Status and Distribution of Fish in an Acid-impacted
Watershed of the Northeastern United States
(Hubbard Brook, NH)
Dana R. Warren, Gene E. Likens, Donald C. Buso,
and Clifford E. Kraft
Northeastern Naturalist, Volume 15, Issue 3 (2008): 375–390
Full-text pdf (Accessible only to subscribers.To subscribe click here.)
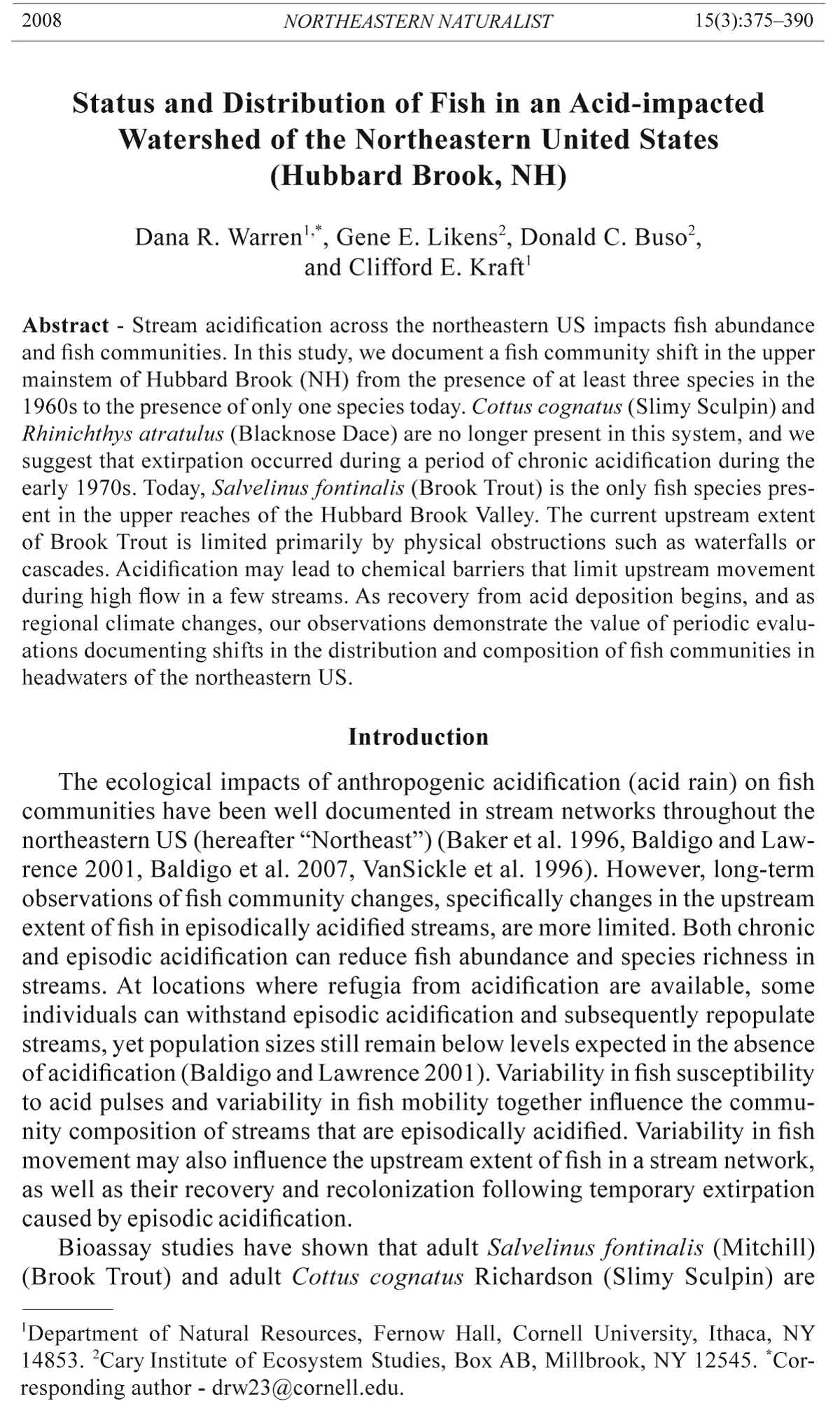
Access Journal Content
Open access browsing of table of contents and abstract pages. Full text pdfs available for download for subscribers.
Current Issue: Vol. 30 (3)
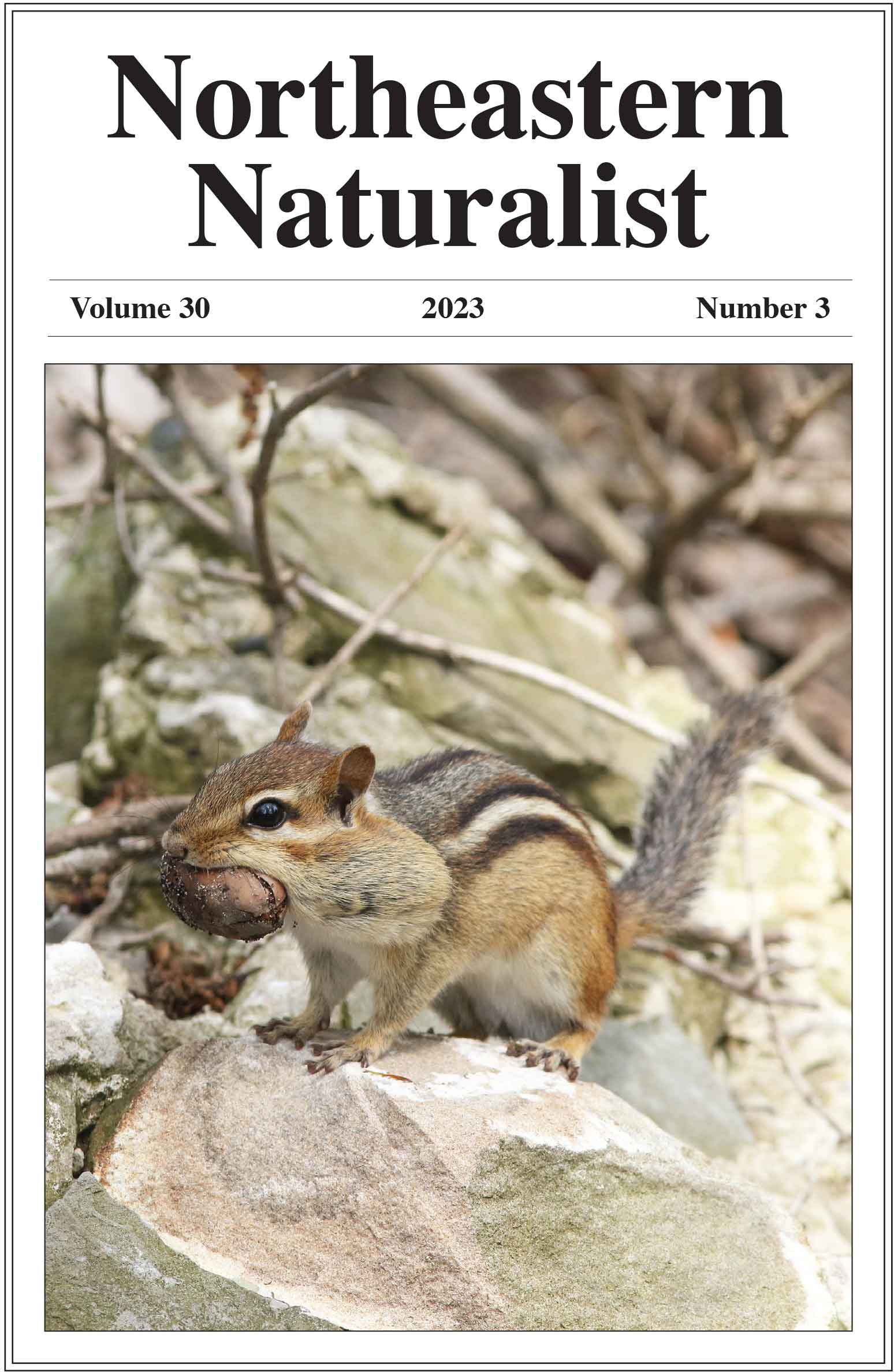
Check out NENA's latest Monograph:
Monograph 22
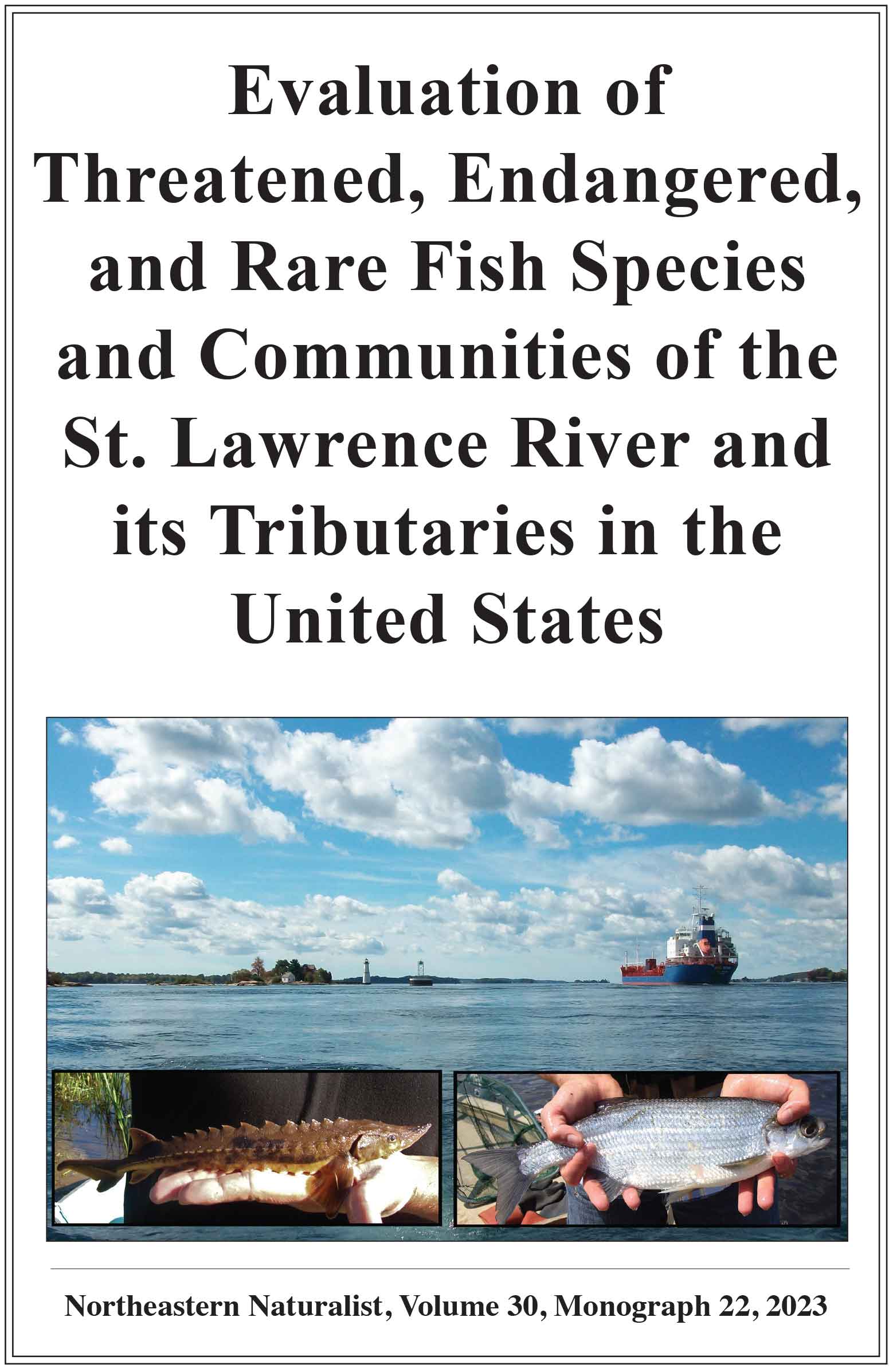
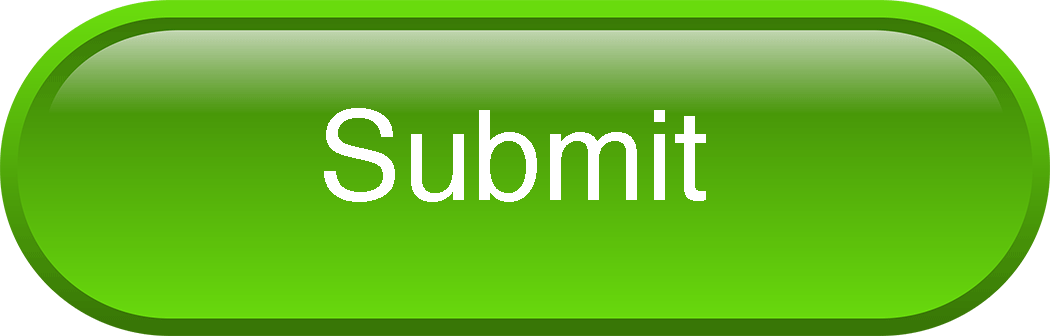
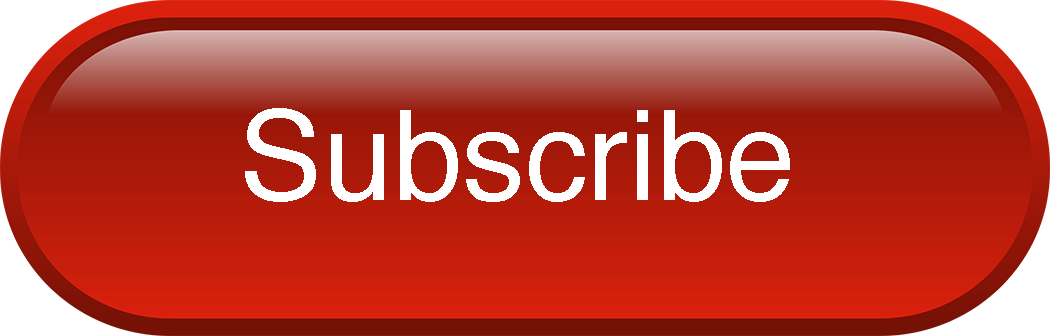
