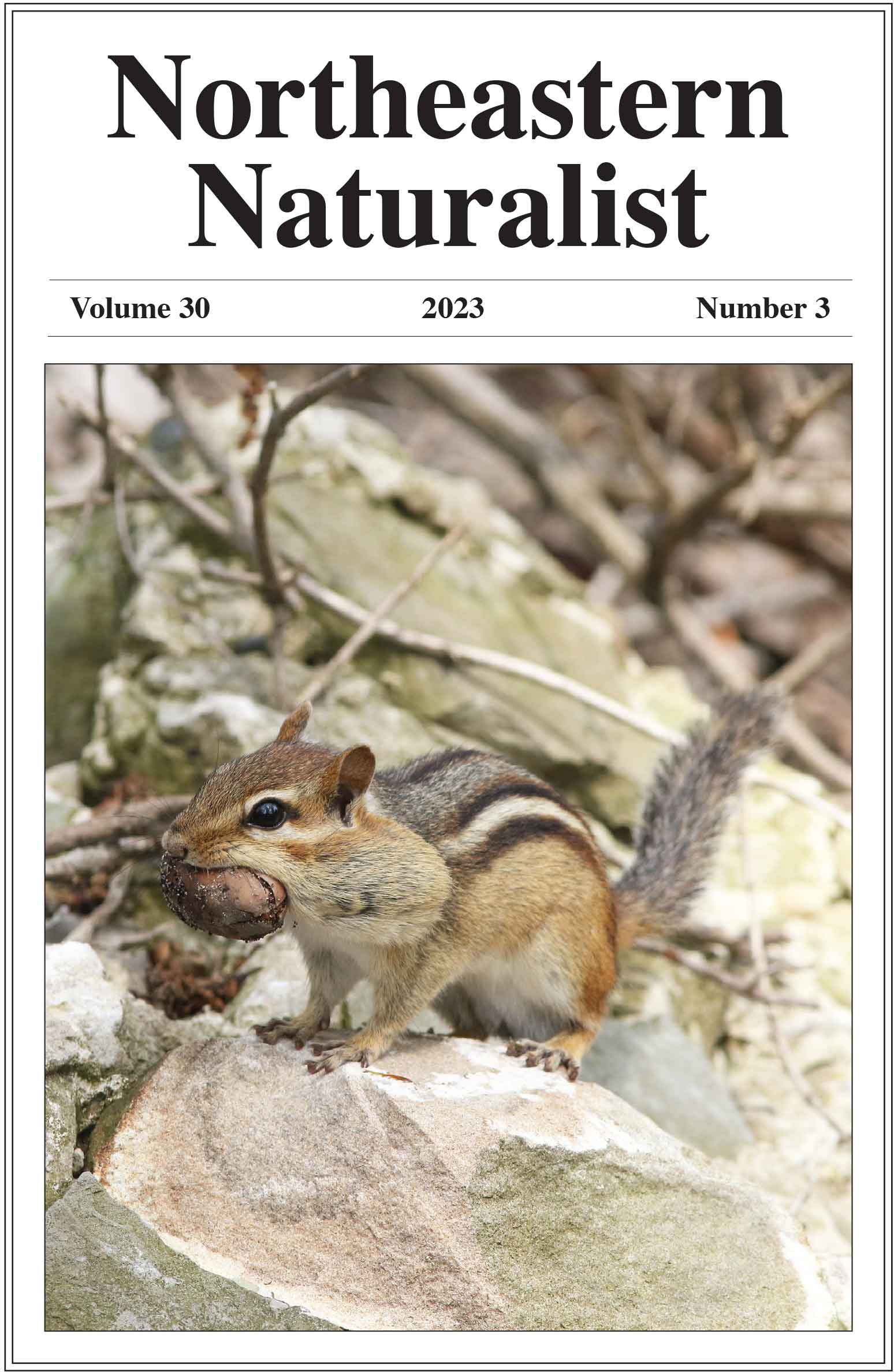
Check out NENA's latest Monograph:
Monograph 22
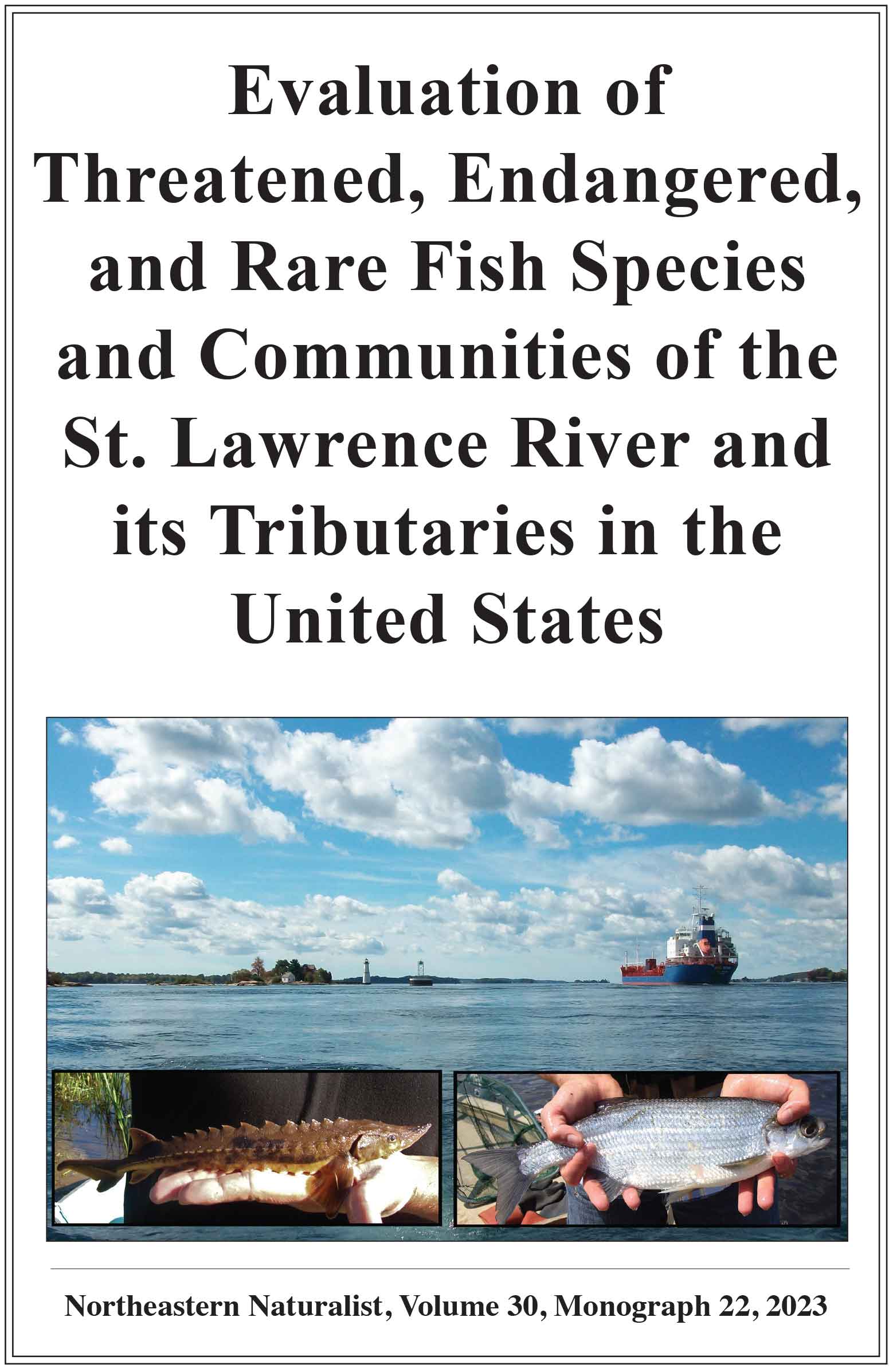
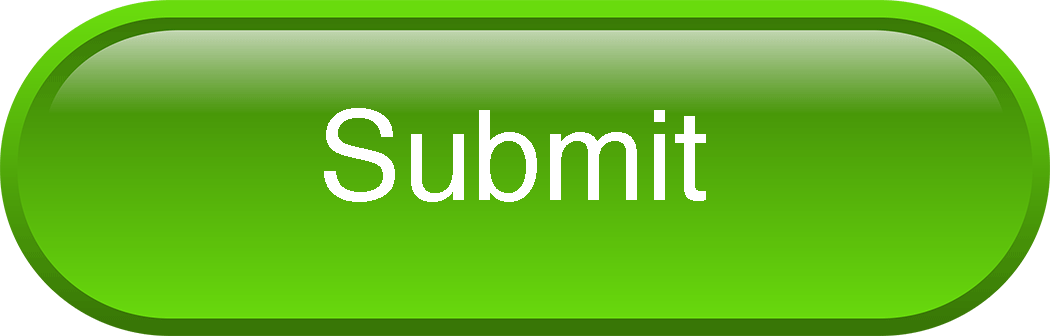
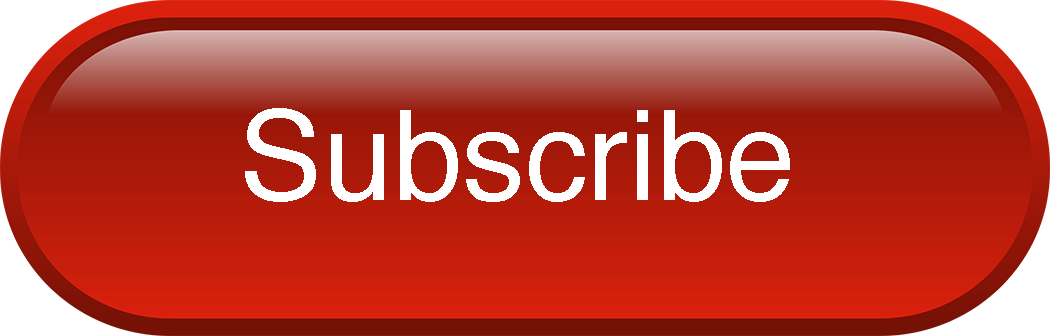
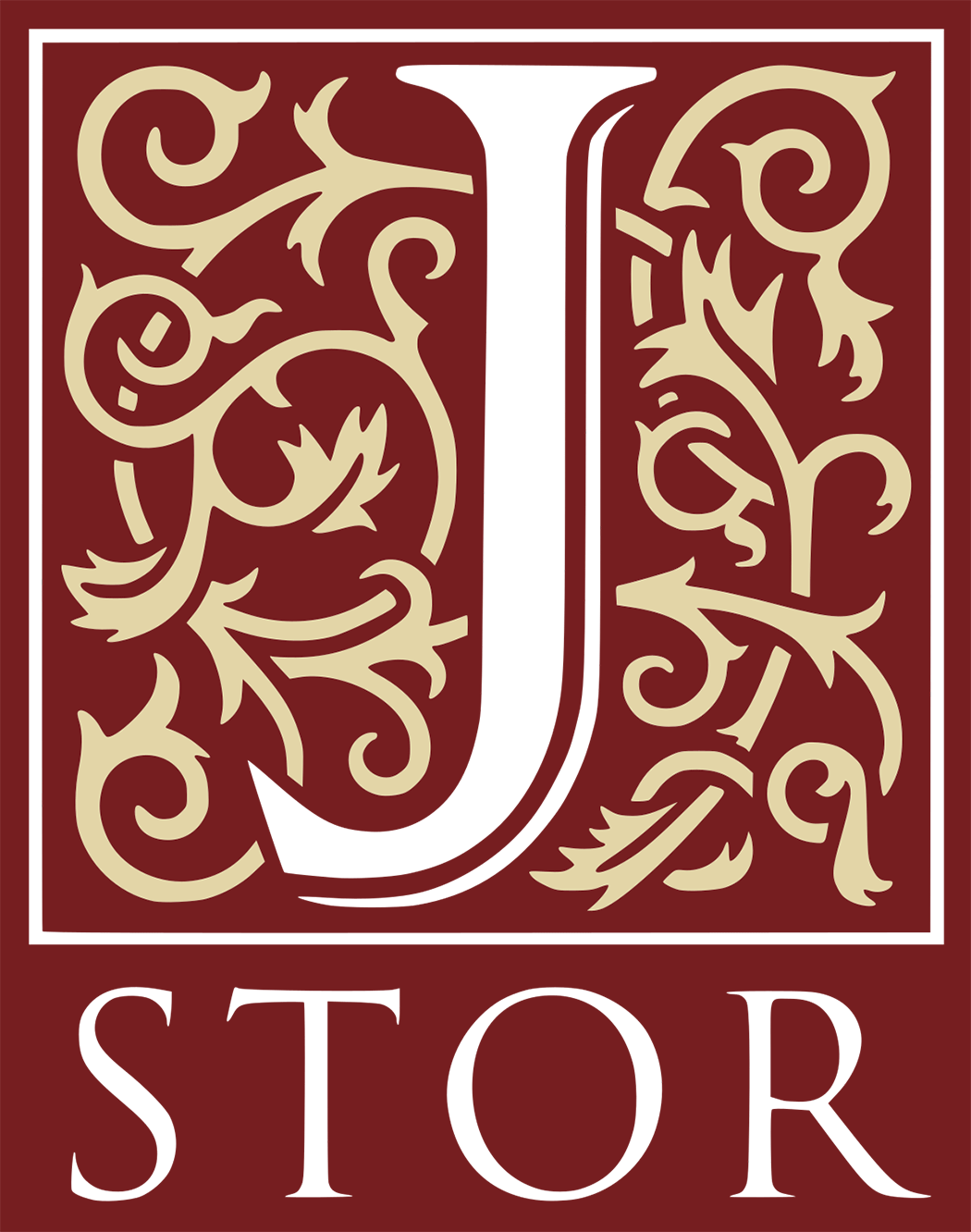


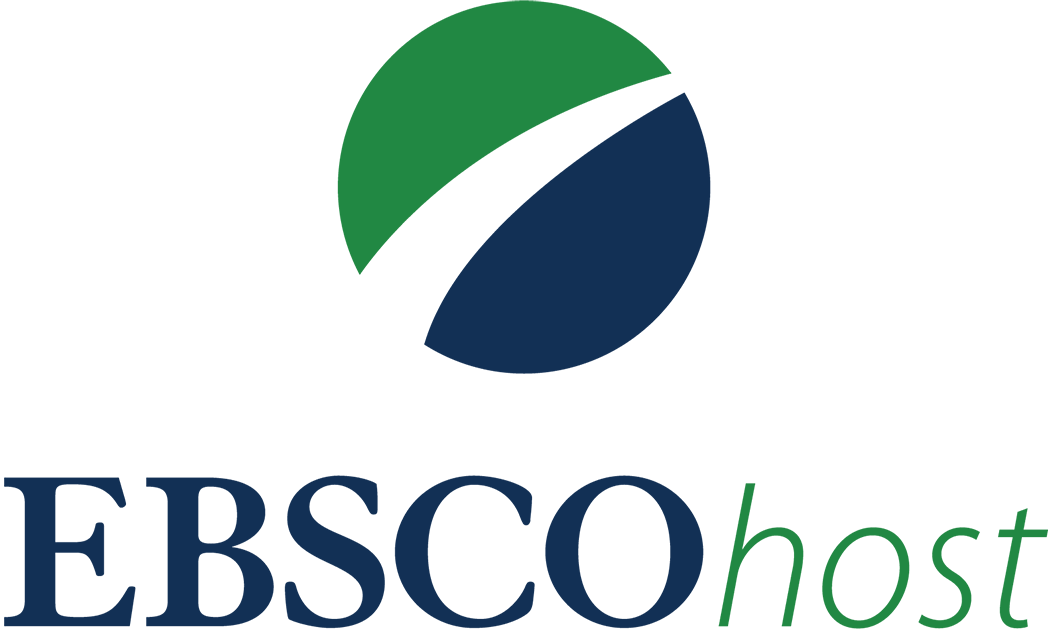

Northeastern Naturalist Vol. 20, No. 4
A.D. Chupp, A.M. Roder, L.L. Battaglia, and J.F. Pagels
2013
631
2013 NORTHEASTERN NATURALIST 20(4):631–654
A Case Study of Urban and Peri-urban Mammal
Communities: Implications for the Management of National
Park Service Areas
Adam D. Chupp1,2,*, Amy M. Roder2, Loretta L. Battaglia1, and John F. Pagels2
Abstract - The primary objective of this study was to compare urban and peri-urban mammal
assemblages and relate variation in these communities to local differences in vegetation.
We surveyed 15 locations in both urban and peri-urban habitats (n = 30). Boundaries of our
survey areas coincided with those of National Park Service (NPS) areas in central Virginia.
Over a 14-month period, we used five trap-types to document species in three guilds. A
total of 9 and 15 species were documented at urban and peri-urban locations, respectively.
Top predators Canis latrans (Coyote) and Felis rufus (Bobcat) were undetected at urban
sites, while mesopredators were consistently more abundant. The absence of four small
prey species and reduced abundances of the most common native generalist, Peromyscus
leucopus (White-footed Mouse), were also associated with urban locations. Multivariate
analyses of relative abundance data indicated significantly dissimilar mammal communities
in urban and peri-urban locations. Shrub cover was highest in peri-urban locations, while
grass cover was highest in urban sites—a pattern that was only marginally significant due
to greater variability among these sites. The exotic grass Microstegium vimineum (Japanese
Stiltgrass) was present at several urban sites and contributed to the complex relationship
between percent grass cover and the small-mammal assemblages that we surveyed. Our
results suggest that disturbances that reduce the recruitment of shrubs and other native
plants and promote the spread of invasive grasses may have severe consequences for smallmammal
communities. In addition, culturally preserved areas within both survey sites (i.e.,
battlefields planted with fescue grasses) were inhospitable to most small-mammal species
and wildlife in general. In many NPS areas, there is great opportunity for development of
adaptive management strategies that integrate ongoing NPS efforts to control invasive plant
species with the enhancement of wildlife habitat in both culturally and naturally preserved
areas. There is an urgent need for the conservation of native habitat in NPS areas and nonpark
sites threatened by urbanization. The primary focus of these efforts should include the
control of exotic species and mesopredators, facilitation of native shrub recruitment, and,
