Spatial and Temporal Patterns of Ground-level Ozone
within North-central Pennsylvania Forests
Eric R. Britzke, Price Sewell, Matthew G. Hohmann, Ryan Smith,
and Scott R. Darling
Northeastern Naturalist, Volume 17, Issue 2 (2010): 247–260
Full-text pdf (Accessible only to subscribers.To subscribe click here.)
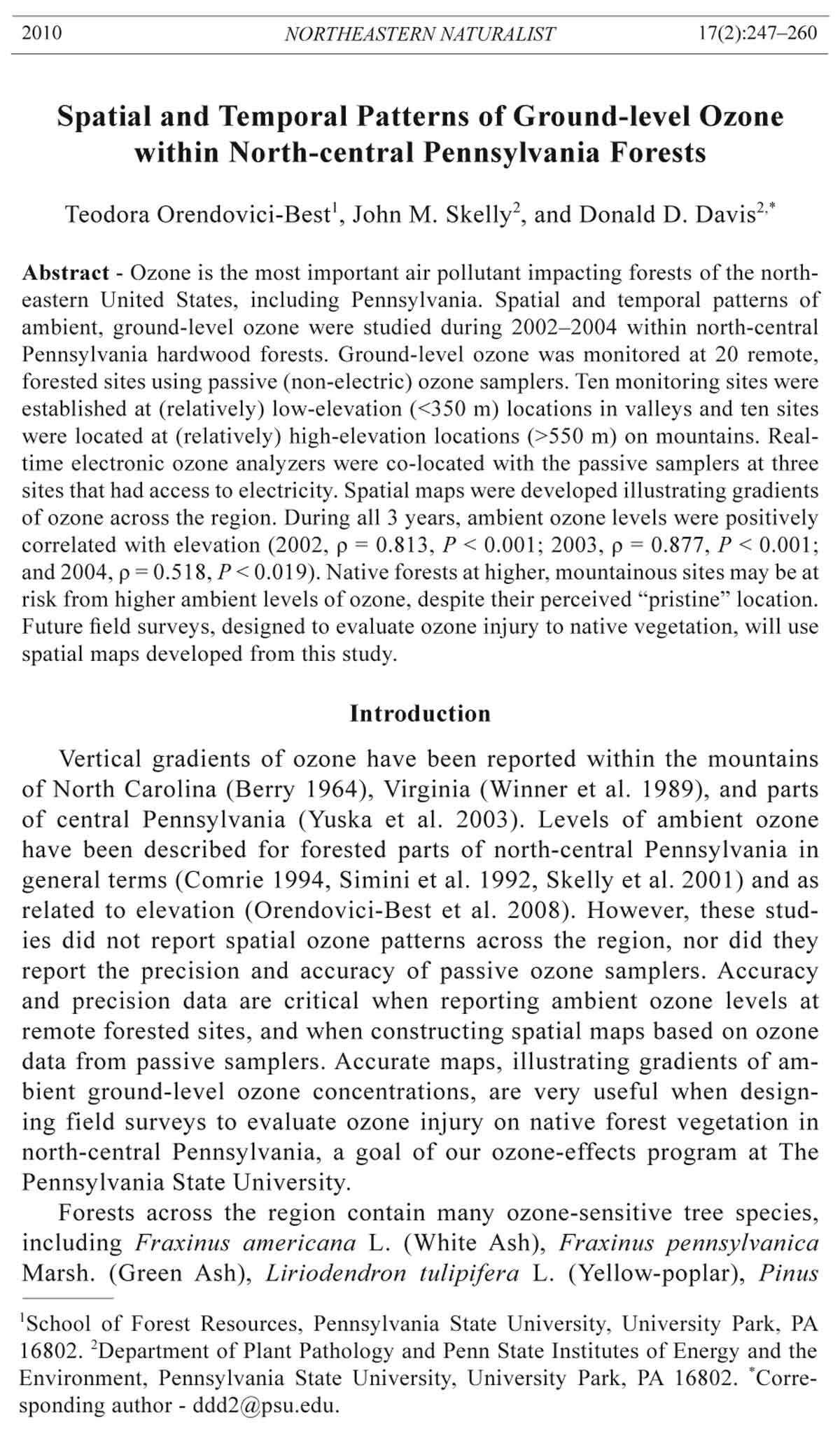
Access Journal Content
Open access browsing of table of contents and abstract pages. Full text pdfs available for download for subscribers.
Current Issue: Vol. 30 (3)
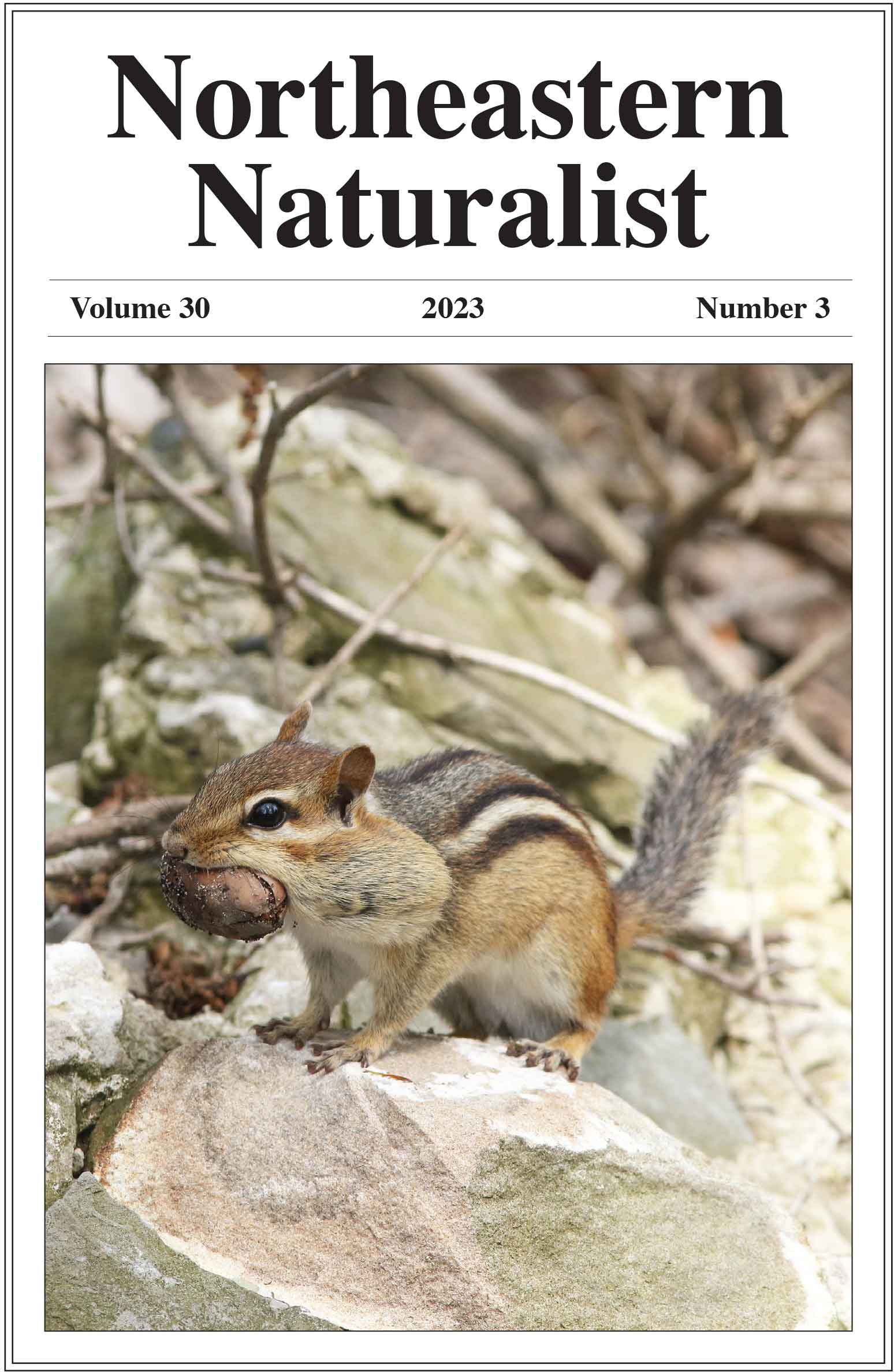
Check out NENA's latest Monograph:
Monograph 22
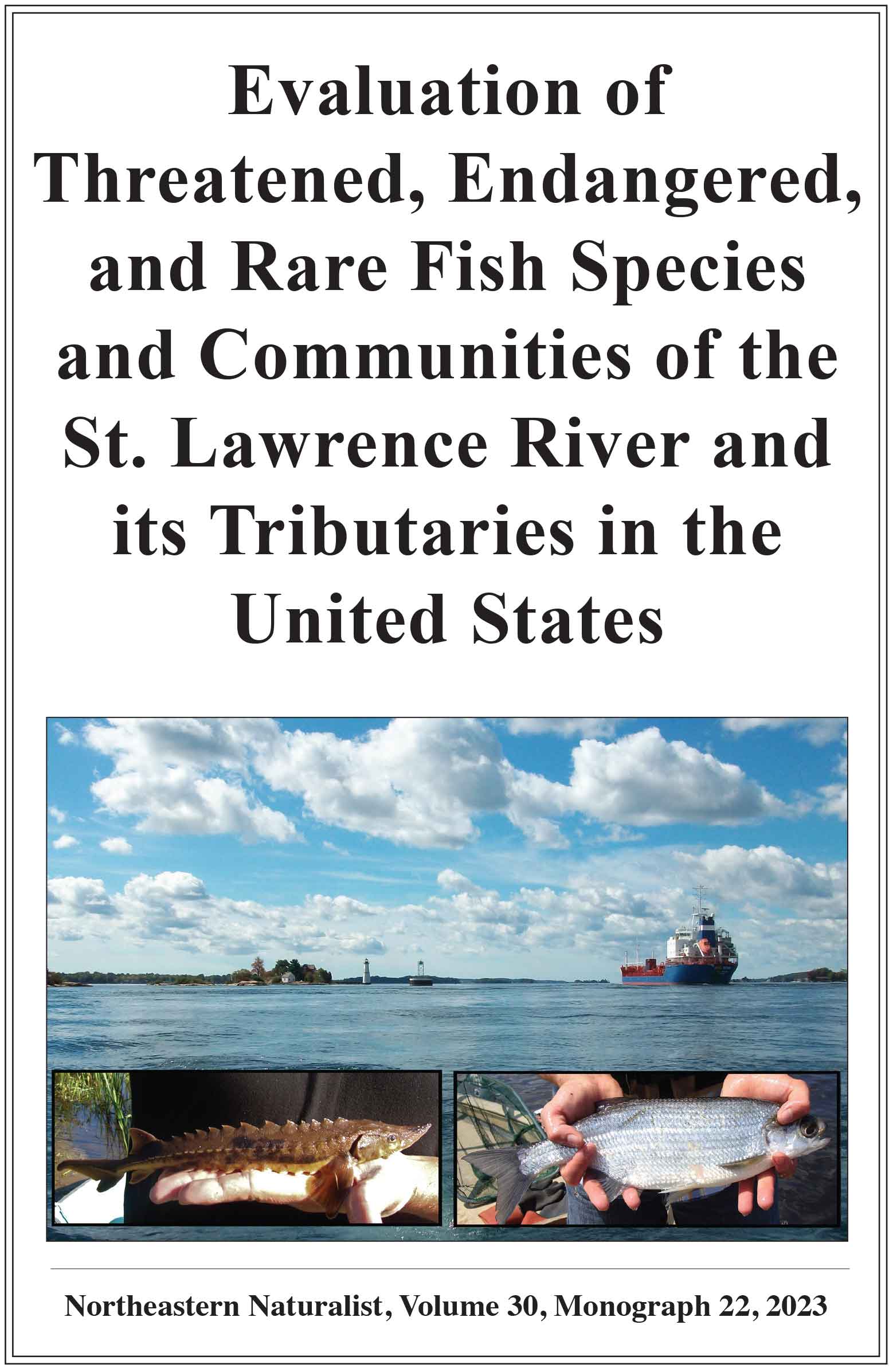
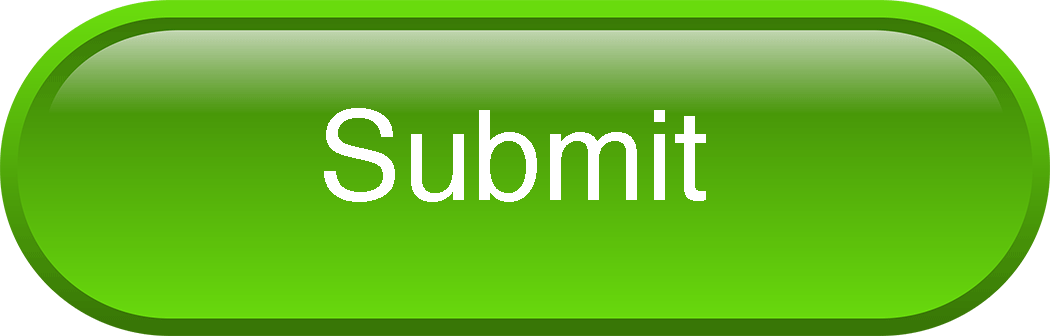