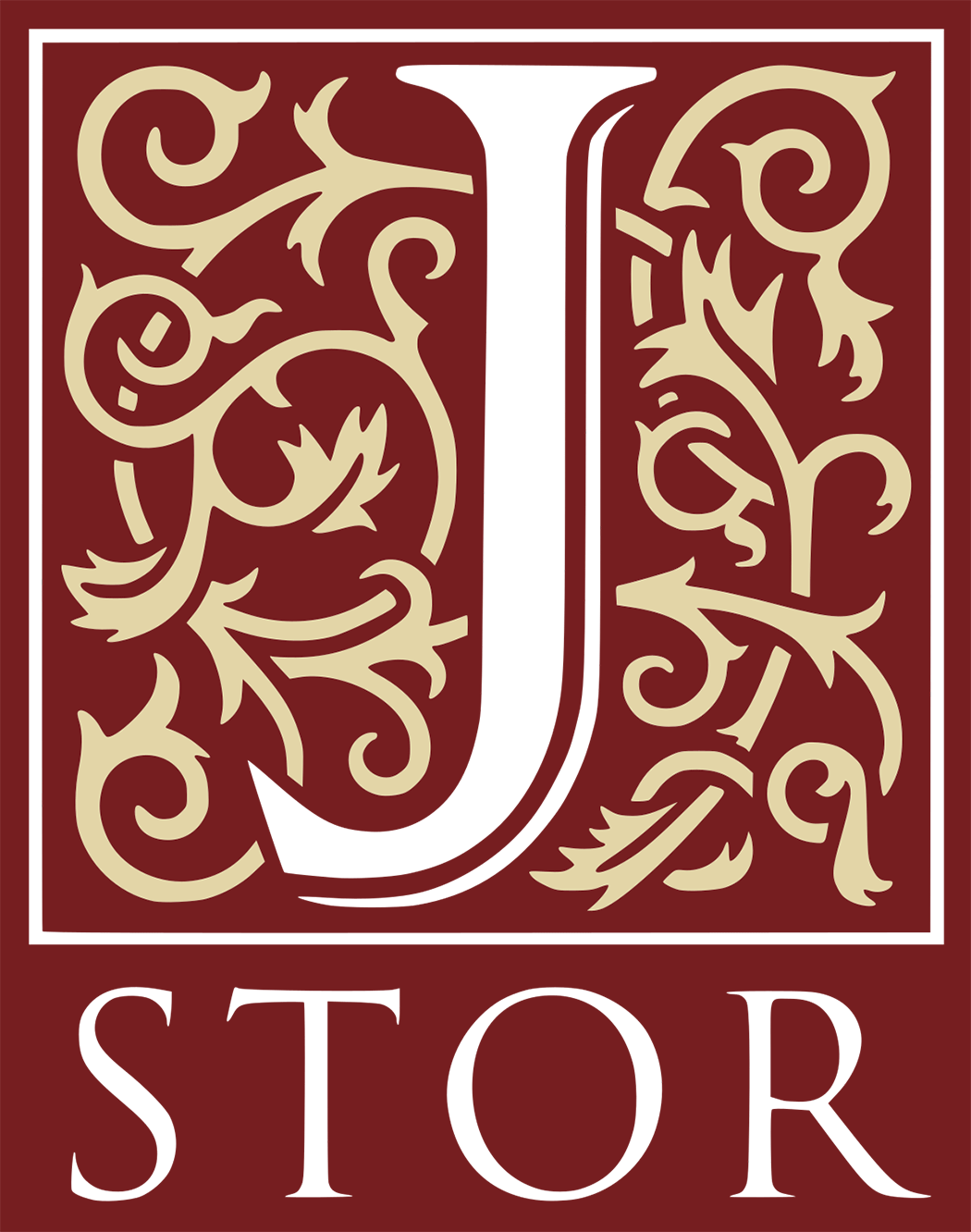


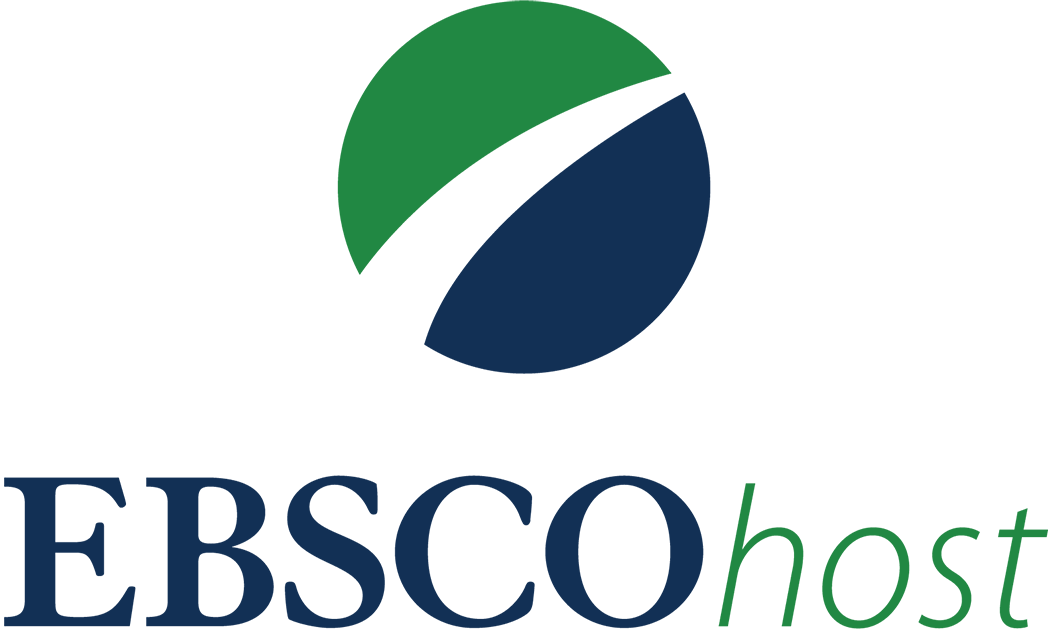

2008 NORTHEASTERN NATURALIST 15(3):375–390
Status and Distribution of Fish in an Acid-impacted
Watershed of the Northeastern United States
(Hubbard Brook, NH)
Dana R. Warren1,*, Gene E. Likens2, Donald C. Buso2,
and Clifford E. Kraft1
Abstract - Stream acidification across the northeastern US impacts fish abundance
and fish communities. In this study, we document a fish community shift in the upper
mainstem of Hubbard Brook (NH) from the presence of at least three species in the
1960s to the presence of only one species today. Cottus cognatus (Slimy Sculpin) and
Rhinichthys atratulus (Blacknose Dace) are no longer present in this system, and we
suggest that extirpation occurred during a period of chronic acidification during the
early 1970s. Today, Salvelinus fontinalis (Brook Trout) is the only fish species present
in the upper reaches of the Hubbard Brook Valley. The current upstream extent
of Brook Trout is limited primarily by physical obstructions such as waterfalls or
cascades. Acidification may lead to chemical barriers that limit upstream movement
during high flow in a few streams. As recovery from acid deposition begins, and as
regional climate changes, our observations demonstrate the value of periodic evaluations
documenting shifts in the distribution and composition of fish communities in
headwaters of the northeastern US.