in many of these areas, the ecological restoration of historic sites. NPS lands in urbanized
areas offer unique conditions for wildlife management and abundant opportunities for conserving
native communities.
Introduction
Of the 363 National Park Service (NPS) lands that reported visitation in 1985,
29.5% (107) were located in urban or suburban areas (NPS 1985). With population
growth and increased exurban sprawl over the last 25 years, it is likely that many
more NPS areas are now facing the effects of urbanization. In these fragmented and
1Southern Illinois University, Department of Plant Biology and Center for Ecology, 1125
Lincoln Drive, Carbondale, IL 62901. 2Virginia Commonwealth University, Department of
Biology, 1000 W. Cary Street, Richmond, VA 23284. Corresponding author - adam.chupp@
gmail.com.
632
A.D. Chupp, A.M. Roder, L.L. Battaglia, and J.F. Pagels
2013 Northeastern Naturalist Vol. 20, No. 4
degraded landscapes, small parks often contain remnants of natural habitats that
provide refugia and corridors that are vital to biodiversity conservation (Falkner
and Stohlgren 1997, Ferguson et al. 2001). In fact, small national parks and other
managed areas may support a disproportionately large portion of the regional species
pool (Falkner and Stohlgren 1997). However, in fragmented urban or suburban
landscapes, the continuing effects of land-use modification and other human activities
often threaten the ecological value of these protected areas.
On remnant patches in fragmented landscapes, native small-mammal species
often persist because of their small home ranges (Mahan and O’Connell 2005).
However, these species respond to local variation in microhabitat structure (e.g.,
vertical vegetation structure, volume of coarse woody debris, canopy openness,
and percent grass-cover), affecting the survival of individuals and the dynamics of
local populations (Bellows et al. 2001; Bowman et al. 2000; Clark et al. 1987; Dickman
1987; Dickman and Doncaster 1987; Garden et al. 2007; Kaufman et al. 1983,
1985; Manning and Edge 2004; Washburn et al. 2000). Disturbances that alter the
structure of native habitats may reduce species richness and result in compositional
shifts toward more generalist species (DeStefano and DeGraaf 2003; Donnelly
and Marzluff 2004; McKinney 2002a, 2006; Nilon and VanDruff 1987). The invasion
of exotic generalist species (e.g., Mus musculus L. [House Mouse] and Rattus
norvegicus Berkenhout [Norway Rat]), and associated reductions in abundance of
native generalists (e.g., Peromyscus leucopus Rafinesque [White-footed Mouse])
may be indicative of highly disturbed areas (Huckstep 1996, Nilon and VanDruff
1987). Such compositional shifts have been correlated with increases in bare soil,
tree-canopy loss, and litter and other anthropogenic refuse (Huckstep 1996, Nilon
and VanDruff 1987, VanDruff and Rowse 1986).