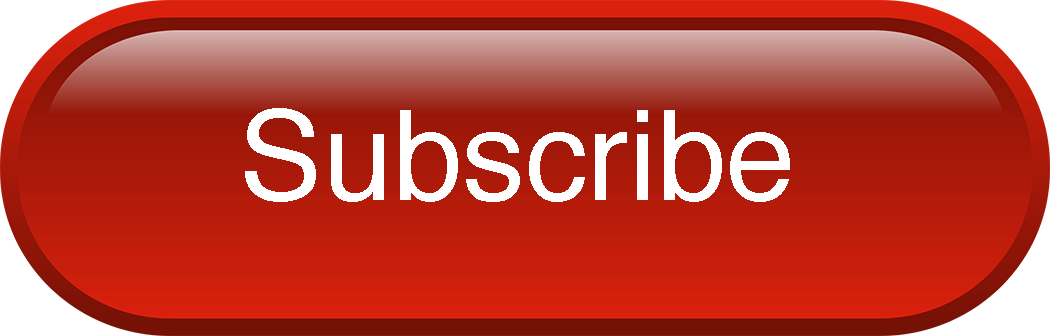
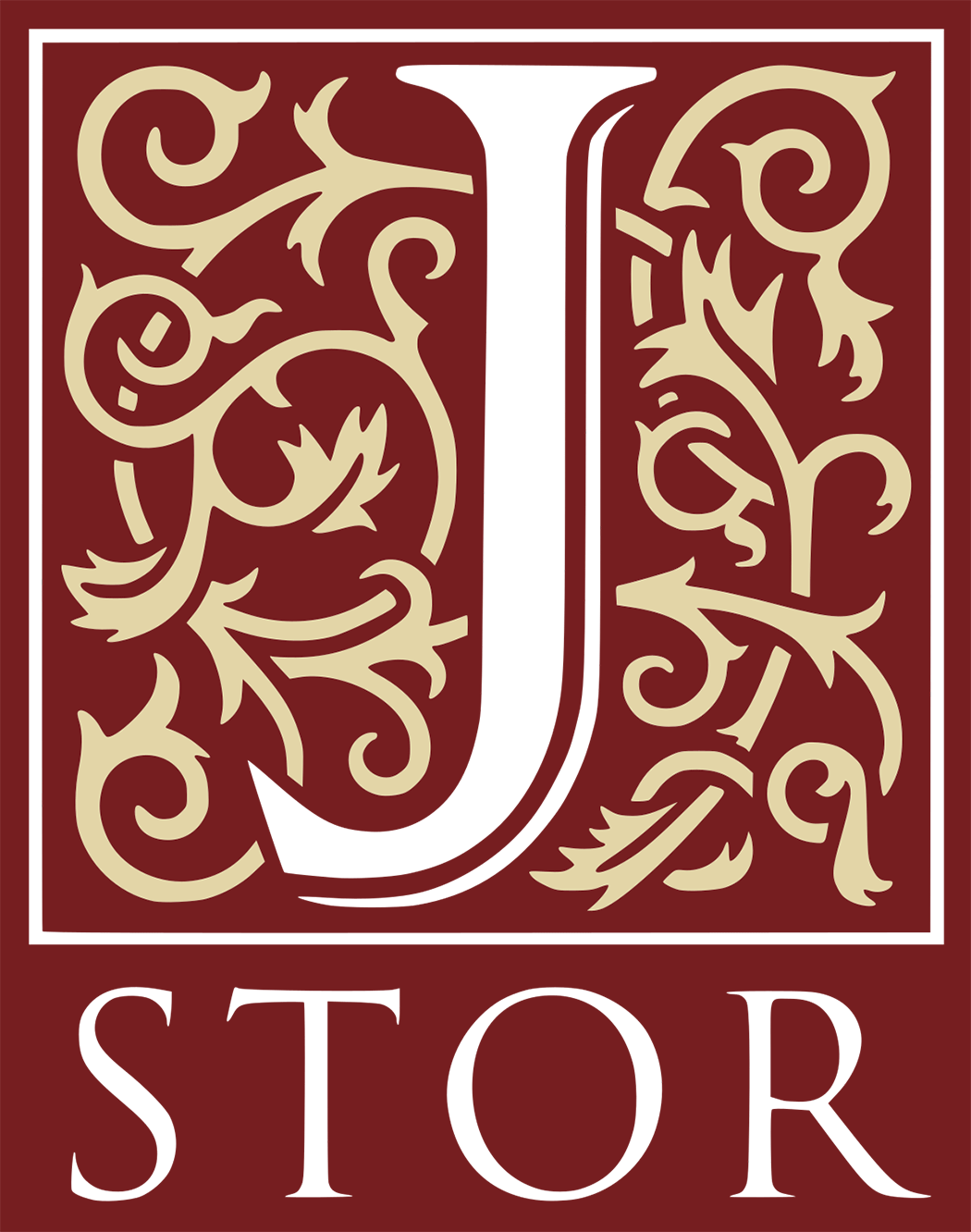


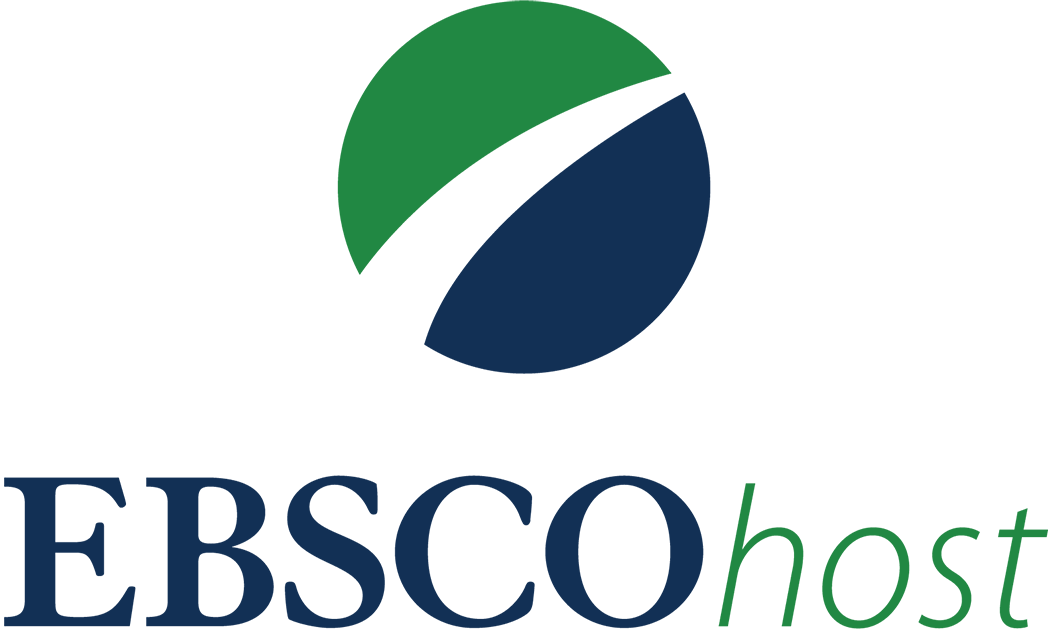

2010 NORTHEASTERN NATURALIST 17(2):247–260
Spatial and Temporal Patterns of Ground-level Ozone
within North-central Pennsylvania Forests
Teodora Orendovici-Best1, John M. Skelly2, and Donald D. Davis2,*
Abstract - Ozone is the most important air pollutant impacting forests of the northeastern
United States, including Pennsylvania. Spatial and temporal patterns of
ambient, ground-level ozone were studied during 2002–2004 within north-central
Pennsylvania hardwood forests. Ground-level ozone was monitored at 20 remote,
forested sites using passive (non-electric) ozone samplers. Ten monitoring sites were
established at (relatively) low-elevation (<350 m) locations in valleys and ten sites
were located at (relatively) high-elevation locations (>550 m) on mountains. Realtime
electronic ozone analyzers were co-located with the passive samplers at three
sites that had access to electricity. Spatial maps were developed illustrating gradients
of ozone across the region. During all 3 years, ambient ozone levels were positively
correlated with elevation (2002, ρ = 0.813, P < 0.001; 2003, ρ = 0.877, P < 0.001;
and 2004, ρ = 0.518, P < 0.019). Native forests at higher, mountainous sites may be at
risk from higher ambient levels of ozone, despite their perceived “pristine” location.