Introduction
The ecological impacts of anthropogenic acidification (acid rain) on fish
communities have been well documented in stream networks throughout the
northeastern US (hereafter “Northeast”) (Baker et al. 1996, Baldigo and Lawrence
2001, Baldigo et al. 2007, VanSickle et al. 1996). However, long-term
observations of fish community changes, specifically changes in the upstream
extent of fish in episodically acidified streams, are more limited. Both chronic
and episodic acidification can reduce fish abundance and species richness in
streams. At locations where refugia from acidification are available, some
individuals can withstand episodic acidification and subsequently repopulate
streams, yet population sizes still remain below levels expected in the absence
of acidification (Baldigo and Lawrence 2001). Variability in fish susceptibility
to acid pulses and variability in fish mobility together influence the community
composition of streams that are episodically acidified. Variability in fish
movement may also influence the upstream extent of fish in a stream network,
as well as their recovery and recolonization following temporary extirpation
caused by episodic acidification.
Bioassay studies have shown that adult Salvelinus fontinalis (Mitchill)
(Brook Trout) and adult Cottus cognatus Richardson (Slimy Sculpin) are
1Department of Natural Resources, Fernow Hall, Cornell University, Ithaca, NY
14853. 2Cary Institute of Ecosystem Studies, Box AB, Millbrook, NY 12545. *Corresponding
author - drw23@cornell.edu.
376 Northeastern Naturalist Vol. 15, No. 3
among the most acid-tolerant fishes found in North Temperate Zone streams
and lakes (Gagen et al. 1993, VanSickle et al. 1996). Although Brook Trout
often persist in acid-stressed systems (summer pH as low as 4.6; D.R. Warren,
pers. observ.), Slimy Sculpin are frequently absent from episodically
acidified systems (Kaeser and Sharpe 2001). This pattern could be a result
of more limited movement by sculpin (and thus reduced access to refuges)
as well as greater susceptibility of juvenile Slimy Sculpin to acidification. In
Pennsylvania streams, Kaeser and Sharpe (2001) found that acid conditions
in spring reduced Slimy Sculpin reproductive success such that even when
adults survived episodic acidification, juvenile mortality could still lead to
the eventual local extirpation of the species over time.
Brook Trout are often the only species found in the uppermost reaches
of buffered and acidified streams in the Northeast. The upstream extent
of Brook Trout distribution in headwater streams therefore often defines
the boundary between the fish and fishless sections of a stream system. As
an apex predator in these headwaters, the presence or absence of fish has
the potential to influence a broad suite of ecological interactions. Studies
in Rocky Mountain streams in the western US found that fishless streams
have substantially different ecological conditions than streams containing
fish (McIntosh and Peckarsky 1996, McIntosh et al. 2002). In northeastern
streams, trout presence has been linked to reduced juvenile salamander
abundances (Lowe and Bolger 2002). In addition to these influences on
stream biotic interactions, many forest management regulations are designed
to protect fish resources in streams within forested watersheds (Cole et al.
2006). Knowing the upstream extent of fish distribution can be important
in determining biotic impacts from acid stress and in determining potential
refuge provided by specific stream locations.
In well-buffered streams, Brook Trout colonization during invasions
or following natural extirpation events can be rapid (Peterson and Fausch
2003, Roghair et al. 2002); however, in systems subject to episodic acidifi-
cation the rate and extent of (re)colonization is limited. Under favorable pH
conditions, Brook Trout tend to move upstream during periods of elevated
discharge, when fish can circumvent barriers that are otherwise impassable
during low flow conditions (Gowan and Fausch 1996). In acid-stressed systems,
pH sometimes decreases during high flow, especially during spring
snowmelt (Driscoll et al. 2001; Likens et al. 2002, 2004). In an episodically
acidified stream in Pennsylvania, Gagen et al. (1994) found that fish moved
downstream rather than upstream during a high-flow event with associated
declines in stream pH. Thus, an acid pulse during high flow that would
otherwise provide an opportunity for upstream fish movement beyond lowflow, physical barriers may restrict the upstream distribution of fish. In areas
where acidification is not an issue (e.g., in western North America where
Brook Trout are invasive) and in laboratory experiments, Brook Trout are
able to recolonize upstream locations and pass large barriers during high
flow (Adams et al. 2000, Kondratieff and Myrick 2006). By contrast, in
many stream systems in the Northeast, fish distribution is likely to be limited
2008 D.R. Warren, G.E. Likens, D.C. Buso, and C.E. Kraft 377
by a combination of chemical barriers at high flow and physical barriers at
low flow. Therefore, the geomorphic barriers that define the upstream extent
of Brook Trout distributions may be smaller than anticipated based on research
conducted in laboratories or in other regions.
In the Pacific Northwest, Latterell et al. (2003) and Cole et al. (2006) found
that the upstream distributions of salmonids were primarily limited by steep
gradients and waterfalls. Studies in the Northeast have suggested that both
stream chemistry and physical barriers will limit upstream fish distribution in
areas subject to acidification (Baldigo and Lawrence 2001, Kocovsky and Carline
2005). However, no previous studies have surveyed and documented the
upstream extent of fish in headwater streams of eastern North America.
Sulfate deposition across the Northeast has declined over the last twentyfive years, and a concurrent but diminished decline in the amount of sulfate
in stream water has also been observed (Likens et al. 2002). The reduction
in stream sulfate concentrations has coincided with slight increases in stream
pH and in the acid neutralizing capacity (ANC) of many streams across the
region (Driscoll et al. 2001, Likens 2004, Yan et al. 2003). Reductions in acid
deposition and increases in stream pH may allow fish to recolonize some areas
from which they have been extirpated, especially if episodic acidification
is reduced enough to allow previous barriers to become passable. While the
impacts of acidification are expected to persist for many years, evaluations
of the current upstream extent of fish in headwater streams are necessary if
we are to determine future changes in fish distribution associated with the
potential for increased habitat availability.
The Hubbard Brook Valley (HBV) in central New Hampshire has been
widely used as a model system in documenting ecosystem impacts of acidification (Likens and Bormann 1995), but no studies to date have evaluated
fish communities or fish distributions throughout the HBV. In this study, we
(1) compile available historic information from field notes and limited early
survey work on fish in the stream network of the HBV, (2) document current
fish communities in this system, and (3) determine the upstream extent of fish
in this system and evaluate stream features at the fish distributional limits.
This information will allow us to determine the broader impacts of acidification
on fish communities in this system. Surveys of the upstream extent of fish
will also fill a knowledge gap regarding the features and limits to fish distribution
in headwater stream networks of the Northeast. Further, documenting the
current upstream extent of fish in this stream network will establish baseline
information for future research evaluating changes to fish distribution in response
to changing environmental conditions.
Study Site
The Hubbard Brook Valley is a fifth-order watershed located in the White
Mountain Region of New Hampshire and drains to the Pemigewasset River.
This study focuses on the portion of the watershed within the Experimental
Forest boundary and excludes nearby Mirror Lake, its outlet, and areas of
378 Northeastern Naturalist Vol. 15, No. 3
the watershed that have been subject to recent development. All streams
except Norris Brook (Fig. 1) enter the mainstem Hubbard Brook within the
Experimental Forest.