In urban environments, many human activities may directly or indirectly disturb
the structure of native habitat. For example, the spread of exotic grasses, which is
facilitated by the presence of edge habitat, corridors for dispersal (e.g., roadways),
heavy visitation/tourism, and ecologically unsound management, may homogenize
local plant communities and degrade native habitat (Cilliers et al. 2008; Ehrenfeld
et al. 2001; Lesica et al. 1993; Lonsdale 1999; McKinney 2002b, 2004, 2006; Tyser
and Worley 1992; Whipple 2001). In addition, the culturally based management of
historic sites may have similar effects. For example, historic battlefields are more
easily maintained as dense mats of cool season grasses such as Festuca spp. (fescue
grasses), but are likely inhospitable to many small vertebrate species (Pagels
et al. 2005a). Such practices may promote novel communities that are detrimental
to biodiversity conservation. Along urban-to-rural gradients, the importance of addressing
these issues is increasing as human disturbances become more persistent
and the structure of our native landscape is more severely degraded. Understanding
compositional trends with respect to local habitat conditions is vital to planning for
and prioritizing wildlife conservation in all types of managed areas (Andren 1994,
Sauvajot et al. 1998).
Whereas small-mammal communities may be affected by local habitat conditions,
larger carnivorous mammals have more expansive home ranges and respond
Northeastern Naturalist Vol. 20, No. 4
A.D. Chupp, A.M. Roder, L.L. Battaglia, and J.F. Pagels
2013
633
to larger scale variations in habitat suitability. Populations of large mammalian
predators may respond negatively to any disturbance that reduces patch connectivity
and increases the likelihood of encountering humans (Crooks 2002, Noss et al.
1996, Tigas et al. 2002, Woodroffe and Ginsberg 1998). While top predators may
persist in urban areas (e.g., Tigas et al. 2002), declining numbers of large carnivores
in remnant patches may have cascading effects on multiple trophic levels.
Mesopredator-release theory suggests that mesopredators (i.e., medium-sized
carnivores) benefit from the absence of larger predators and ultimately reduce the
abundance of smaller species upon which they prey (Soule et al. 1988). However,
like other generalist species, the success of mesopredators in urbanized landscapes
may also result from their ability to take advantage of anthropogenic subsidies
(e.g., human refuse, ornamental fruits and vegetables, and pet food). Carnivores of
all sizes present unique conservation challenges in human-dominated landscapes
(Gehrt et al. 2010).
Small national park areas may be extremely important for maintaining the richness
of regional mammal communities, and in urbanized areas, remnant patches
contained within protected areas should be prioritized for biological conservation
(Croci et al. 2008). Improving the environmental conditions for native flora and
fauna requires specific knowledge of local species assemblages and how they are
affected by specific habitat characteristics. Empirical studies that identify relationships
between local communities and their environment are of broad interest to
managers and biologists who are concerned with maintaining the ecological value
of natural resources and regional species pools.
The primary objective of this study was to compare mammal assemblages and
vegetation characteristics at locations in urban and peri-urban habitat patches (see
next section for definitions of urban and peri-urban). Patches were categorized into
habitat types that were equally replicated in urban and peri-urban areas. Our study
addressed the following questions: (1) Are there differences between the mammal
assemblages of urban and peri-urban locations? (2) If the mammal assemblages
differ, which species and trophic levels (prey species, mesopredators and/or top
predators) account for the differences? (3) Are vegetation characteristics good
predictors of prey-species abundances? (4) Is there a relationship between the
abundances of prey and mesopredator species?
Study Areas
We defined an urban area as one that was located within city limits and included
high-density development with impervious structures (e.g., buildings, sidewalks,
parking lots) and roads with high traffic volume (>100 cars/day) (Forman 1995).
For this study, we established urban locations within the Eastern Front Unit of
Petersburg National Battlefield (Eastern Front). This area is located within the city
limits of Petersburg, VA. Other surrounding metropolitan areas include Colonial
Heights, VA to the northwest and Hopewell, VA and Fort Lee to the northeast
(Figs. 1, 2). This NPS area contains remnant habitat near a center of urbanization
and is located in the vicinity of the Fall Line near the juncture of the Piedmont and
634
A.D. Chupp, A.M. Roder, L.L. Battaglia, and J.F. Pagels
2013 Northeastern Naturalist Vol. 20, No. 4
Figure 1. Map of urban and peri-urban survey areas and the surrounding landscape. Locations
of these areas are shown with respect to the urban-to-rural land-use gradient that
radiates outward from downtown Petersburg, VA. Urban and peri-urban survey areas are
represented by a triangle and a square, respectively. The map was created with ArcGIS 10
software (Esri).
Coastal Plain physiographic provinces. The Eastern Front covers an area of 585 ha
that includes fields of fescue grasses and a mosaic of forested successional habitat
types ranging from pine- to hardwood-dominated forests. Less-developed areas
adjacent to the Eastern Front occur only on the southeastern boundary. As a result
of its proximity to Petersburg, Colonial Heights, Hopewell, and Fort Lee (combined
population ≈80,000), park visitation (≈150,000 visitors/yr), and surrounding commercial
and residential development, the flora and fauna of this NPS area are likely
heavily influenced by the effects of urbanization (see Fig. 2).
We define peri-urban as a rural area adjacent to a city or town (Clergeau et al.
2001, Snep et al. 2006). The peri-urban locations of our survey were established
within the Five Forks Unit of Petersburg National Battlefield (Five Forks) in Dinwiddie
County, 24 km southwest of the urban trapping area (Figs. 1, 3). Five Forks is
also located in close proximity to the Fall Line. However, relative to urban trapping
locations, the peri-urban locations are closer to the rural end of the urban-to-rural
gradient. The area covers 452 ha, and includes mowed fields of fescue grasses and
a variety of successional habitat types ranging from pine- to hardwood-dominated
forests. The peri-urban sites lie within a rural setting that includes agricultural
fields, forests of various ages, and scattered residences that are characteristic of
Northeastern Naturalist Vol. 20, No. 4
A.D. Chupp, A.M. Roder, L.L. Battaglia, and J.F. Pagels
2013
635
much of present-day south-central Virginia. The landscape is less fragmented by
impervious surfaces than our urban site and contains larger contiguous patches of
habitat that overlap with the boundaries of our peri-urban survey area (Figs. 1, 3).
Methods
Trapping locations
Before selecting trapping locations, we first determined the maj or habitat types
(i.e., plant communities) within our urban and peri-urban survey areas. With the
initial help of natural resource managers and orthophotographs, we determined the
available habitat types and scouted possible trapping locations within each. In all, 5
major habitat types were identified in both urban and peri-urban areas: field-forest
Figure 2. Orthophotograph of urban sites located within the city limits of Petersburg, VA.
There are 15 sites total with 3 replicates for each of 5 habitat types: field-forest edge (FFE),
pine-forest plantation (PFP), hardwood (HWD), mixed-hardwood pine (MHP), and bottomland
hardwood (BLHWD). Image was taken in 2006 and used with permission from
the National Park Service.
636
A.D. Chupp, A.M. Roder, L.L. Battaglia, and J.F. Pagels
2013 Northeastern Naturalist Vol. 20, No. 4
edge (FFE), pine-forest plantation (PFP), hardwood (HWD), mixed hardwood-pine
(MHP) and bottomland hardwood (BLHWD). We then established three replicate
trapping locations in each habitat type (total of 15 locations within both survey
areas). We randomly selected trapping locations using a grid (overlain on orthophotographs)
and random number generator. Boundaries of all trapping locations were
at least 300 m apart and >30 m from edge habitat. Stratifying our trapping locations
in this way safeguarded against sampling bias (i.e., incorporation of more habitat
heterogeneity in one area than the other) (Figs. 2, 3).
Field-forest edge (FFE). This habitat type occurred where field maintenance or
mowing created very abrupt or narrow contact areas along field-forest edges. In most
cases, FFE sites were only 1–5 m wide. Vegetation along FFE habitat was typically
a mix of field and forest vegetation, and was much more heterogeneous than that of
either the field or forest habitats. This habitat type contained both coniferous and
Figure 3. Orthophotograph of peri-urban sites located in Dinwiddie County, VA. There are
15 sites total with 3 replicates for each of 5 habitat types: field-forest edge (FFE), pineforest
plantation (PFP), hardwood (HWD), mixed-hardwood pine (MHP), and bottomland
hardwood (BLHWD). Image was taken in 2006 and used with permission from the National
Park Service.
Northeastern Naturalist Vol. 20, No. 4
A.D. Chupp, A.M. Roder, L.L. Battaglia, and J.F. Pagels
2013
637
deciduous species in the overstory, and the understory was comprised of saplings of
overstory species. However, the understory was often dominated by shade-intolerant
pioneer species such as Juniperus virginiana L. (Eastern Red Cedar). Fescue grasses
and other exotic species including Ailanthus altissima Mill. (Tree of Heaven ), Ligustrum
sinense Lour. (Chinese Privet), and Lonicera japonica Thunb. (Japanese
Honeysuckle) were more common here than in other habitats.
Pine forest plantation (PFP). The overstory of this habitat type was dominated
by Pinus taeda L. (Loblolly Pine), and although tree age was not determined, it was
estimated that these stands ranged from 20–25 y in age. The stands we sampled had
begun a natural thinning process, and the understory was beginning to open at most
locations. However, the ground layer still consisted of a thick layer of pine-needle
litter. The understory of this habitat type was dominated by saplings of deciduous
tree species, most commonly Liquidambar styraciflua L. (Sweetgum). Nearly all
understory trees in this habitat type were small (diameter at breast height [DBH;
breast height = 1.5 m] < 10 cm). Although grasses and herbs were relatively uncommon
here, the invasive species Microstegium vimineum Trin. (Japanese Stiltgrass)
was present in urban PFP sampling locations.