Future field surveys, designed to evaluate ozone injury to native vegetation, will use
spatial maps developed from this study.
Introduction
Vertical gradients of ozone have been reported within the mountains
of North Carolina (Berry 1964), Virginia (Winner et al. 1989), and parts
of central Pennsylvania (Yuska et al. 2003). Levels of ambient ozone
have been described for forested parts of north-central Pennsylvania in
general terms (Comrie 1994, Simini et al. 1992, Skelly et al. 2001) and as
related to elevation (Orendovici-Best et al. 2008). However, these studies
did not report spatial ozone patterns across the region, nor did they
report the precision and accuracy of passive ozone samplers. Accuracy
and precision data are critical when reporting ambient ozone levels at
remote forested sites, and when constructing spatial maps based on ozone
data from passive samplers. Accurate maps, illustrating gradients of ambient
ground-level ozone concentrations, are very useful when designing
field surveys to evaluate ozone injury on native forest vegetation in
north-central Pennsylvania, a goal of our ozone-effects program at The
Pennsylvania State University.
Forests across the region contain many ozone-sensitive tree species,
including Fraxinus americana L. (White Ash), Fraxinus pennsylvanica
Marsh. (Green Ash), Liriodendron tulipifera L. (Yellow-poplar), Pinus
1School of Forest Resources, Pennsylvania State University, University Park, PA
16802. 2Department of Plant Pathology and Penn State Institutes of Energy and the
Environment, Pennsylvania State University, University Park, PA 16802. *Corresponding
author - ddd2@psu.edu.
248 Northeastern Naturalist Vol. 17, No. 2
strobus L. (Eastern White Pine), Prunus pensylvanica L. (Pin Cherry), and
Prunus serotina Ehrh. (Black Cherry) (US DOI 2003). On-going surveys
of forest vegetation by the US Forest Service Ozone Biomonitoring Program
(http://nrs.fs.fed.us/fia/topics/ozone/default.asp) demonstrate that
ozone injury occurs on native vegetation in Pennsylvania and throughout
the Northeast, most notably in the northern hardwood forest types that
include Black Cherry (Coulston et al. 2003). Further, we reported foliar
symptoms on artificial plantings of ozone-sensitive hybrid poplar ramets
and ozone-sensitive selections of Black Cherry seedlings within the region
(Orendovici-Best et al. 2008), confirming the potential for ozone injury to
occur on native forest vegetation. However, to improve the design of future
surveys, and enhance the interpretation of on-going regional surveys, spatial
and temporal patterns of ambient ground-level concentrations of ozone
need to be determined.
The objectives of this study were to 1) develop spatial maps to graphically
illustrate patterns of ozone concentrations across the forested region
of north-central Pennsylvania, and 2) determine precision and accuracy of
passive ozone monitors under the environmental conditions of the region.
Methods
Research area and ozone monitoring
The spatial distribution of tropospheric, ground-level ozone was investigated
during the summers of 2002–2004 in the forested and sparsely
populated areas of north-central Pennsylvania using closely paired study
monitoring sites (Orendovici 2005). Ambient levels of ozone were studied
by selecting 10 sites at relatively low elevations (“valley,” <350 m) and 10
sites at relatively high elevations (“mountain,” >550 m). Each low site was
within 5–20 km of a high site, resulting in 10 pairs of matched monitoring
sites (Table 1). The 20 sites were all located within well-exposed clearings
in forests.
Passive ozone samplers do not require electricity and can be used for
monitoring ozone in remote forested areas (Bytnerowicz et al. 2002, Cox
2003). Ogawa passive ozone samplers (Ogawa 1998; Pompano Beach, fl)
were placed at 1.5–2.0 m on poles at each of the 20 sites. Each sampler was
placed under a plastic cap to provide protection from wind and rain, and
contained two nitrite-saturated filters that were kept frozen in an insulated
ice chest until placement. Samplers were replaced weekly at approximately
the same time of day. Filters were returned to the laboratory and analyzed
using ion chromatography to determine conversion of nitrite (NO2) to nitrate
(NO3) for 1-week periods (Orendovici 2005).
Real-time, electronic ozone analyzers were co-located with passive
ozone samplers at three of the monitoring sites that had access to electricity
(Table 1). TECO Model 49 (Hopkinton, MA) ozone analyzers were operated
in 2002 and 2003, whereas an API Model 400A (San Diego, CA) was used
in 2004. Ozone levels (ppb) were monitored at 5-min intervals and recorded
2010 T. Orendovici-Best, J.M. Skelly, and D.D. Davis 249
as 1-hr averages using an Odessa Engineering data-logger model DSM3260
(Austin, TX). Sensors were calibrated in early April of each year, and each
analyzer performed a 2-point calibration check nightly. Calibration and
quality control measures followed the standards set by the Pennsylvania
Department of Environmental Protection, Bureau of Air Quality, Harrisburg,
PA. Ozone monitoring was conducted from 13 June to 9 September 2002 (12
weeks), 9 June to 9 September 2003 (13 weeks), and 23 May to 31 August
2004 (14 weeks). At the co-located sites, temperature (oC), relative humidity
(%), precipitation (mm), and wind speed (ms-1) were recorded with Campbell
meteorological data systems (Campbell Scientific Inc., Logan, UT).