Detailed descriptions of the HBV can be found in Likens and Bormann
(1995), and Likens and Buso (2006). The work by Likens and Buso (2006)
is particularly relevant to this study because it documents low-flow water
chemistry levels every 100 m for all streams in the HBV. Stream flow in this
region is characterized by high discharge in the spring during snowmelt, low
flow during the summer, and a slight recharge in the fall. The mainstem Hubbard
Brook flows west to east and is dominated by large cobble and boulder
substrates in the lower section. The upper section of this mainstem river has
some boulder-dominated reaches, but also contains alluvial sections with
smaller gravel and cobble and slightly lower gradients. Headwater streams
are mid- to high gradient (5% to >20%), and substrates are dominated by
boulders and cobble. The HBV is underlain by igneous and highly metamorphosed
sedimentary bedrock, characterized by base-poor granites and schists.
Glacial till is widespread and highly variable in thickness, and soils are
generally thin, acidic spodosols. Forests are northern hardwood ecosystems,
with the conifer component more common on the ridge tops and on the north-
Figure 1. Map of the HBEF watershed indicating upstream extent of fish (only Brook
Trout in all but Norris Brook) in late summer of 2005 and 2007. Prior to 2003, no
fish were present in Falls Brook due to a physical barrier where the stream enters the
mainstem, but fish were subsequently introduced in an experiment that year. New
year classes were observed in 2005 and 2007 surveys. The upstream x on Canyon
Brook also represents fish that were introduced in the 2003 cage experiment. There
was no evidence of reproduction in this upper section in the 2005 survey.
2008 D.R. Warren, G.E. Likens, D.C. Buso, and C.E. Kraft 379
facing slope of the HBV (Likens and Bormann 1995). Stream chemistry is
variable among and within streams. As a rule, pH is lower in tributaries than
the mainstem river, and the buffering capacity of tributaries declines in an upstream
direction (Likens and Buso 2006). The mainstem maintains consistent
pH relative to its tributaries (Likens and Buso 2006). Areas of groundwater
discharge were apparent in the valley-wide survey in some streams and are
characterized by increased pH, nitrate, and calcium (Likens and Buso 2006).
Mean pH, acid neutralizing capacity (ANC), nitrate, sulfate, and monomeric
aluminum for each tributary are reported in Table 1, as calculated from
Likens and Buso (2006). Chemical characteristics change in these tributaries
in an upstream direction from the mainstem to the headwaters. For example,
pH declined with increasing elevation. Conversely, and logically, aluminum
concentrations are generally lower near the mainstem than in the headwaters.
Other chemical features such as nitrate exhibit stream-specific characteristics,
with higher nitrate concentrations provided by groundwater inputs along
a specific reach, and other sections having low nitrate due to strong in-stream
uptake. Some ions such as sodium and chloride remain relatively constant
across tributary elevational gradients.
Methods
We first document historic records on the fish species present in the HBV
based on field notes by G. Likens in the late 1960s and a set of snorkel surveys
conducted on the mainstem in the late 1980s. In 2005, 2006, and 2007,
we conducted backpack electrofishing surveys to evaluate the presence or
Table 1. Mean water chemistry data from spring, summer, and fall water sampling efforts along
tributary streams throughout the Hubbard Brook Valley from 1997 to 2003. Tributaries are
listed in the order in which they enter the mainstem beginning downstream. The total number
of samples used to calculate mean pH, ANC, [SO4
-2] and [NO3
-] ranged from 11 to 20. Mean
monomeric Al+3 concentrations were calculated from two samples in each stream except Crazy
Brook, where four samples were used.
ANC SO4
-2 NO3
- Al+3 Ca+2 Mg+2 K+ Na+
Stream (μeq/L) pH (mg/L) (mg/L) (mg/L) (mg/L) (mg/L) (mg/L) (mg/L)
Norris Brook 59 6.37 5.01 0.030 0.005 2.11 0.47 0.39 1.68
Paradise Brook 40 5.97 4.35 0.270 0.005 1.41 0.37 0.40 1.46
Bear Brook 27 6.05 4.10 0.050 0.005 1.02 0.31 0.26 1.29
Falls Brook 17 5.80 4.06 0.110 0.013 1.02 0.29 0.21 1.11
Bagley Brook 6 5.35 4.21 0.140 0.015 0.89 0.30 0.23 1.02
Cascade Brook -27 4.61 4.35 0.180 0.190 0.73 0.24 0.20 0.82
Canyon Brook 21 5.68 4.04 0.210 0.015 1.04 0.34 0.19 0.98
Beaver Brook 9 5.42 3.92 0.140 0.035 1.04 0.29 0.20 0.91
Zig-Zag Brook 50 6.24 4.06 0.270 0.008 1.40 0.43 0.25 1.04
Split Brook 24 5.57 4.28 0.180 0.008 1.28 0.37 0.18 0.90
Kineo Brook 28 5.99 4.27 0.260 0.020 1.28 0.41 0.18 0.88
Crazy Brook 24 5.36 4.28 0.310 0.014 1.45 0.40 0.18 0.88
Steep Brook 7 5.49 4.25 0.340 0.020 1.07 0.35 0.14 0.64
Cushman Brook -7 5.02 4.73 0.080 0.080 0.92 0.29 0.11 0.67
Lost Brook 3 5.23 4.57 0.260 0.020 1.09 0.33 0.15 0.69
380 Northeastern Naturalist Vol. 15, No. 3
absence of fish in Hubbard Brook and its tributaries during later summer/
early fall. We documented the upstream extent of fish in each tributary and
recorded habitat features at the location where fish were last observed.
Historic records
Early observations of fish in the Hubbard Brook watershed were made as
visual assessments in the mainstem river and tributaries, primarily in Bear
Brook. Beginning in 1963, anecdotal observations of fish and other wildlife
(salamanders) were recorded in field notebooks. Given the nature of these
field observations, the presence of fish can be reliably documented; however,
without electrofishing or rotenone, the absence of fish, and therefore
the absolute upstream distribution of fish, cannot be explicitly determined.
Early fish surveys conducted by the State of New Hampshire in the White
Mountains using more invasive methods did not include Hubbard Brook, but
did encompass a number of comparable streams in the region (Hoover 1938;
Seamans 1959a, b). We use data from these surveys to infer likely historic
fish communities in our study-area streams.
In August 1988, a limited set of snorkel surveys were conducted to quantify
salmonid densities in two sections of the mainstem of Hubbard Brook by
two individuals counting fish either independently or together (Bryant 1989).
The upstream survey reach extended from the confluence of Cascade Brook,
upstream past the USFS bridge to the confluence of Kineo Brook (Fig. 1). The
downstream reach encompassed the mainstem river from the USFS forest
boundary upstream to the confluence of Falls Brook. A minimum of ten percent
of the area within each reach was included in the snorkel surveys.
Electrofishing survey
Electrofishing surveys in 2005, 2006, and 2007 were conducted using
a modified version of methods in Latterell et al. (2003). Fish presence/absence
was recorded using a backpack electroshocker with a field crew of two
people. Fish were either identified in the water or were captured briefly for
identification when fishes could not be clearly identified while remaining in
the water. Captured fish were returned to the water unharmed after identification.