Hardwood (HWD). This habitat type was characterized by deciduous species in
the overstory and understory. Overstory species common to both urban and periurban
locations included Sweetgum, Acer rubrum L. (Red Maple), Liriodendron
tulipifera L. (Tulip Poplar), Nyssa sylvatica Marshall (Blackgum), Quercus alba
L. (White Oak), Quercus rubra L. (Northern Red Oak), and Carya spp. (hickory
species). Subcanopy species included Sassafras albidum Nutt. (Sassafras), Carpinus
caroliniana Walter (Ironwood), Ilex opaca Aiton (American Holly), Cornus
florida L. (Dogwood), and Eastern Red Cedar. Understory tree species were mostly
saplings of overstory species. Ground-cover consisted primarily of deciduous leaf
litter. Herbs, grasses, and shrubs were relatively sparse in the HWD habitat type.
Mixed hardwood-pine (MHP). This habitat type included both deciduous and
coniferous species in the overstory and is an intermediate successional stage between
pine and hardwood forest types. Loblolly Pine was the dominant coniferous
species. Pinus virginiana Mill. (Virginia Pine) was also present in this habitat
type. Although deciduous trees were more abundant than conifers in the overstory,
the average DBH of conifers was considerably larger than that of deciduous species.
Hardwood species in this habitat type were a mix of those species found in
the HWD habitat type. Understory tree species were mostly saplings of overstory
hardwood species. Few coniferous saplings were found in the understory. At urban
locations, Japanese Stiltgrass was observed in this habitat type.
Bottomland hardwood (BLHWD). This habitat type was restricted to floodplain
forests associated with streams or rivers. Overstory trees were primarily
deciduous, and typical species included Platanus occidentalis L. (American
Sycamore), Fraxinus pennsylvanica Marshall (Green Ash), and Betula nigra L.
(River Birch). The understory was comprised of saplings of overstory species.
The aromatic shrub Lindera benzoin L. (Spicebush) was also common. Grasses
and herbs were observed in much greater abundance here than in any other habitat
638
A.D. Chupp, A.M. Roder, L.L. Battaglia, and J.F. Pagels
2013 Northeastern Naturalist Vol. 20, No. 4
type. Japanese Stiltgrass (exotic) and Arundinaria gigantea Walter (Switch Cane)
were abundant at urban BLHWD locations. Ferns were also common in this
habitat type. With locations so close to water sources, BLHWD sampling sites had
very moist substrates, and washouts from flooding were not unusual at many of
our BLHWD sampling sites.
Mammal surveys
Seven trapping sessions were partitioned into seasons that occurred between
June 2003 and August 2004. During each session, urban and peri-urban locations
were sampled for the same number of nights during the same two-week time period.
Depending on season, trapping sessions ranged from 2–4 nights of continuous trapping.
A trap-night was subtracted from the effort when a trap was found to be sprung
but unoccupied (modified from Nelson and Clark 1973).
We used a circular-plot sampling scheme for all trapping locations except those
located in field-forest edges. The circular design has been successfully used in
studies focused on mammal population dynamics (Orrock et al. 2000), mammal
communities (Bellows et al. 2001, McShea et al. 2003), endangered species surveys
(Orrock et al. 2000), and determining new records of occurrence (Bellows et
al. 1999). Each trapping plot (i.e., trapping location) consisted of a 30-m-diameter
circle with markers at the center and 15 m from the center in each cardinal direction
so that the plot was divided into four equal quadrants. We used 60-m-long transects
instead of circular plots in the FFE habitat type because patches in this habitat type
were often narrow, and circular plots would have overlapped into other habitat
types. Based on trap type and trap number, the sampling effort on transects was
equivalent to that of the circular plots, but traps were arranged in a linear fashion at
20-m intervals (i.e., points at 0, 20, 40 and 60 m).
At each location, we used a combination of four different trap types that would
allow us to effectively sample for all small prey and mesopredator species. Three
Sherman live traps (7.5 x 9 x 23 cm) were placed at likely capture spots within a
2-m-radius of each cardinal direction marker. Two small Tomahawk live traps (40.6
x 12.7 x 12.7 cm) were placed in opposite quadrants from each other, and one medium-
size Tomahawk live trap (81.3 x 25.4 x 30.5 cm) was placed at or near the center
of the plot. Sherman live traps were baited with an oatmeal-peanut butter mixture
that was wrapped in wax paper and hung from the back door of the trap. Small
amounts of peanut butter were also placed on the open front door. Small Tomahawk
traps were baited with apples covered in peanut butter, and large Tomahawk traps
were baited with apples and sardines.
For more effective sampling of the smallest prey species (i.e., shrews), two pitfall
traps were placed in each of the four quadrants (Kirkland and Sheppard 1994,
Mitchell et al. 1993). Sixteen-ounce (533 ml) beverage cups (i.e., pitfall traps) were
placed in the ground at likely capture spots, i.e., where natural drift fences (logs and
stumps) were found. We filled the bottom 5 cm of each pitfall trap with water, and
we used plastic mesh lids (15 x 15 cm) elevated by nails to shield the pitfall traps
from falling leaves and other debris. Because we observed poor capture success
with shrews in 2003, we added two larger pitfall traps to each site for sampling in
Northeastern Naturalist Vol. 20, No. 4
A.D. Chupp, A.M. Roder, L.L. Battaglia, and J.F. Pagels
2013
639
spring 2004. We made the larger pitfall traps from 2-L plastic soda-bottles with the
tops cut off (after Handley and Varn 1994) and lowered the plastic mesh lids over
all pitfall traps to close them between sampling sessions.
Mammals smaller than or equal to the size of an adult Sigmodon hispidus Say
and Ord (Hispid Cotton Rat; ≈100–225 g) were tagged with Monel ear tags (National
Band and Tag Co., Newport, KY). Mammals larger than or equal to the size
of an adult Sciurus carolinensis Gmelin (Eastern Gray Squirrel; ≈400–600 g) were
marked with non-toxic spray paint and examined for distinguishing features and
approximate age. Both marking techniques allowed us to identify recaptures within
each trapping session. All mammals were released at the location of capture.
Using TrailMaster’s Active Infrared Trail Monitor (Model # TM1550) and Camera
Kit (Model # TM35-1) (TrailMaster Infrared Trail Monitors, Lenexa, KS), we
conducted night-camera photography to document medium to large species (i.e.,
mesopredators and top predators). This method is appropriate for use in mammal
inventories where larger mammals need to be surveyed (Silveira et al. 2003). We
used three camera traps simultaneously. During five trapping sessions, cameras
were active for the same number of nights as the trapping locations. However, camera
traps were not placed near trapping locations and instead were most often in
secluded wooded areas. Locations of camera traps varied among sampling sessions.
We placed cameras in areas most likely to be frequented by medium to large nocturnal
mammals (i.e., game trails and small unpaved roads or walking paths), and
where vegetation and topography would not trigger the trail monitors. We situated
trail monitors at a height of approximately 0.25–0.5 m and set them for a 2-minute
delay after each photograph. These camera sites were active from dusk to dawn and
were baited with sardines, peanut butter, apples, and raw chicken.
Vegetation surveys
Within each trapping location, DBH was recorded for all trees, defined as woody
plants with a DBH ≥5 cm. For transect-style trapping locations, any tree less than
6 m from the transect line was considered to be within the trapping location; therefore,
the total area sampled was nearly equivalent to that of circular plots. All trees
were identified to species, with a few exceptions when we were only able to assign a
genus. Groundcover was quantified using the line-transect method (Canfield 1941).
For circular trapping locations, we established two 40-m transects that crossed in
the plot center to divide the trapping location into four equal quarters. For transectstyle
trapping locations, we extended the same transect line established for mammal
sampling (60 m) by 20 m and used it to quantify groundcover. Using the line-transect
method, we recorded observations at 1-m intervals in the following categories:
grass, non-grass herbaceous, shrub (woody plants <5 cm DBH), and downed wood.
Downed wood was considered to be any portion of a woody stem or trunk >1 m in
length. We recorded the diameter of any intercepted piece of downed wood that had
a DBH ≥4 cm (i.e., coarse woody debris).
Data treatment and statistical analyses
For each trapping location, we calculated the relative abundance of prey and
640
A.D. Chupp, A.M. Roder, L.L. Battaglia, and J.F. Pagels
2013 Northeastern Naturalist Vol. 20, No. 4
mesopredator species as the total number of unique individuals captured (Mt+1,
excludes recaptures) divided by the total number of trap-nights, for traps in which
a species could be captured during that trapping session (Slade and Blair 2000).
The relative abundance of each species captured was expressed per 100 trap-nights
and averaged across all 7 trapping sessions for each trapping location; species
abundances at each trapping location are expressed as mean relative abundances.
Abundance estimated using Mt+1 is an index of population size because the number
of individuals captured is a function of population size as well as the likelihood that
an individual will be captured (Pollock et al. 2002, Slade and Blair 2000). Mt+1 was
used because it performs as well as an estimator that incorporates capture probability
(e.g., the Lincoln-Petersen estimator) when captures are low (Slade and Blair
2000), as was the case in this study and other short-term surveys. Although capture
probability for a single species may vary depending upon habitat type, season, or
trap type (Pollock et al. 2002), our trapping effort was designed to eliminate variation
in these factors between survey areas (urban vs. peri-urban) and among habitats
(FFE, PFP, MHP, HWD, BLHWD).
We used two-way ANOVA to test for effects of habitat type and landscape type
(urban vs. peri-urban) on the mean relative abundances of species captured. In
addition, we compared mammal species abundances among habitats within each
landscape type using one-way ANOVA. We calculated the Shannon-Weiner index
of diversity for each trapping location using the mean relative abundances of
mesopredator and small-prey species and compared diversity between urban and
peri-urban trapping locations using an independent samples t-test. Diversity of
small-prey species alone was similarly compared. All univariate tests were conducted
using SAS 9.2 (SAS Institute 2007).
Across all locations, trends in mammal community composition (excluding top
predators) were examined using non-metric multidimensional scaling ordination
(NMDS, McCune and Grace 2002); we used Bray-Curtis dissimilarities, based on
the mean relative abundances of species captured, to generate the ordination. We
also performed an NMDS ordination using only the mean relative abundances of
small prey species (excluding mesopredators and top predators). We used analysis
of similarity (ANOSIM, Clarke 1993) to test for significant differences between urban
and peri-urban communities. The NMDS and ANOSIM were conducted using
the DECODA software package (Minchin 1989).