Resulting data were used to evaluate the influence of meteorological factors
on the precision and accuracy of the passive samplers.
Data analyses
The precision of the passive ozone samplers was determined by deploying
duplicate samplers at three sites (Table 1) where a 1-week average ozone concentration
was calculated. Sampler precision was determined by calculating
the percentage difference between replicates (relative error * 100). Relative
error is defined as the ratio of the absolute value of the difference of the replicates
samplers and their average. The accuracy of the passive monitors was
determined by comparing values from the passive monitors with those obtained
from the (more accurate) co-located electronic ozone monitors.
Table 1. Location of paired monitoring sites equipped with Ogawa passive ozone samplers in
north-central Pennsylvania.
Elevation Pair distance
Site name County Latitude Longitude (m) Pair (Km)
CURWENSVILLE CLEARFIELD 40 56 54 78 32 29 320 1
LUTHERSBURG CLEARFIELD 41 04 15 78 42 24 540 1 19.45
PENFIELD CLEARFIELD 41 13 05 78 35 21 390 2
MOSHANON SFA CLEARFIELD 41 07 02 78 32 01 660 2 11.67
MEDIX RUN ELK 41 17 35 78 24 07 300 3
PIPER CLEARFIELD 41 13 01 78 09 58 660 3 20.26
HYNER PARK CLINTON 41 21 49 77 38 14 285 4
PETE’S RUN CLINTON 41 15 10 77 45 50 690 4 16.46
CEDAR RUN LYCOMING 41 30 44 77 27 42 250 5
GAGE ROAD LYCOMING 41 24 09 77 32 37 570 5 14.24
PINE LAKE LYCOMING 41 22 20 77 21 47 250 6
TIADAGHTON A LYCOMING 41 20 05 77 27 35 550 6 9.07
ROTE CLINTON 41 05 24 77 28 30 250 7
PINE ROAD CLINTON 41 04 21 77 19 05 550 7 13.28
CANTON TIOGA 41 40 14 76 50 12 350 8
GLEASONA TIOGA 41 39 14 76 56 37 700 8 9.06
MT. PISGAH ST BRADFORD 41 48 39 76 40 27 350 9
MT. PISGAH CO BRADFORD 41 49 03 76 42 58 680 9 4.55
OGDONIA SULLIVAN 41 25 05 76 42 28 300 10
WORLD'S END SULLIVAN 41 26 03 76 36 43 600 10 8.18
ASite where duplicate passive ozone samplers were co-located with real-time ozone analyzers,
and a meteorological station. All other sites contain only passive ozone samplers.
250 Northeastern Naturalist Vol. 17, No. 2
Weekly ozone averages were used to compute seasonal ozone averages
for each site. ANOVA was used to test for significant differences (P-value
< 0.05) of seasonal ozone concentrations among sites and years. Seasonal
averages were also used to compare ozone concentrations at different elevations
and to reveal spatial patterns. Regression analysis was used to study
the relationship between seasonal ozone concentrations and elevation.
Spatial maps illustrating patterns of ozone distributions were generated
using spatial analysis (Kaluzny et al. 1998) of the data within the monitored
area. Intensity maps of ozone distributions were generated using an interpolation
algorithm that estimated spatial ozone distribution. The algorithm
was selected based on positive variance and the lowest errors criteria (Orendovici
2005).
Data were analyzed using the Statistical Analysis System (SAS, Inc.
2000), MINITAB Release 14 (Minitab, Inc. 2003), and S-plus (S-plus 2003).
Results
Sampler precision and accuracy
During the 2002 season, we did not employ duplicate samplers; therefore
the precision and accuracy of the passive samplers was not studied. In 2003,
the precision of the Ogawa passive samplers was between 0 and 20%, and
the range of the differences (between the two replicate samplers over oneweek
period) was (-5.25; 5.73 ppb), with one outlying value of 14.64 ppb.
The precision of the Ogawa passive samplers in 2004 was between 0 and
20%, and the range of the differences was (-5.42; 4.77 ppb), with two outlying
values of 15.42 and 10.34 ppb.
Accuracy, as measured by the correlation coefficient between weekly average
of ozone measured via passive samplers vs. electronically monitored
ozone, was 0.853 (P < 0.001) in 2002, 0.911 (P < 0.001) in 2003, and 0.064
(P = 0.561) in 2004. When data from the Gleason site was removed in 2004,
the accuracy increased to 0.712 (P < 0.001); therefore, passive data from
that site was not used in 2004. These results revealed that mean ozone values
from the passive samplers generally corresponded to the data from real-time
electronic monitors.
Ozone and elevation
There were significant positive correlations between seasonal ozone concentrations
and elevation in 2002 (ρ = 0.813, P < 0.001), 2003 (ρ = 0.877, P <
0.001), and 2004 (ρ = 0.518, P < 0.019), revealing that ozone concentration
increased with elevation. The differences in ozone concentrations between
low- and high-elevation sites were greater in 2002 and 2003 than in 2004
(Fig. 1). There were greater differences in seasonal ozone concentrations
between paired sites when the high-elevation site was >600 m altitude. In
2004, the differences in seasonal ozone between sites were less than the
previous 2 years, and the differences were not always significant. During all
2010 T. Orendovici-Best, J.M. Skelly, and D.D. Davis 251
Figure 1. Comparison of seasonal ozone between pair of sites (high vs. low elevation)
during 2002–2004. H = high elevation and L = low elevation site; data represents
means ± SE.
252 Northeastern Naturalist Vol. 17, No. 2
3 years of the investigation, the highest ozone concentrations were found at
Mt. Pisgah County Park and Pete’s Run, two of the highest elevation sites.
In contrast, the lowest ozone concentrations were found at Hyner Park, one
of the lowest elevation sites (Table 1). Elevation was a significant factor
in determining ozone concentrations, but meteorological conditions and
hourly mean patterns influenced weekly ozone levels as well. Ozone values
from the three monitoring sites were positively correlated with temperatures
and wind speed values in 2002 and 2003, but negatively correlated in 2004
(Table 2). The correlation between ozone level and % relative humidity was
negative for all 3 years.
Table 2. Correlation coefficients between ambient ozone concentrations, temperature, relative
humidity, and wind speed recorded at the air quality monitoring sites in north-central Pennsylvania
during 2002–2004.