In 2005, each tributary was surveyed in an upstream direction from its
confluence with the mainstem until fish were no longer observed. Surveys
were conducted a minimum of 100 m past the last fish observed to be sure
that fish were not present further upstream. The distance upstream from the
mainstem was measured with a field tape to the nearest of the 100-m markers
that were established during the 2001 valley-wide surveys (Likens and
Buso 2006). The 2006 electrofishing surveys were initiated at least 150 m
downstream from the furthest extent of fish found in that tributary during the
previous year, then surveys were continued upstream using the same methods
as in 2005. In all cases, fish were encountered when initiating surveys in
2006. The 2007 surveys were conducted beginning at the confluence with the
mainstem for each stream. All major tributaries within the Hubbard Brook
watershed were surveyed in 2007.
2008 D.R. Warren, G.E. Likens, D.C. Buso, and C.E. Kraft 381
After we surveyed a minimum of 100 m past the last fish observed, we
returned to the point where the last fish was observed and qualitatively
evaluated stream characteristics at that point. We noted if there were cascades,
waterfalls, stream sections with very steep gradients, a lack of water,
or “unknown barriers.” We noted an “unknown barrier” when fish were
absent beyond a given point in the stream with no obvious physical limits
to upstream distributions. This category included cases where the last fish
was located below a cascade of a size comparable to other cascades in that
system, which had clearly been passable for fish to reach that point. Potential
physical barriers to fish movement were documented and included waterfalls
or a series of two or three large cascades that reached heights of 2 m or more
over a short distance and with no pools below from which fish could jump
(e.g., Zig-Zag Brook). In some cases, individual barriers were not large but
cascades or granite outcroppings were frequent, leading to a consistent high
gradient reach (e.g., Falls Brook). In other streams, a lack of water was
clearly limiting upstream distributions, and in these cases, we noted that
“streambeds were dry.”
Results
Historic and current fish communities
Historic field notes and anecdotal evidence indicate that Brook Trout,
Slimy Sculpin, and at least one minnow species (Rhinichthys atratulus (Hemann)
[Blacknose Dace]) were observed in the mainstem of Hubbard Book
at least 7.4 km (4.6 miles) upstream from I-93 (near the USFS bridge) in the
1960s. Bear Brook is known to have contained both Brook Trout and Slimy
Sculpin at this time, based on field observations. Historic surveys of fishing
streams in the nearby Saco River watershed indicated that both sculpin and
dace were commonly encountered and at times were found in high abundance
(Seamans 1959a, b). In a survey of streams across the White Mountains,
Hoover (1938) found community shifts in an upstream direction from mainstem
rivers to headwater streams. In undisturbed (non-stocked, non-fished)
high-elevation streams, both Brook Trout and Slimy Sculpin were present in
seven of the eight streams sampled during these 1938 surveys.
Apart from a single Salmo salar L. (Atlantic Salmon), Brook Trout were
the only fish documented in the Hubbard Brook mainstem snorkel surveys
in 1988 (Bryant 1989). Atlantic Salmon fry were stocked into a few of
the HBEF tributaries in the mid- to late 1980s, but survival was low. With
regard to other fish species, Bryant (1989) specifically noted that “other species
were not observed in Hubbard Brook”; yet that survey was focused on
documenting the presence of salmonids, so few definitive conclusions can
be derived from this work.
Streamside visual surveys by D. Buso in 2001 indicated the presence of
Brook Trout in Norris Brook, Bear Brook, Zig-Zag Brook, and the mainstem
river. Extensive fish surveys throughout the HBEF stream network conducted
in late summer 2005 indicated that, with the exception of Norris Brook,
382 Northeastern Naturalist Vol. 15, No. 3
Brook Trout were the only fish present in HBEF tributaries. In all mainstem
surveys above the Hubbard Brook gorge (a series of waterfalls and cascades
up to 4-m in height), Brook Trout were the only fish present. Norris Brook
also contains Slimy Sculpin, but this stream enters below the gorge.
Fish distributions
Fish were absent from five (Cascade Brook, the west branch of Beaver
Brook, the tributary to the west branch of Kineo Brook, Steep Brook, and
Cushman Brook) of the nineteen streams we surveyed. Brook Trout were
the only fish found in the other streams, except for Norris Brook, which also
contained Slimy Sculpin. In most cases, the upstream distribution of fish appeared
to be constrained by a waterfall or other large physical barrier. In a
few cases, the mechanism for the upstream loss of fish was not immediately
apparent. Cascade Brook, which is a chronically acidified stream with a
mean summer pH of 4.6 (Likens and Buso 2006), contained no fish.
Between 2005 and 2006, the upstream extent of Brook Trout decreased
in most streams (the east branch of Zig-Zag Brook being the exception), but
these changes in distribution were minor (less than 50 m). Similarly, the upstream
extent of Brook Trout during late summer was relatively consistent from
2005 to 2007, with little change in most streams. The maximum increase
in upstream extent was 48 m in Paradise Brook. The largest decrease in
distribution (-162 m) occurred in Split Brook (Table 2, Fig. 1). Potential
physical barriers to late-summer fish distributions were apparent in slightly
more than half (11 of 19) of the surveyed streams. These potential physical
barriers included both waterfalls/cascades and a lack of surface flow. In one
stream, Canyon Brook, fish reached their upstream limit at a road culvert.
Table 2. Upstream extent of Brook Trout, change in distribution from 2005 to 2007 and presence
of potential physical barrier in each Hubbard Brook tributary from 2005, 2006, and 2007
fish surveys. Tributaries are listed in the order in which they enter the mainstem beginning
downstream (“-” = no data). For Canyon Brook, the first number represents the upstream extent
of fish from an introduction of adults in summer 2003 via a failed caging experiment, and the
second number represents the natural upstream extent of fish.
Change
Maximum upstream distance (m) 2005 to Potential
Stream 2005 2006 2007 2007 (m) barrier?
Norris Brook 1383 1378 1379 -4 Yes
Paradise Brook 419 - 467 48 No
Bear Brook 962 959 960 -2 No
Falls Brook 479* 436* 433* -46 Yes
Canyon Brook (W8) 430*/124 -*/125 -*/125 1 No**
Beaver Brook - East Branch 473 - 496 23 Yes
Zig-Zag Brook - East Branch (W7) 883 888 891 8 Yes
Zig-Zag Brook - West Branch 511 511 503 -8 Yes
Split Brook 720 - 558 -162 No**
Kineo East 521 515 522 1 Yes
Kineo West 719 700 711 -8 No
Crazy Brook - - 8 na Yes
Lost Brook 25 - 25 0 Yes
*Fish introduced in an earlier experiment.
**Potential low-flow barrier.
2008 D.R. Warren, G.E. Likens, D.C. Buso, and C.E. Kraft 383
This culvert was the only case where an anthropogenic feature was associated
with upstream distributions. The pool below the culvert is quite large,
and at high flows, the drop from the culvert to the pool is relatively small
(<0.5 m). Evidence from other streams in the system suggest that Brook
Trout are capable of passing a barrier of this size when there is an adequate
pool below the barrier; however, Canyon Brook is episodically acidified,
and as such, fish are likely to be moving downstream rather than upstream in
this system during high flow, when the culvert is most likely to be passable
(Gagen et al. 1994). Although identifying specific barriers to fish movement
may be subjective during low-flow conditions, potential low-flow barriers
can be clearly identified, as has been done in previous studies in the Pacific
Northwest (e.g., Cole et al. 2006, Latterell et al. 2003).