We also conducted an indicator species analysis to identify species indicative
of either landscape type (PC-ORD; McCune and Mefford 1999). For trapping locations
within each landscape type, we calculated indicator values from the mean
relative abundance and/or relative frequency of occurrence for individual species.
Faithful occurrence and/or concentrated abundance within a single group of sample
units (i.e., urban or peri-urban) produce a higher indicator value for that species
(Dufrene and Legendre 1997, McCune and Grace 2002).
We used the number of night photographs as an estimate of relative abundance
for species of mesopredator size or larger within each survey area. This was the sole
indicator of top predator abundance, and a supplemental measure for the abundance
Northeastern Naturalist Vol. 20, No. 4
A.D. Chupp, A.M. Roder, L.L. Battaglia, and J.F. Pagels
2013
641
of mesopredators. Because individuals could not be discerned among night-camera
photographs, we used the proportion of each species represented in photographs
as estimates of relative abundance. Abundance estimates from camera traps were
summarized but not used in any statistical analysis.
We calculated the percent cover of grasses, non-grass herbaceous plants, shrubs,
and woody debris from line intercept data for each location. We also calculated the
volume of coarse woody debris (after Van Wagner 1968, Warren and Olsen 1964)
and basal area for all tree species (genus in some cases) at each site. All vegetation
data were square-root transformed to satisfy statistical assumptions. We used
two-way ANOVA to test for differences in vegetation variables with respect to
landscape and habitat type. We used one-way ANOVA to test for significant differences
in vegetation among habitat types within each landscape type.
We used vector fitting to test whether trends in the NMDS ordinations of
mammal assemblages were significantly correlated with vegetation variables
(vectors). For ordinations using only small-prey species abundances, the relative
abundances of mesopredator species were also used as vectors. Vector fitting was
conducted using the DECODA software package (Minchin 1989). Finally, for
species with mean relative abundances that differed significantly between landscape
types and/or among habitat types, we conducted regressions of their mean
relative abundance against each vegetation variable. In addition, the mean relative
abundances of prey species were regressed against the mean relative abundances
of mesopredator species. We conducted all regressions using SAS version
9.2 (SAS Institute 2007). For all analyses, mean abundance data were square-root
transformed to meet statistical assumptions.
Results
Across 7 seasonal trapping sessions (21 nights), we recorded a total of 7352
and 7596 trap-nights at urban and peri-urban trapping locations, respectively
(Table 1). The small discrepancy in trap nights between the two focal areas is
due to a greater number of sprung traps at urban trapping locations. Sprung traps
were most likely the result of tampering by mesopredator species. Excluding
results from camera trapping, a total of 145 unique individuals (excluding recaptures),
representing 8 species, was captured at urban sites (Table 2); five species
were small prey and 3 were mesopredator species. The White-footed Mouse was
the most abundant species (32% of individuals captured), followed by Procyon
Table 1. Number of trap nights per trap type at trapping locations within each landscape type.
Urban Peri-urban
Pitfall 3013 3030
Sherman 3416 3603
Small Tomahawk 584 613
Medium Tomahawk 297 308
Camera 42 42
Total 7352 7596
642
A.D. Chupp, A.M. Roder, L.L. Battaglia, and J.F. Pagels
2013 Northeastern Naturalist Vol. 20, No. 4
Table 2. Number of captures (includes recaptures) per trap type of all mammalian species captured
at trapping locations in the urban and peri-urban survey areas (camera captures = number of photographs).
The number of unique individuals captured (excludes recaptures) is in parentheses. SM =
small prey, MP = mesopredator, and TP = top predator.
Tomahawk
Guild Pitfall Sherman Small Medium Camera
Urban
Peromyscus leucopus SP 118 (47)
Blarina carolinensis SP 14 (14) 11 (11)
Sigmodon hispidus SP 13 (10)
Tamias striatus L. SP 1 (1)
(Eastern Chipmunk)
Sciurus carolinensis SP 4 (3)
Procyon lotor MP 35 (35) 89
Didelphis virginiana MP 3 (3) 16 (16) 11
Felis catus MP 6 (5) 1
Urocyon cinereoargenteus MP 89
Peri-urban
Peromyscus leucopus SP 3 (3) 274 (120)
Blarina carolinensis SP 6 (6) 13 (13)
Sigmodon hispidus SP 1 (1) 1 (1)
Oryzomys palustris SP 13 (10)
Sorex longirostris SP 11 (11) 2 (2)
Sorex hoyi SP 1 (1)
Microtus pinetorum SP 1 (1) 2 (2)
Mus musculus L. SP 1 (1)
(House Mouse)
Ochrotomys nuttalli SP 1 (1) 5 (5)
Didelphis virginiana MP 1 (1) 9 (9) 25
Mephitis mephitis Schreber MP 1 (1) 1 (1)
(Striped Skunk)
Felis catus MP 1 (1)
Procyon lotor MP 2 (2) 5
Felis rufus TP 1
Canis latrans TP 5
lotor L. (Raccoon: 24%), Blarina carolinensis Bachman (Southern Short-tailed
Shrew: 17%), and Didelphis virginiana Kerr (Virginia Opossum: 13%). A total
of 193 individuals, representing 13 species, was captured at peri-urban trapping
locations; 9 species were small prey and the remaining 4 were mesopredators
(Table 2). The White-footed Mouse was again the most abundant species (64% of
individuals captured), followed by the Southern Short-tailed Shrew (10%), and
Sorex longirostris Bachman (Southeastern Shrew: 7%). Five small-mammal species
were captured at peri-urban trapping locations that were not captured at urban
sites: Sorex hoyi Baird (Pygmy Shrew), Southeastern Shrew, Microtus pinetorum
LeConte (Pine Vole), Ochrotomys nuttalli Harlan (Golden Mouse), and Oryzomys
palustris Harlan (Marsh Rice Rat). Based on the mean relative abundances
of small prey and mesopredator species, the average Shannon-Weiner indices
Northeastern Naturalist Vol. 20, No. 4
A.D. Chupp, A.M. Roder, L.L. Battaglia, and J.F. Pagels
2013
643
(± standard error) for urban and peri-urban trapping locations were 0.82 ± 0.11
and 0.76 ± 0.11, respectively. Using only the relative abundances of prey species,
average indices were 0.46 ± 0.10 (urban) and 0.50 ± 0.08 (peri-urban). In both
cases, an independent samples t-test verified that differences between urban and
peri-urban trapping locations were non-significant (P > 0.05).
There was a total effort of 42 camera trap-nights within the boundaries of each
survey area (Table 1). Photographs from urban camera traps indicated an abundance
of Raccoon and Urocyon cinereoargenteus Schreber (Gray Fox). Each species was
recorded on 89 photographs, and combined represented 94% of the photographs
taken within this survey area. The remaining 6% of photographs identified Felis
catus L. (Feral Cat) and Virginia Opossum (Table 2). Peri-urban camera traps took
36 photographs, revealing the presence of Virginia Opossum (69% of photos), Raccoon
(14%), Canis latrans Say (Coyote: 14%), and Felis rufus Schreber (Bobcat:
3%). Five photographs of Coyote and one photograph of a Bobcat documented
the presence of these top predators on the peri-urban landscape. Camera-trapping
documented one additional species at urban sites (Gray Fox) and two additional
species at peri-urban sites (Bobcat and Coyote) that other trap types failed to capture
(Table 2).
Two-way ANOVA results indicated that White-footed Mouse and Raccoon
abundances differed significantly between urban and peri-urban landscape types.
The White-footed Mouse was significantly more abundant (F1,20 = 23.62, P <
0.0001) at peri-urban (mean = 5.21 ± 0.78) versus urban (mean =1.62 ± 0.28) trapping
locations. In contrast, the Raccoon was significantly more abundant (F1,20 =
39.73, P < 0.0001) at urban (mean = 12.02 ± 2.65) versus peri-urban (mean = 1.43
± 1.03) sites. Indicator-species analysis suggested that the White-footed Mouse,
Southeastern Shrew, and Raccoon were good indicator species. The White-footed
Mouse and Southeastern Shrew were significant indicators of peri-urban locations
(IV = 76.3, P = 0.001; IV = 46.7, P = 0.007) and the Raccoon was indicative of
urban communities (IV = 83.4, P = 0.001). Between landscape types, differences
in the relative abundance of all other species were non-signific ant (P > 0.05).
Two-way ANOVA results suggested the Southern Short-tailed Shrew (captured
only at peri-urban sites) was the only prey species with significant differences in
mean relative abundance among habitat types (F1,20 = 4.94, P = 0.0062). Abundance
of this species was higher in MHP (mean = 0.67 ± 0.17) relative to HWD (mean
= 0.12 ± 0.07) and PFP (mean = 0.06 ± 0.04), and significantly higher in BLHWD
(mean = 0.46 ± 0.07) relative to PFP. Results from one-way ANOVA confirmed that
across peri-urban sites, the mean relative abundance of the Southern Short-tailed
Shrew was significantly higher (F4,10 = 10.03, P = 0.0016) in MHP (mean = 0.73 ±
0.20) and BLHWD (mean = 0.45 ± 0.15). On the urban landscape, the Raccoon was
significantly more abundant (F4,10 = 5.87, P = 0.0107) in FFE (mean = 18.06 ± 7.73)
and BLHWD (mean = 24.20 ± 4.03). No other species showed significant differences
among habitat types across or within landscape types. In addition, there were
no interactive effects between landscape and habitat type that were significantly
related to differences in species abundances.
644
A.D. Chupp, A.M. Roder, L.L. Battaglia, and J.F. Pagels
2013 Northeastern Naturalist Vol. 20, No. 4
Two-way ANOVA suggested percent shrub-cover was significantly higher
(F1,20 = 37.20, P < 0.0001) at peri-urban locations (mean = 32.1 ± 5.3) compared
to urban sites (mean = 6.0 ± 1.9). However, there were no significant patterns in
shrub cover with respect to habitat type. Although percent grass-cover was higher
in urban (mean = 39.3 ± 10.6) versus peri-urban sites (mean = 22.2 ± 7.1), the
differences were not statistically significant (F1,20 = 3.68, P = 0.0694). Results
from one-way and two-way ANOVA suggested that grass cover was significantly
higher in FFE and BLHWD, a trend that was observed across all sites in both
landscape types. The highly invasive Japanese Stiltgrass was found at several
urban trapping locations including BLHWD sites and was frequently observed
throughout the survey area. Across habitat types, other significant trends in vegetation
included a greater coverage of non-grass herbs and less coverage of coarse
woody debris in FFE habitat.