Year Variables Correlation coefficient P-value
2002 O3, Temperature 0.738 <0.001
O3, Relative humidity -0.582 <0.001
O3, Wind speed 0.342 <0.001
2003 O3, Temperature 0.614 <0.001
O3, Relative humidity -0.575 <0.001
O3, Wind speed 0.478 <0.001
2004 O3, Temperature -0.034 0.003
O3, Relative humidity -0.101 <0.001
O3, Wind speed -0.070 <0.001
Figure 2. Hourly ozone behavior from 17 July 2002 to 23 July 2002 at the three sites
equipped with real-time ozone monitors.
2010 T. Orendovici-Best, J.M. Skelly, and D.D. Davis 253
Hourly patterns of ozone
The real-time electronic ozone monitors co-located with passive samplers
monitors at three sites provided the means to not only study the accuracy of
the passive sampler devices, but also to study daily ozone patterns. Diurnal
distribution of ozone at the Tiadaghton and Moshannon monitoring sites
expressed the typical ozone fluctuation, having daily maxima during early
afternoon hours and daily minima at night. However, a significant drop did
not follow the maximum daily ozone values at the Gleason site at night in
2002 (Fig. 2) and 2003 (data not shown).
Spatial and seasonal variation
Seasonal means of ozone concentrations were compared to determine
significant differences among monitoring sites (Table 3). When two-way
ANOVA was used, it revealed that both year of study and location were
Table 3 (continued on next page). Results of ANOVA test for significant differences in ozone
concentrations measured with Ogawa passive samplers in north-central Pennsylvania during
2002–2004.
Seasonal ozone
Year Site Elevation (m) mean (ppb) Std. Tukey groupingA
2002 GLEASON 700 54.08 10.35 A
MT. PISGAH CO 680 53.38 10.87 A
PETE’S RUN 690 53.04 8.19 A
MOSHANNON 660 46.39 8.35 B A
WORLD’S END 600 46.08 10.23 B A
PIPER 660 44.11 4.04 B A C
CANTON 350 40.25 8.19 B D C
MT. PISGAH ST 350 40.10 7.13 B D C
TIADAGHTON 550 37.10 8.51 B E D C
PINE ROAD 550 36.46 5.04 B E D C
LUTHERSBURG 540 35.77 5.83 B E D C
CURWENSVILLE 320 34.89 4.85 E D C
GAGE ROAD 570 34.01 9.10 E D C
PINE LAKE 250 33.91 4.99 E D C
ROTE 250 32.31 5.07 E D
CEDAR RUN 250 32.14 5.30 E D
PENFIELD 390 30.98 4.78 E D
MEDIX RUN 300 30.38 3.90 E D
OGDONIA 300 28.46 7.69 E
HYNER PARK 285 26.77 5.54 E
2003 PETE’S RUN 690 47.73 11.50 A
MT. PISGAH CO 680 47.22 8.66 B A
GLEASON 700 45.41 7.37 B A C
MOSHANNON 660 40.12 7.29 B D A C
WORLD’S END 600 37.61 8.46 B D E C
PIPER 660 37.18 8.03 B D E C
TIADAGHTON 550 32.76 6.00 F D E G
MT. PISGAH ST 350 30.96 8.45 H F D E G
LUTHERSBURG 540 30.35 5.22 H F E G
PINE ROAD 550 29.06 7.09 H F E G
GAGE ROAD 570 28.96 5.76 H F E G
CANTON 350 28.54 7.16 H F E G
254 Northeastern Naturalist Vol. 17, No. 2
significant sources of variance, and that there was a year-by-location
interaction (all P-values < 0.001). In 2004, ozone concentrations decreased
at high-elevation monitoring sites and increased at low-elevation sites; therefore,
there was not a significant difference between ozone means in 2003 and
2004 (Fig. 1). One-way ANOVA Tukey’s test showed that seasonal ozone
concentrations were different in 2002 (μ = 38.479, σ = 10.704), as compared
to 2003 (μ = 32.858, σ = 10.535) or 2004 (μ = 33.142, σ = 5.192), and that
in 2002 and 2003, ozone concentrations at sites >600 m were grouped in
similar classes (A and B), with none of the sites <600 m being in class A;
however, in 2004, ozone concentrations grouped differently and some of the
sites <500 m were in class A of ozone values (Table 3).
Maps describing seasonal averages for each year were constructed to
evaluate spatial and temporal patterns of ozone (Fig. 3a, b, c). Weekly ozone
distribution maps (Orendovici 2005) showed similar weekly and seasonal
trends in 2002 and 2003, with little spatial variability of ozone distribution
from week to week. Temporal variation was minimal during 2002 and 2003.
The highest temporal variability of ozone distribution was observed in 2004,
Table 3, continued from previous page.
Seasonal ozone
Year Site Elevation (m) mean (ppb) Std. Tukey groupingA
CURWENSVILLE 320 27.88 6.94 H F G
PENFIELD 390 27.78 5.91 H F G
ROTE 250 26.58 5.86 H G
OGDONIA 300 25.14 7.76 H G
PINE LAKE 250 24.82 4.90 H G
CEDAR RUN 250 23.76 6.02 H G
MEDIX RUN 300 23.29 5.02 H G
HYNER PARK 285 22.30 7.05 H
2004 MT. PISGAH CO 680 38.76 4.11 A
WORLD’S END 600 37.95 5.40 A
PETE’S RUN 690 37.79 3.19 A
PIPER 660 36.98 6.85 B A
MOSHANNON 660 36.39 1.62 B A C
CURWENSVILLE 320 34.84 4.93 B D A C
PINE ROAD 550 34.68 4.95 B D A C
CANTON 350 34.57 4.52 B D A C
GAGE ROAD 570 33.91 4.95 B D A C
LUTHERSBURG 540 33.71 4.71 B D A C
MT. PISGAH ST 350 32.34 3.48 B D C
OGDONIA 300 32.10 4.27 B D C
PENFIELD 390 31.99 4.33 B D C
TIADAGHTON 550 31.59 2.17 B D E C
ROTE 250 31.41 4.64 D E C
CEDAR RUN 250 31.01 4.23 D E
PINE LAKE 250 30.82 4.03 D E
MEDIX RUN 300 30.60 4.87 D E
HYNER PARK 285 29.90 4.54 E
GLEASON 700 26.46 2.76 E
AMeans followed the same letter are not statistically different at the 0.05 level of significance.
2010 T. Orendovici-Best, J.M. Skelly, and D.D. Davis 255
Figure 3.
Maps of estimated
seasonal
ozone
concentration
in northcentral
Pennsylvania
for
2002 (a),
2003 (b), and
2004 (c).