The upper extent of fish distribution in Canyon and Falls brooks was artificially enhanced in 2005. During July 2003, adult Brook Trout >100 mm
in length were held in cages placed in the upstream (and formerly fishless)
sections of Canyon and Falls Brooks as part of a study conducted to evaluate
the influence of trout on salamander behavior (B. Cosentino, University of
Illinois, Urbana, IL, pers. comm.). During this study, a flood broke the cages
in which fish were housed, and some escaped. Surveys in 2005 revealed that
large adult fish were still present at locations upstream from the farthest upstream
distribution observed in a brief preliminary survey conducted in 2003.
No fish were found in Falls Brook in 2003, presumably resulting from the
presence of a 100-m section of stream with a consistent gradient >25%. In our
2005, 2006, and 2007 surveys, we attributed the presence of large fish at these
upstream sections to the cage study rather than to natural movement. In addition
to the large adults, a distinct year-class of young-of-year trout was present
in Falls Brook in the newly colonized upstream section. In Canyon Brook,
only larger fish (>150 mm) were found in the area at which cages were placed.
In documenting the upstream extent of fish in Falls Brook in 2005, 2006, and
2007, we included the introduced fish because recruitment had clearly occurred.
In Canyon Brook, we focused the 2006 surveys only on the natural
upstream extent of fish, and a survey upstream in 2007 did not reveal any fish
remaining in the upstream section (Table 2).
Discussion
Recent surveys document that fish species have been extirpated from
Hubbard Brook and its tributaries since the 1960s. Slimy Sculpin and
Blacknose Dace have been lost from the mainstem of Hubbard Brook, and
Slimy Sculpin are no longer found in at least one of its tributaries. Brook
Trout are currently present in the mainstem of Hubbard Brook and in the
lower reaches of most tributaries (Fig. 1). It is likely that the native fish
community was still intact, although stressed, through the mid-1960s.
In 1970, stream acidification at HBEF peaked, and for a period of about
six years, streams in this region experienced chronic and acute acidification
(Fig. 2). We suggest that sculpin and dace were extirpated during this
384 Northeastern Naturalist Vol. 15, No. 3
period from tributaries in the HBV; based on historic surveys in the region,
it is likely that Slimy Sculpin have also been lost from similar stream
systems across the northeastern US. Calculations of acid neutralizing
capacity (ANC) at this time indicated that the cations present in streams
of the HBEF were not sufficient to balance anion loss (negative ANC’s;
Table 1, Fig. 2). Although inorganic monomeric aluminum was not measured
directly, it was most likely the balancing cation at this time (Buso et
Figure 2. Average annual values for stream pH, nitrate concentration, and ANC over
time in the reference watershed at Hubbard Brook (Watershed 6). The dashed box
encloses the period over which we hypothesize dace and sculpin were extripated
from the upper tributaries of the HBEF.
2008 D.R. Warren, G.E. Likens, D.C. Buso, and C.E. Kraft 385
al. 2000). High inorganic monomeric aluminum concentrations are the primary
cause of fish mortality during acidification (Baker et al. 1996, Booth
et al. 1988, Dietrich and Schlatter 1989, VanSickle et al. 1996).
Brook Trout are currently present in most tributaries in the upper
HBV, yet the upstream extent to which they are present varies. Along the
north-facing slope of the HBV, fish generally occurred further upstream
in tributaries that had greater buffering capacity (e.g., Zig-Zag and Kineo
brooks vs. Canyon Brook vs. Cascade Brook; Table 1, Fig. 1). However,
this trend was limited and was not constant across the south-facing slope
of the HBV. Potential physical barriers such as waterfalls, cascades, and
long, steep-gradient sections were the most common feature that appeared
to limit upstream fish distributions in HBEF in late summer (Table 2). In one
stream (Lost Brook), most of the streambed was dry during our late-summer
surveys. A few fish were found in remnant isolated pools near the mainstem,
but fish were absent from isolated pools at upstream locations. In a few
cases, fish distributional limits were attributed to a combination of physical
and chemical barriers. Kineo Brook and Bear Brook are all relatively well
buffered for a substantial portion of their length (Table 1), but the pH and
buffering capacity of these streams declines at higher elevations (Likens
and Buso 2006). In both Kineo-West and Bear Brook during 2005, 2006,
and 2007 and in Kineo-East during 2006, the location at which the last fish
was present was not associated with a likely physical barrier. Although the
fish observed farthest upstream were found below a small cascade in each of
these three streams, the cascade upstream was the same size or smaller than
those observed downstream. Therefore, cascades of this height could not be
considered strictly physical barriers to fish movement in these streams. In
these cases and in streams such as Split Brook and Cascade Brook where
there was no obvious barrier present, we suggest that the limits to upstream
fish distributions were a combination of physical and chemical barriers.
Latterell et al. (2003) found that (1) large shifts in stream gradient,
(2) declines in pool frequency, and (3) channel constrictions with limited water
were all key factors in limiting upstream distributions of Oncorhynchus
clarkii (Richardson) (Cutthroat) and Oncorhynchus mykiss (Walbaum) (Rainbow
Trout) in Cascade Mountain streams of the Pacific Northwest. Waterfalls
were noted as the primary barrier to the upper extent of fish distributions in
14% of the 21 unlogged streams that were surveyed in that study. In a similar
study, also in the Pacific Northwest, Cole et al. (2006) found that the dominant
feature determining the upper boundary to fish distribution was most often an
organic debris dam (48%), and the second-most common feature was a waterfall
or a cascade (30%). The potential transient nature of debris dams could
account for some year-to-year variability in fish distribution in their study.
Within the HBV, organic debris dams were never noted as key features potentially
limiting fish distributions. Although such dams become increasingly
common in the headwaters of HBEF tributaries, they do not appear to influence
fish distributions (Likens and Bilby 1982, Warren et al. 2007).
386 Northeastern Naturalist Vol. 15, No. 3
Knowing where headwater streams become fishless has important implications
for current and future research on stream ecosystem ecology in the
Northeast. In the western US, Brook Trout alter the behavior and life histories
of aquatic macroinvertebrates, algal growth, and resource patch dynamics
(McIntosh and Peckarsky 1996; McIntosh et al. 2002, 2004). In addition, across
eastern North America, the presence or absence of fish can influence stream amphibian
abundance and distribution (Lowe and Bolger 2002, Lowe et al. 2004).
Fish can interact with salamanders at multiple trophic levels; they can be predators
upon salamander larvae and competitors with some larger larvae and adults
for invertebrate prey (Lowe and Bolger 2002, Lowe et al. 2004).