Results from a two-dimensional NMDS ordination of trapping locations indicated
differences between urban and peri-urban sites based on the mean relative
abundances of mesopredator and small prey species (Fig. 4), and analysis of similarity
(ANOSIM) confirmed this trend (R = 0.54, P < 0.0001). Vector fitting suggested
that these dissimilarities were significantly correlated with the aforementioned
Figure 4. Non-metric multi-dimensional scaling (NMDS) ordination of the relative abundance
of mesopredators and small-prey species at 30 trapping locations. Trapping locations
are grouped by the areas within which they are located (urban and peri-urban). Analysis
of similarity (ANOSIM) indicated significant dissimilarities between the two groups (R =
0.54, P < 0.0001). The vector represents the direction through the ordination space that is
significantly correlated with shrub cover.
Northeastern Naturalist Vol. 20, No. 4
A.D. Chupp, A.M. Roder, L.L. Battaglia, and J.F. Pagels
2013
645
differences in the percent cover of shrubs (R = 0.67, P = 0.001), where shrub
cover was significantly higher in peri-urban locations. A two-dimensional NMDS
ordination using only small-prey species abundances suggested a similar pattern
of dissimilarity between urban and peri-urban communities (Fig. 5), a trend that
was again statistically verified by ANOSIM (R = 0.26, P = 0.0002). Results from
vector fitting also suggested that these dissimilarities were significantly correlated
with differences in the percent cover of shrubs (R = 0.65, P = 0.001). In addition,
percent grass-cover was correlated with trends in ordination space (R = 0.47, P =
0.03). However, the direction of the grass vector was not well aligned with dissimilarities
between urban and peri-urban locations. Instead, this vector was more
closely aligned with dissimilarities among urban sites (Fig. 5). Mesopredator species
abundances and all other habitat variables were not correlated with trends in
small-mammal composition and relative abundance (P > 0.05).
The White-footed Mouse and Raccoon were the most abundant prey and mesopredator
species, respectively, captured during our surveys and the only species
whose mean relative abundances differed between urban and peri-urban trapping
Figure 5. Non-metric multi-dimensional scaling (NMDS) ordination of the relative abundance
of mesopredators and small-prey species at 30 trapping locations. Trapping locations
are grouped by the areas within which they are located (urban and peri-urban). Analysis
of similarity (ANOSIM) indicated significant dissimilarities between the two groups (R =
0.26, P = 0.0002). The vectors represent the directions through the ordination space that are
significantly correlated with shrub and grass cover.
646
A.D. Chupp, A.M. Roder, L.L. Battaglia, and J.F. Pagels
2013 Northeastern Naturalist Vol. 20, No. 4
locations. Results of regression analyses show a significant positive and negative
relationship between shrub cover and the relative abundances of White-footed
Mouse and Raccoon, respectively (R2 = 0.37, P = 0.0004; R2 = 0.27, P = 0.003).
Regression analyses failed to detect significant relationships between the relative
abundances of these species and any other vegetation variable or mesopredator
abundances. (P > 0.05).
Discussion
Of the 38 mammalian species with distributions that overlap our survey areas
(Linzey 1998, Webster et al. 1985), 24 and 40% of these species were captured at
urban and peri-urban trapping locations, respectively. These results are consistent
with observations from much smaller urban and peri-urban woodlands where urban
sites accommodated just over half the number of species found at peri-urban locations
(Croci et al. 2008). However, small NPS areas are federally managed, and tend
to be larger than other urban parks and woodlands. Therefore, with proper management
we would expect the mammal assemblages of sites within urban NPS areas
to more closely resemble their more rural counterparts. Nevertheless, we did not
expect large-bodied carnivores, which have extensive home ranges, to be present
on the urban landscape.
During our surveys, camera traps recorded the presence of Coyote and Bobcat
only at peri-urban locations. Although top predators have been observed in
urban habitats (Tigas et al. 2002), our results suggest at the very least a reduced
presence of Coyote and Bobcat within the urban landscape we surveyed. Smaller
patch sizes, reduced connectivity, and the negative consequences of encounters
with humans may all be responsible for reducing populations of top predators
(Crooks 2002, Riley et al. 2006, Tigas et al 2002, Woodroffe and Ginsberg 1998).
In comparison, results from both live- and camera-trapping produced convincing
evidence that three important mesopredator species (Raccoon, Gray Fox, and Feral
Cat) are significantly more abundant at urban sites relative to peri-urban locations.
We suspect that a release from predation is at least partly responsible for this trend
(Soule 1988). However, we also agree that the total effect of mesopredator release
is mediated by the productivity of the system (Elmhagen and Rushton 2007). If
mesopredators can more thoroughly exploit the productivity of a system when
top-down control (i.e., lethal and non-lethal effects of predation) is removed, then
removal of top predators in more productive systems will have a greater positive
effect on the abundance of mesopredators. In urban ecosystems, natural productivity
is subsidized by food from anthropogenic sources (Prange et al. 2004). As such,
omnivorous mesopredators (Raccoon, Gray Fox, and Feral Cat), which are free
from predation and can exploit anthropogenic subsidies, may flourish under these
circumstances (Sinclair et al. 1998, Soule et al. 1988). The Feral Cat is perhaps the
best known example of this phenomenon (Sims et al. 2008). On our urban sites, the
adjacent residential and commercial developments offer numerous anthropogenic
subsidies including refuse from dumpsters and trash cans, pet food, and ornamental
fruits and vegetables. Inside park boundaries, refuse from trash cans and scattered
Northeastern Naturalist Vol. 20, No. 4
A.D. Chupp, A.M. Roder, L.L. Battaglia, and J.F. Pagels
2013
647
randomly by the nearly 150,000 park visitors per year also contributes to the availability
of these subsidies (John Pagels and Adam Chupp, pers. observ.). We argue
that Raccoon, Gray Fox, and Feral Cat are benefiting from the exploitation of these
subsidies and a release from predation. Managers should implement measures
that focus on the control of these species, including educational outreach to local
neighborhoods and businesses that address the need for reducing the availability of
anthropogenic subsidies.
Relationships between mesopredator and prey species abundances were not
evident at any level of analysis and thus we provide no evidence for the cascading
effects of mesopredator release (Soule et al. 1988). In addition, we found no
consistent trends with respect to prey species assemblages and habitat type, although
abundances of the Southeastern Shrew and Southern Short-tailed Shrew
were elevated at MHP sites on the peri-urban landscape. Instead, dissimilarities
between urban and peri-urban small-mammal communities were highly correlated
with shrub cover, which was significantly greater at peri-urban locations. Mean
relative abundance of the White-footed Mouse, an indicator of peri-urban sites,
was consistent with these trends. Although the White-footed Mouse is a ubiquitous
generalist, it has been known to associate with certain habitat characteristics,
specifically vertical vegetative structure (Kaufman et al. 1983, 1985). Other small
mammal species that were unique to peri-urban trapping locations (Pygmy Shrew,
Southeastern Shrew, Pine Vole, Golden Mouse, and Marsh Rice Rat) may also
benefit from increases in percent shrub-cover. For example, greater abundances
of the Golden Mouse, an arboreal nest-builder, and Southeastern Shrew have been
correlated with higher frequencies of shrubs and resultant increases in the vertical
structure of woody vegetation (Bellows et al. 2001, Wagner et al. 2000). In addition,
forest specialists like the Pygmy Shrew and Pine Vole depend on moisture
gradients and spatial heterogeneity that result from more complex assemblages of
understory vegetation (Bellows et al. 2001). Furthermore, shrubs may reduce the
possibility of detection from predators by increasing overhead cover and decreasing
the density of herbaceous vegetation, which may hinder movements of small mammals
and increase detection by acoustically oriented predators (Kotler 1984, Rice
1982, Simonetti 1989).
Percent grass cover was well aligned with dissimilarities in small-prey species
communities, especially among urban sites (Fig. 5). We documented higher
grass coverage at urban sites (marginally significant) and between FFE and
BLHWD habitat types. The presence of Japanese Stilt-grass on the urban landscape
contributed to higher grass coverage observed at several trapping locations
in these areas. However, due to high variability, these observations were only
marginally significant. At the Eastern Front (i.e., our urban survey area), efforts
to control the spread of Japanese Stilt-grass are targeted towards BLHWD
habitat and other communities that are heavily invaded and/or susceptible to
invasion (Tim Blumenschine, Petersburg National Battlefield, Petersburg, VA,
pers. comm.). While native grasses may be integral components of small mammal
habitat, invasions by exotic grasses and other understory plants are a major
648
A.D. Chupp, A.M. Roder, L.L. Battaglia, and J.F. Pagels
2013 Northeastern Naturalist Vol. 20, No. 4
detriment to the function of native habitat (Ehrenfeld et al. 2001, McKinney,
2004). Dense populations of Japanese Stilt-grass may have widespread impacts
on the recruitment of native woody species, and thus, limit the amount of shrub
cover for small mammals (Flory 2010). Although several control methods can
mitigate the spread of this species (Judge et al. 2005, Tu 2000), NPS areas are
vulnerable to re-introductions due to heavy traffic of vehicles, recreational activities,
and other avenues of propagule transport (Lesica et al. 1993, Lonsdale
1999, Whipple 2001). In addition, parks located near population centers and
sources of exotic species dispersal (e.g., roadways) may be more vulnerable to
invasions (Tyser and Worley 1992). Controlling the spread of Japanese Stiltgrass
and other invasive grasses is a complex but crucial step towards preserving
suitable habitat in these areas.