256 Northeastern Naturalist Vol. 17, No. 2
when high-elevation monitoring sites experienced higher ozone variability,
whereas other locations showed less variation in ozone concentration (Orendovici
2005).
In 2002 and 2003, there was a consistent trend of lower ozone concentrations
in the central part of the study region. Even during periods of overall
high ozone concentrations, the central region exhibited low ozone levels.
In 2003, average ozone values were significantly lower than in 2002, but
the spatial trend was consistent with that observed in 2002. That is, areas
with higher ozone concentrations surrounded the central part of the region
that had lower ozone concentrations. Seasonal ozone averages were not
significantly different between 2003 and 2004, but the spatial patterns were
different. In 2004, ozone concentrations were more uniformly distributed
throughout the entire region. Nevertheless, the central area exhibited lower
ozone concentrations regardless of season (Fig. 3c).
Discussion
Passive samplers for quantification of cumulative ozone exposures are
inexpensive, easy to deploy, reliable, and of necessity in rural forested
areas without electricity. Generally, passive samplers are not affected by
temperature, humidity, or interference with other co-pollutants (Koutrakis
et al. 1993). Nevertheless, there are limitations and uncertainties that need
consideration. Interferences from other oxidants besides ozone may occasionally
cause overestimation or underestimation of the cumulative ozone
concentration measured with passive samplers (Campbell et al. 1994). Turbulent
transfer caused by wind incursion into the open-ended tube sampler
may result in overestimation of the gas concentrations by as much as 30%
(Campbell et al. 1994). It is recommended that some electronic monitors
be co-located with passive monitors to obtain estimates of accuracy and
reduction of uncertainties (Cox 2003). Our 3-year ozone monitoring investigation
revealed that passive samplers are useful in remote areas, but
accuracy of passive samplers is highly dependent upon wind velocity, ambient
temperatures, and relative humidity. During years of low temperature
and high winds (i.e., 2004), the accuracy of passive samplers is lower. Care
in placement and protection of the samplers did not always minimize the
problem, and diffusion barriers did not always provide protection against
changing wind turbulence. Also, since meteorological data are often not
available in rural areas, it may not be possible to use wind data to adjust
passive sampler values.
During all 3 years of study, there was a significant positive correlation
between elevation and seasonal ozone. In a typical year, monitoring sites
at higher elevations exhibited higher ozone values. Yuska et al. (2003) also
reported greater ozone levels at higher elevations in central Pennsylvania,
but did not utilize paired sites. Our results with paired monitoring sites
indicate that the difference between ozone at low/high sites is due to elevation
(Fig. 1). Sites >600 m elevation generally exhibited greater ozone
2010 T. Orendovici-Best, J.M. Skelly, and D.D. Davis 257
concentrations than valleys. For sites <600 m, the differences in ozone concentrations
between mountain and valley were not significant. In 2004, there
was an exception from the higher elevation higher ozone pattern when two
sites at lower elevations (Curwensville and Canton) exhibited greater ozone
concentrations than their paired higher elevation sites (Table 3).
The hourly data from the real-time monitors was used to study the
ozone and elevation relationship. The diurnal distribution of ozone in
the study area followed the typical daily pattern, exemplified by data
from the Tiadaghton and Moshannon monitoring sites, wherein maximum
concentrations occurred during early afternoon and minimum concentrations
occurred at night (Fig. 2). However, this diurnal pattern was not
observed at the Gleason monitoring site (Fig. 2), where ozone levels remained
high at night. Differences in average ozone concentrations among
sites may be partially due to differences in ozone levels between day and
night, but as related to elevation. The Tiadaghton monitoring site, located
at 550 m, exhibited lower ozone even when surrounding areas exhibited
high levels of ozone. Moshannon, located at 660 m, showed high ozone
values during daytime, but dropped significantly at night. Gleason, located
at 700 m, showed little day-to-night variation in ozone concentrations, and
thus exhibited higher daily averages. Apparently, 600 m in elevation within
the study region separates higher ozone exposure areas from lower ozone
exposure areas.
Lefohn et al. (1992) described the relationship between ozone and elevation
as being dependent upon time of day. In our study, daily minimum
and maximum concentrations were dependent upon site, elevation, and meteorological
conditions. When meteorological conditions were conducive to
major ozone episodes, the difference in ozone concentrations between lower
and higher elevations was likely imposed by the difference between day and
night ozone values. During the day, ozone concentrations were quite similar
at sites of varying elevation. However, due to vertical mixing and depositions
at night, lower elevation sites exhibited lower average ozone levels.
There have been reports of sometimes significant nocturnal leaf conductance
resulting in nocturnal ozone uptake, which might be more harmful to plants
than diurnal ozone uptake (Emberson et al. 2000, Matyssek et al. 1993, Musselman
and Minnick 2000). Further study is needed to determine if native
vegetation is subject to a greater risk of injury from high night ozone levels
at higher elevation sites in Pennsylvania.
Intensity maps (Fig. 3) of ozone distribution over north-central Pennsylvania
revealed a persistent pattern of low ozone in the central part of the region,
surrounded by higher ozone concentrations. This pattern was also reported by
Jagodzinski (2000), Skelly et al. (1994), and Simini et al. (1992), but never
explained. Simini et al. (1992) reported a west-to-east decreasing gradient of
ozone concentrations across north-central Pennsylvania. However, their easternmost
monitoring site was located at Tiadaghton. In our study, we monitored
ozone farther east of Tiadaghton and observed that ozone concentrations were
258 Northeastern Naturalist Vol. 17, No. 2
actually increasing with increasing elevation. Skelly et al. (2001) suggested
that the pattern of lower ozone concentrations in the north-central region of
Pennsylvania was likely related to regional air masses that originate in the
northwest part of the state (Great Lakes), usually characterized by low ozone
concentrations. However, our study revealed that monitoring sites exhibiting
the greatest ozone concentrations in that area were all >600 m, and had signifi-
cantly greater ozone levels than sites <600 m elevation (Table 3). Prior to this
study, lower ozone concentrations in the central area of north-central Pennsylvania
had not been related to topography.
This study resulted in the production of spatial maps that illustrate patterns
of ground-level ambient ozone within forested areas of north-central
Pennsylvania. We also described the relationship between elevation and
ambient ozone concentrations, and confirmed that passive ozone samplers
were useful devices to monitor ozone within the environmental constraints
of forested north-central Pennsylvania. These results, along with those of
Orendovici et al. (2008), form a solid platform from which to launch impact
studies on ozone-sensitive tree species, including Eastern White Pine, Green
Ash, Pin Cherry, Black Cherry, White Ash, and Yellow-poplar (US DOI
2003) within the high-risk (i.e., high-elevation, high-ozone) areas delineated
by this study.