In NPS areas, the structure of native habitat may also be reduced by historic
landscape restorations that focus on the cultural significance of historic sites. NPS areas
designated as National Historical Parks, National Monuments, or National Battlefields
may have areas that maintain the cultural appeal of the site at the expense of natural
resource preservation (John Pagels and Adam Chupp, pers. observ.). For example, at
Petersburg National Battlefield in Petersburg, VA, fields of cool season fescue grasses are
used to maintain cultural vistas and “enhance the visitor’s ability to interpret the siege
that occurred around Petersburg” (NPS 2006). However, unlike warm season bunchgrasses
which have good vertical structure and allow a greater freedom of movement
for wildlife while also providing cover (DeLong and Brittingham 2001, Ryan and Marks
2006, Washburn et al. 2000), fescue grasses form dense mats and virtually eliminate
native habitat structure in these culturally restored patches (IDFW 2006; Mengak 2004;
John Pagels and Adam Chupp, pers. observ.). In addition, species of fescue that form
symbiotic relationships with endophytic fungi may be even less attractive to herbivorous
mammals (Coley et al. 1995). Conversion to warm season bunch-grasses and periodic
maintenance (mowing and fescue control) would result in old-field habitat that is preferable
to many small-mammal species. Not captured at any trapping location, Cryptotis
parva (Say) (Least Shrew), Reithrodontomys humulis Audubon & Bachman (Eastern
Harvest Mouse), Microtus pennsylvanicus (Ord) (Meadow Vole), and Zapus hudsonius
Zimmerman (Woodland Jumping Mouse) are frequently found in old-field habitat in
central Virginia (Bellows et al. 2001; Pagels et al. 1992, 2005b). Among both urban and
peri-urban sites, several small-mammal species may benefit from the restoration and
maintenance of old-field habitat with little-to-no impact on the cultural interpretation of
these historic sites. Combining principles of cultural and ecological conservation could
provide numerous opportunities for wildlife conservation in many NPS areas.
In urbanized landscapes, small NPS areas may contain relatively large
remnant habitat patches with the potential for native species conservation. Efforts
to manage these areas for robust mammal assemblages should seek to increase
general habitat complexity for small-mammal species (forest- and field-dwellers,
alike). Controlling the spread of exotic grass species and facilitating the recruitment
of native shrubs may be crucial components of these management strategies.
There is great opportunity for development of adaptive management strategies
Northeastern Naturalist Vol. 20, No. 4
A.D. Chupp, A.M. Roder, L.L. Battaglia, and J.F. Pagels
2013
649
that integrate ongoing NPS efforts to control invasive species with the enhancement
of wildlife habitat in both culturally and naturally preserved areas. Linking
these goals in a flexible management framework could provide an efficient and
effective approach for achieving multiple desirable conservation outcomes. While
balancing the conservation of natural and cultural resources may be challenging,
there are great to the successful integration of these priorities. A great example
that is relevant to this study is the conversion of 202 ha (500 ac) at Manassas
National Battlefield Park (MNBP) in Manassas, VA to warm-season grasses. In
addition to providing higher quality habitat for wildlife, resource managers planted
warm-season grasses wherever possible because these species require no lime
or fertilizer, are adapted to acidic soils, function as a good riparian buffer that
preserves water quality, and maintain historic views of battlefields (NPS 2007).
It should be noted that similar to our urban survey site, the MNBP is located in a
densely populated area and thus influenced by surrounding urban development.
This example is a great success story for managers and conservation biologists to
ponder before making decisions about the direction of future management strategies.
The ecological value of NPS areas is increasingly threatened by human
disturbances. On urban lands that are often culturally preserved, NPS areas offer
unique opportunities for wildlife management and conservation.
Acknowledgments
We wish to thank National Park Service natural resource manager Tim Blumenschine for
his help in the selection and mapping of trapping locations, coordination of field work, and
all other logistical concerns. We thank Jim Comiskey, of the National Park Service, for valuable
discussions and comments throughout the study. We are also grateful to Jesse Fruchter
for his comments and suggestions during the preparation of this manuscript. In addition, two
anonymous reviewers provided excellent comments and recommendations for improving this
manuscript. Stephen Kroh and Khara Coburn assisted with activities in the field. Funds were
made available by the National Park Service Inventory and Monitoring Program with contract
CA4560C0042 to John Pagels at Virginia Commonwealth University, Richmond, VA.
Literature Cited
Andren, H. 1994. Effects of habitat fragmentation on birds and mammals in landscapes with
different proportions of suitable habitat: A review. Oikos 71:355–366.
Bellows S.A., J.F. Pagels, and J.C. Mitchell. 1999. First record of the Least Weasel, Mustela
nivalis (Carnivore: Mustelidae), from the Coastal Plain of Virginia. Northeastern
Naturalist 6:238–240.
Bellows, S.A., J.F. Pagels, and J.C. Mitchell. 2001. Macrohabitat and microhabitat affinities
of small mammals in a fragmented landscape on the upper Coastal Plain of Virginia.
American Midland Naturalist 146:345–360.
Bowman, J.C., D. Sleep, G.J. Forbes, and M. Edwards. 2000. The association of small mammals
with coarse woody debris at log and stand scales. Forest Ecology and Management
129:119–124.
Canfield, R.H. 1941. Application of the line-interception method in sampling range vegetation.
Journal of Wildlife Management 39:388–394.
650
A.D. Chupp, A.M. Roder, L.L. Battaglia, and J.F. Pagels
2013 Northeastern Naturalist Vol. 20, No. 4
Cilliers, S.S., N.S.G. Williams, and F.J. Barnard. 2008. Patterns of exotic plant invasions
in fragmented urban and rural grasslands across continents. Landscape Ecology
23:1243–1256.
Clark, B.K., D.W. Kaufman, G.A. Kaufman, and E.J. Finck. 1987. Use of tallgrass prairie
by Peromyscus leucopus. Journal of Mammalogy 68:158–160.
Clarke, K.R. 1993. Non-parametric multivariate analysis of changes in community structure.
Australian Journal of Ecology 18:117–143.
Clergeau, P., J. Jokimaki, and J.-P.L. Savard. 2001. Are urban bird communities influenced
by the bird diversity of adjacent landscapes? Journal of Applied Ecology 38:1122–1134.
Coley, A.B., H.A. Fribourg, M.R. Pelton, and K.D. Gwinn. 1995. Effects of Tall Fescue endophyte
infestation on relative abundance of small mammals. Journal of Environmental
Quality 24:472–475.
Croci, S., A. Butet, A. Georges, R. Aguejdad, and P. Clergeau. 2008. Small urban woodlands
as biodiversity conservation hot-spot: A multi-taxon approach. Landscape Ecology
23:1171–1186.
Crooks, K.R. 2002. Relative sensitivities of mammalian carnivores to habitat fragmentation.
Conservation Biology 16:488–502.
DeLong, C., and M. Brittingham. 2001. Warm-season grasses and wildlife. Pennsylvania
Wildlife 12:1–8.
DeStefano, S., and R.M. DeGraaf. 2003. Exploring the ecology of suburban wildlife. Frontiers
in Ecology and the Environment 1:95–101.
Dickman, C.R. 1987. Habitat fragmentation and vertebrate species richness in an urban
environment. Journal of Applied Ecology 24:337–351.
Dickman, C.R., and C.P. Doncaster. 1987. The ecology of small mammals in urban habitats.
I. Populations in a patchy environment. Journal of Animal Ecology 56:629–640.
Donnelly, R., and J.M. Marzluff. 2004. Importance of reserve size and landscape context to
urban bird conservation. Conservation Biology 18:733–745.
Dufrene, M., and P. Legendre. 1997. Species assemblages and indicator species: The need
for a flexible asymmetrical approach. Ecological Monographs 67:3 45–366.
Ehrenfeld, J.G., P. Kourtev, and W. Huang. 2001. Changes in soil functions following
invasions of exotic understory plants in deciduous forests. Ecological Applications
11:1287–1300.
Elmhagen, B., and S.P. Rushton. 2007. Trophic control of mesopredators in terrestrial ecosystems:
Top-down or bottom-up? Ecology Letters 10:197–206.
Faulkner, M.B., and T.J. Stohlgren. 1997. Evaluating the contribution of small National
Park areas to regional biodiversity. Natural Areas Journal 17:324–330.
Ferguson, H.L., K. Robinette, and K. Stenberg. 2001. Wildlife of urban habitat. Pp. 317–
341, In D.H. Johnson, and T.A. O’Neil (Eds.). Wildlife-habitat Relationships in Oregon
and Washington. Oregon State University Press, Corvallis, OR. 768 pp.
Flory, S.L. 2010. Management of Microstegium vimineum invasions and recovery of resident
plant communities. Restoration Ecology 18:103–112.
Forman, R.T.T. 1995. Land Mosaics: The Ecology of Landscapes and Regions. Cambridge
University Press, New York, NY. 652 pp.
Garden, J.G., C.A. McAlpine, H.P. Possingham, and D.N. Jones. 2007. Habitat structure is
more important than vegetation composition for local level management of native terrestrial
reptile and small-mammal species living in urban remnants: A case study from
Brisbane, Australia. Austral Ecology 32:669–685.
Northeastern Naturalist Vol. 20, No. 4
A.D. Chupp, A.M. Roder, L.L. Battaglia, and J.F. Pagels
2013
651
Gehrt, S.D., S.P.D. Riley, and B.L. Cyper. 2010. Urban Carnivores: Ecology, Conflict, and
Conservation. John Hopkins University Press, Baltimore, MD. 304 pp.
Handley, C.O., Jr., and M. Varn. 1994. The trapline concept applied to pitfall arrays. Pp.
285–287, In J.F. Merritt, G.L. Kirkland, Jr., and R.K. Rose (Eds.). Advances in the Biology
of Shrews. Carnegie Museum of Natural History, Pittsburgh, PA. 468 pp.
Huckstep, G.S. 1996. Effects of adjacent residential land-use on small mammals inhabiting
woodlands. M.Sc. Thesis. University of Missouri-Columbia, Columbia, MO.
Indiana Division of Fish and Wildlife (IDFW). 2006. Habitat management fact sheet:
Fescue eradication. Available online at http://www.in.gov/dnr/fishwild/files/fescue.pdf.
Accessed August 2012.
Judge, C.A., J.C. Neal, and J.F. Derr. 2005. Preemergence and postemergence control of
Japanese Stiltgrass (Microstegium vimineum). Weed Technology 19:183–189.
Kaufman, D.W., S.K. Peterson, R. Fristik, and G.A. Kaufman. 1983. Effect of microhabitat
features on habitat use by Peromyscus leucopus. American Midland Naturalist
110:177–185.
Kaufman, D.W., M.E. Peak, and G.A. Kaufman. 1985. Peromyscus leucopus in riparian
woodlands: Use of trees and shrubs. Journal of Mammalogy 66:139–143.
Kirkland, G.L., Jr., and P.K. Sheppard. 1994. Proposed standard protocol for sampling of
small mammal communities. Pp. 273-283, In J.F. Merritt, G.L. Kirkland, Jr., and R.K.
Rose (Eds.). Advances in the Biology of Shrews. Carnegie Museum of Natural History
Pittsburgh, PA. 468 pp.
Kotler, B.P. 1984. Risk of predation and the structure of desert rodent communities. Ecology
65:689–701.
Lesica, P., K. Ahlenslager, and J. Desanto. 1993. New vascular plant records and the increase
of exotic plants in Glacier National Park, Montana. Madroño 40:126–131.
Lonsdale, W.M. 1999. Global patterns of plant invasions and the concept of invisibility.
Ecology 80:1522–1536.
Linzey, D.W. 1998. The Mammals of Virginia. The McDonald and Woodward Publishing
Company, Blacksburg, VA. 459 pp.
Mahan, C.G., and T.J. O’Connell. 2005. Small-mammal use of suburban and urban parks in
central Pennsylvania. Northeastern Naturalist 12:307–314.
Manning, J.A., and W.D. Edge. 2004. Small-mammal survival and downed wood at multiple
scales in managed forests. Journal of Mammalogy 85:87–96.
McCune, B., and J.B. Grace. 2002. Analysis of ecological communities. MjM Software
Design Gleneden Beach, OR.
McCune, B., and M.J. Mefford. 1999. PC-ORD. Multivariate analysis of ecological data.
Version 4, MjM Software Design Gleneden Beach, OR.
McKinney, M.L. 2002a. Influence of settlement time, human population, park shape and
age, visitation, and roads on the number of alien plant species in protected areas in the
USA. Diversity and Distributions 8:311–318.
McKinney, M.L. 2002b. Urbanization, biodiversity, and conservation. BioScience 52:883–
890.
McKinney, M.L. 2004. Do exotics homogenize or differentiate communities? Roles of sampling
and exotic species richness. Biological Invasions 6:495–504.
McKinney, M.L. 2006. Urbanization as a major cause of biotic homogenization. Biological
Conservation 127:247–260.
652
A.D. Chupp, A.M. Roder, L.L. Battaglia, and J.F. Pagels
2013 Northeastern Naturalist Vol. 20, No. 4
McShea, W.J., J. Pagels, J. Orrock, E. Harper, and K. Koy. 2003. Mesic deciduous forest as
patches of small-mammal richness within an Appalachian mountain forest. Journal of
Mammalogy 84:627–643.
Mengak, M. T. 2004. Response of small mammal populations to fescue hayfield conversion
to native warm season grasses in Bath County, Virginia. Virginia Journal of Science
55:169–176.
Minchin, P.R. 1989. DECODA: Database for ecological community data. Version 3, Anutech
Pty. Ltd. Canberra, Australia
Mitchell, J.C., S.Y. Erdle, and J.F. Pagels. 1993. Evaluation of capture techniques for
amphibian, reptile, and small mammal communities in saturated forested wetlands.
Wetlands 13:130–136.
National Park Service, Department of the Interior (NPS). 1985. NPS statistics website.
Public Use Statistics Office, Denver, CO. Available online at http://nature.nps.gov/stats/.
Accessed August 2012.
NPS. 2006. Petersburg National Battlefield Nature and Science. Petersburg Natioanl Battlefield,
Hopewell, VA. Available online at http://www.nps.gov/pete/naturescience/index.
htm. Accessed August 2012.
NPS. 2007. Grassland Conservation at Manassas National Battlefield Park. Manassas
National Battlefield Park, Manassas, VA. Available online at http://www.nps.gov/cue/
publications/grassland_4_24_07_low_res.pdf. [PROVIDE DATE ACCESSED].
Nelson, L., Jr., and F.W. Clark. 1973. Correction for sprung traps in catch/effort calculations
of trapping results. Journal of Mammalogy 54:295–298.
Nilon, C.H., and L.W. VanDruff. 1987. Analysis of small-mammal community data and
applications to management of urban greenspaces. Pp. 53-59, In L.W. Adams and D.L.
Leedy (Eds.). Integrating Man and Nature in the Metropolitan Environment. National
Institute for Urban Wildlife Columbia, MD. 249 pp.
Noss, R.F., H.B. Quigley, M.G. Hornocker, T. Merrill, and P.C. Paquet. 1996. Conservation
biology and carnivore conservation in the Rocky Mountains. Conservation Biology
10:949–963.
Orrock, J.L., J.F. Pagels, W.J. McShea, and E.K. Harper. 2000. Predicting presence and
abundance of a small-mammal species: The effect of scale and resolution. Ecological
Application 10:1356–1366.
Pagels, J.F., S.Y. Erdle, K.L. Uthus, and J.C. Mitchell. 1992. Small-mammal diversity in
hardwood forest and clearcut habitats in the Virginia piedmont. Virginia Journal of Science
43:171–176.
Pagels, J.F., A.D. Chupp, and A.M. Roder. 2005a. Survey of mammals at Petersburg National
Battlefield. Technical Report NPS/NER/NRTR—2005:027. National Park Service.
Philadelphia, PA.
Pagels, J.F., A.D. Chupp and A.M. Roder. 2005b. Survey of mammals at Appomattox Court
House National Historical Park. Technical Report NPS/NER/NRTR—2005:030. National
Park Service, Philadelphia, PA.
Pollock, K.H., J.D. Nichols, T.R. Simons, G L. Farnsworth, L.L. Bailey, and J.R. Sauer.
2002. Large-scale wildlife monitoring studies: Statistical methods for design and analysis.
Environmetrics 13:105–119.
Prange, S., S.D. Gehrt, and E.P. Wiggers. 2004. Influences of anthropogenic resources on
Raccoon (Procyon lotor) movements and spatial distribution. Journal of Mammalogy
85:483–490.
Northeastern Naturalist Vol. 20, No. 4
A.D. Chupp, A.M. Roder, L.L. Battaglia, and J.F. Pagels
2013
653
Rice, W.R. 1982. Acoustical location of prey by the Marsh Hawk: Adaptation to concealed
prey. Auk 99:403–412.
Riley, S.P.D. 2006. Spatial ecology of Bobcats and Gray Foxes in urban and rural zones of
a national park. Journal of Wildlife Management 70:1425–1435.
Ryan, M.B., and R. Marks. 2005. Native warm-season grasses and wildlife. Fish and Wildlife
Management Leaflet 25:1–8.
SAS Institute. 2007. Statistical analysis systems version 9.2, SAS Institute, Cary, NC.
Sauvajot, R.M., M. Buechner, D.A. Kamradt, and C.M. Schonewald. 1998. Patterns of
human disturbance and response by small mammals and birds in chaparral near urban
development. Urban Ecosystems 2:279–297.
Silviera, L., A.T.A. Jacomo, and J.A.F. Diniz-Filho. 2003. Camera trap, line-transect census,
and track surveys: A comparative evaluation. Biological Conservation 114:351–355.
Simonetti, J.A. 1989. Microhabitat use by small mammals in central Chile. Oikos
56:309–318.
Sims, V., K.L. Evans, S.E. Newson, J.A. Tratalos, and K.J. Gaston. 2008. Avian assemblage
structure and domestic cat densities in urban environments. Diversity and Distributions
14:387–399.
Sinclair, A.R.E., R.P. Pech, C.R. Dickman, D. Hik, P. Mahon, and A.E. Newsome. 1998.
Predicting effects of predation on conservation of endangered prey. Conservation Biology
12:564–575.
Slade, N.A., and S.M. Blair. 2000. An empirical test of using counts of individuals captured
as indices of population size. Journal of Mammalogy 81:1035–1045.
Snep, R.P.H., P.F.M. Opdam, J.M. Baveco, M.F. WallisDeVries, W. Timmermans, R.G.M.
Kwak, and V. Kuypers. 2006. How peri-urban areas can strengthen animal populations
within cities: A modeling approach. Biological Conservation 127:345–355.
Soule, M.E., D.T. Bolger, A.C. Alberts, R. Sauvagot, J. Wright, M. Sorice, and S. Hill.
1988. Reconstructed dynamics of rapid extinctions of chaparral-requiring birds in urban
habitat islands. Conservation Biology 2:75–92.
Tigas, L.A., D.H. Van Vuren, and R.M. Sauvagot. 2002. Behavioral responses of Bobcats
and Coyotes to habitat fragmentation and corridors in an urban environment. Biological
Conservation 108:299–306.
Tu, M. 2000. Element stewardship abstract for Microstegium vimineum. The Nature Conservancy
Arlington, VA. 9 pp.
Tyser, R.W., and C.A. Worley. 1992. Alien flora in grasslands adjacent to road and trail.
Corriders in Glacier National Park, Montana. Conservation Biology 6:253–262.
VanDruff, L.W., and R.N. Rowse. 1986. Habitat association of mammals in Syracuse, New
York. Urban Ecology 9:413–434.
Van Wagner, C.E. 1968. The line-intersect method in forest fuel sampling. Forest Science
14:20–26.
Wagner, D.M., G.A. Feldhamer, and J.A. Newman. 2001. Microhabitat selection by
Golden Mice (Ochrotmys nuttalli) at arboreal nest sites. American Midland Naturalist
144:220–225.
Warren, W.G., and P.F. Olsen. 1964. A line-intersect technique for assessing logging waste.
Forest Science 10:267–276.
Washburn, B.E., T.G. Barnes, and J.D. Sole. 2000. Improving Northern Bobwhite habitat by
converting Tall Fescue fields to native warm-season grasses. Wildlife Society Bulletin
28:97–104.
654
A.D. Chupp, A.M. Roder, L.L. Battaglia, and J.F. Pagels
2013 Northeastern Naturalist Vol. 20, No. 4
Webster, W.D., J.F. Parnell, and W.C. Biggs, Jr. 1985. Mammals of the Carolinas, Virginia,
and Maryland. University of North Carolina Press, Chapel Hill, NC. 255 pp.
Whipple, J. 2001. Annotated checklist of exotic vascular plants in Yellowstone National
Park. Western North American Naturalist 61:336–346.
Woodroffe, R., and J.R. Ginsberg. 1998. Edge effects and the extinction of populations
inside protected areas. Science 280:2126–2128.