Acknowledgments
The authors thank the Pennsylvania Department of Environmental Protection,
Bureau of Air Quality for the main financial support for this study. Allegheny
Energy Supply of Greensburg, PA and Reliant Energy of Johnstown, PA also supported
this project. We wish to thank the Pennsylvania Bureau of Forestry, the
Tiadaghton Sportsman’s Club, The Mountain Run Hunt Club, and many other local
landowners for allowing the placement of the PA-DEP buildings, electrical supply
lines, and the passive samplers on their properties, and to Penn State Institutes for
Energy and the Environment for the laboratory analyses. The authors gratefully
acknowledge technical assistance from J. Ferdinand, J. Savage, and R. Stevenson.
Literature Cited
Berry, C.R. 1964. Differences in concentrations of surface oxidant between valley
and mountaintop conditions in the southern Appalachians. Journal of the Air Pollution
Control Association 14:238–239.
Bytnerowicz, A., M. Tausz, R. Alonso, D. Jones, R. Johnson, and N.E. Grulke. 2002.
Summertime distribution of air pollutants in the Sequoia National Park, California.
Environmental Pollution 118:187–203.
Campbell, G.W., J.R. Stedman, and A. Stevenson. 1994. A survey of nitrogen dioxide
concentrations in the United Kingdom using diffusion tubes, July–December
1991. Atmospheric Environment 28:477–486.
Comrie, A.C. 1994. A synoptic climatology of surface ozone in rural ozone pollution
at three forest sites in Pennsylvania. Atmospheric Environment 28:1601–1614.
Coulston, W., G.C. Smith, and W.D. Smith. 2003. Regional assessment of ozonesensitive
tree species using bioindicator plants. Environmental Monitoring and
Assessment 83:113–127.
2010 T. Orendovici-Best, J.M. Skelly, and D.D. Davis 259
Cox, R.M. 2003. The use of passive sampling to monitor forest exposure to O3,
NO2, and SO2: A review and some case studies. Environmental Pollution
126:301–311.
Emberson, L.D., M.R. Ashmore, H.M. Cambridge, D. Simpson, and J.P. Tuovinen.
2000. Modeling stomatal ozone flux across Europe. Environmental Pollution
109:403–412.
Jagodzinski, J.M. 2000. An analysis of tropospheric ozone transport in north-central
Pennsylvania during the summer of 1999. M.Sc. Thesis. Pennsylvania State University,
University Park, PA.
Kaluzny, S.P., S.C. Vega, T.P. Cardoso, and A.A. Shelly. 1998. S+Spatial Stats:
User’s Manual for Windows and UNIX. Springer-Verlag, New York, NY.
Koutrakis, P., J.M. Wolfson, A. Bunyaviroch, S.E. Froelich, K. Hirano, and J.D.
Mulik. 1993. Measurement of ambient ozone using a nitrite-saturated filter. Analytical
Chemistry 65:210–214.
Lefohn, A.S., H.P. Knudsen, D.S. Shadwick, and K.A. Hermann. 1992. Surface
ozone exposures in the eastern United States (1985–1989). Pp. 81–93, In R.B.
Flagler, (Ed.). Transactions of the Response of Southern Commercial Forests to
Air Pollution Specialty Conference. Air and Waste Management Association,
Pittsburgh, PA.
Matyssek, R., T. Keller, and T. Koike. 1993. Branch growth and leaf gas exchange of
Populus tremula exposed to low ozone concentrations throughout two growing
seasons. Environmental Pollution 79:1–7.
Minitab, Inc. 2003. MINITAB Statistical Software, Release 14 for Windows. State
College, PA.
Musselman, R.C., and T.J. Minnick. 2000. Nocturnal stomatal conductance and ambient
air quality standards for ozone. Atmospheric Environment 34:719–733.
Ogawa and Company, USA, Inc. (Ogawa). 1998. NO–NO2 simultaneous sampling
protocol using Ogawa sampler. Pompano Beach, fl.
Orendovici, T. 2005. The response of Black Cherry and hybrid poplar to ozone under
varying environmental conditions. Ph D. Dissertation. Pennsylvania Sate University,
University Park, PA.
Orendovici-Best, T., D.D. Davis, J.M. Skelly, J.A. Ferdinand, J.E. Savage, and R.E.
Stevenson. 2008. Modeling ozone uptake (flux) and determining the influence of
ozone on the foliar symptoms of Prunus serotina seedlings and Populus maximowicii
x trichocarpa ramets. Environmental Pollution 151:79–92.
SAS Institute, Inc. 2000. SAS/STAT User’s Guide, Version 6, Fourth Edition. SAS
Institute Inc., Cary, NC.
Simini, M., J.M. Skelly, D.D. Davis, J.E. Savage, and A.C. Comrie. 1992. Sensitivity
of four hardwood species to ambient ozone in north-central Pennsylvania.
Canadian Journal of Research 22:1789–1799.
Skelly, J.M., J.E. Savage, K.R. Snyder, and T.S. Fredericksen. 1994. Tropospheric
ozone occurrences at remote sites in Pennsylvania: A database for evaluation of
changing pollution scenarios. Environmental Resources Institute, Pennsylvania
State University, University Park, PA.
Skelly, J.M., J.A. Ferdinand, J.M. Jagodzinski, J.E. Savage, and J.D. Mulik. 2001. A
thirteen-week comparison of passive and continuous ozone monitors at forested
sites in north-central Pennsylvania. Journal of the Air and Waste Management
Association 51:1280–1287.
260 Northeastern Naturalist Vol. 17, No. 2
S-PLUS 6 for Windows (S-plus). 2003. Insightful Corporation, Seattle, WA.
United States Department of the Interior (US DOI). 2003. Ozone sensitive plant species
on National Park Service and US Fish and Wildlife Service Lands: Results
of a June 24–25, 2003 workshop, Baltimore, MD. Natural Resource Report NPS/
NRARD/NRR-2003/01. 21 pp.
Winner, P.B., A.S. Lefohn, I.S. Cotter, C.S. Greitner, L.R. Nellesen, C.J. McEvoy,
R.L. Olson, C.J. Atkinson, and L.D. Moore. 1989. Plant responses to elevational
gradients of O3 exposures in Virginia. Proceedings of the National Academy of
Science, USA 86:8828–8832.
Yuska, D.E., J.M. Skelly, J.A. Ferdinand, R.E. Stevenson, J.E. Savage, J.D. Mulik,
and A. Hines. 2003. Use of bioindicators and passive sampling devices to evaluate
ambient ozone concentrations in north-central Pennsylvania. Environmental
Pollution 125:71–80.