Given the potential for fish to influence stream communities, large changes
in the upstream extent of fish distributions may have important ecological
implications in some streams. Changes of this magnitude are most likely to
occur when fish naturally or artificially expand beyond a previous barrier
(chemical or physical). Cole et al. (2006) evaluated changes in the upstream
extent of salmonids in 172 Pacific Northwest streams. When they observed
a change in the upper extent of fish between 2001 and 2002, the distance difference
between years (both upstream and downstream) was typically 50 m
or less (94% of the study streams), but distribution shifts >200 m in extent
were documented in eight of their streams (Cole et al. 2006). Changes in the
upstream extent of fish in HBV were far less evident than those observed
in the Pacific Northwest. In our surveys, shifts in the upper extent of fish
distribution from 2005 to 2006 and 2005 to 2007 were relatively short.
Changes within the northern forest ecosystem including northern forest
streams will influence the status of trout and other fish species in HBV and
elsewhere across the region. The 2005, 2006, and 2007 surveys establish
clear records that will allow future research documenting impacts of natural
changes, such as continued forest development or periodic disturbances such
as the 1998 ice storm, as well as changes caused by anthropogenic activities
including forest management, fish stocking, climate change, and continued
acidic deposition.
Forests in the Hubbard Brook watershed are at or near maturity, at least
in terms of biomass accumulation (Fahey et al. 2005), but the streams
may not yet be near a “mature” state. As forests in the HBV and across the
northern forest region continue to progress toward old-growth status, wood
loading, pools, and light dynamics in associated streams will increase
(Keeton et al. 2007; D.R. Warren, unpubl. data). These changes have the
potential to influence fish abundance (through increases in habitat) and primary
production. Forest management and forest disturbances that slow or
delay forest maturation can also influence fish abundance and productivity
in headwater systems (Nislow and Lowe 2006). Logging by clear cutting
can cause nitrate pulses in stream water (e.g., Likens et al. 1970) that are
clearly lethal to fish in associated streams, and these would be expected to
decrease both fish abundance and fish distribution (Baldigo et al. 2005).
If fish were to survive or recolonize following these pulses, and if stream
temperatures were to remain below lethal levels, production in the post2008
D.R. Warren, G.E. Likens, D.C. Buso, and C.E. Kraft 387
harvest systems would increase well above levels observed in mature forest
streams (Burton and Likens 1973, Nislow and Lowe 2006).
Climate projections for the Northeast indicate the potential for increased
temperatures in the summer and decreased snowpack in the winter (Hayhoe
et al. 2007). Current limitations to the upstream distribution of fish in the
HBV appear to be driven primarily by physical barriers and episodic acidifi-
cation, but increased anchor ice in winter (as a result of reduced snowpack)
and greater areas of dry stream bed in summer are important factors to
consider in evaluating future limitations to the distribution of fish in the
Hubbard Brook ecosystem. A modeling study from the southern Appalachian
Mountains also suggests that the downstream distribution of trout will also
decline with increasing temperatures across eastern North America (Flebbe
et al. 2006). Under these scenarios, trout distributions may be compressed
with reductions in available habitat both upstream and downstream.
Streams in the HBV and across the northern forest region are experiencing
increases in pH and ANC; however, recovery from acidification in
headwater streams requires more than just an increase in mean pH. The
re-establishment of natural fish communities is a key component of ecosystem
recovery. The current water chemistry conditions in some of the HBEF
tributaries and in the mainstem suggest that there may be potential for fish
other than Brook Trout (e.g., Slimy Sculpin) to survive and reproduce if
they were to be re-introduced to the system. It is important to recognize,
however, that the streams in this watershed continue to be influenced by the
effects of long-term base-cation loss, and pH and ANC have not yet returned
to pre-disturbance conditions (Lawrence 2002, Likens et al. 1998, Palmer et
al. 2004). With the loss of base cations from the soils of the HBEF (Likens
et al. 1998), the chemical stability of watersheds has declined, and the dilute
waters in these systems may be even more sensitive to acidic deposition as
a result. Decreases in the capacity of the forest ecosystem to buffer episodic
events will continue to result in episodic depressions in stream pH and associated
spikes in monomeric aluminum during acidicification events. In
addition, recolonization and establishment of the original fish community in
the upper section of mainstem Hubbard Brook and its associated tributaries
is unlikely to occur at locations upstream from large fish barriers, even with
changes in chemical conditions in streams that would otherwise allow for
fish survival. The recovery of native fish communities in headwater systems
across the Northeast will likely take many years to occur and will rely not
only upon adequate stream conditions but also upon chance recolonization
events that move fish above otherwise impassable barriers.
Acknowledgments
We thank Jason Demers, Allison Fritts-Penniman, Madeleine Mineau, Jared
Nunery, Jason Robinson, and Hannah Shayler for help with fieldwork and for reviewing
early drafts of this manuscript. We thank Thomas M. Burton and Stuart Fisher
for their historical perspectives on the HBEF tributaries. This project was funded in
part by the Cornell Biogeochemistry and Environmental Biocomplexity IGERT (NSF
DGE-0221658), by the EPA Science to Achieve Results (STAR) graduate research
388 Northeastern Naturalist Vol. 15, No. 3
fellowship program, and by the NSF LTREB and the A.W. Mellon Foundation to G.E.
Likens. The Hubbard Brook Experimental Forest is operated and maintained by the
US Forest Service, Newton Square, PA. This publication does not reflect the view of
any sponsoring agency.
Literature Cited
Adams, S.B., C.A. Frissell, and B.E. Rieman. 2000. Movements of nonnative Brook
Trout in relation to stream channel slope. Transactions of the American Fisheries
Society 129:623–638.
Baker, J.P., J. VanSickle, C.J. Gagen, D.R. DeWalle, W.E. Sharpe, R.F. Carline, B.P.
Baldigo, P.S. Murdoch, D.W. Bath, W.A. Kretser, H.A. Simonin, and P.J. Wigington.
1996. Episodic acidification of small streams in the northeastern United
States: Effects on fish populations. Ecological Applications 6:422–437.
Baldigo, B.P., and G.B. Lawrence. 2001. Effects of stream acidification and habitat
on fish populations of a North American river. Aquatic Sciences 63:196–222.
Baldigo, B.P., P.S. Murdoch, and D.A. Burns. 2005. Stream acidification and mortality
of Brook Trout (Salvelinus fontinalis) in response to timber harvest in
Catskill Mountain watersheds, New York, USA. Canadian Journal of Fisheries
and Aquatic Sciences 62:1168–1183.
Baldigo, B.P., G. Lawrence, and H. Simonin. 2007. Persistent mortality of Brook
Trout in episodically acidified streams of the southwestern Adirondack Mountains,
New York. Transactions of the American Fisheries Society 136:121–134.
Booth, C.E., D.G. McDonald, B.P. Simons, and C.M. Wood. 1988. Effects of aluminum
and low pH on net ion fluxes and ion balance in the Brook Trout (Salvelinus
fontinalis). Canadian Journal of Fisheries and Aquatic Sciences 45:1563–1574.
Bryant, M.D. 1989. Salmonid densities and habitat characteristics of two New
Hampshire Streams. US Forest Service, Broomall, PA. USDA FS report 6200-28
(7-82).
Burton, T.M., and G.E. Likens. 1973. Effect of strip-cutting on stream temperatures
in Hubbard Brook Experimental Forest, New Hampshire. Bioscience 23:433–
435.
Buso, D.C., G.E. Likens, and J.S. Eaton. 2000. Chemistry of precipitation, streamwater,
and lakewater from the Hubbard Brook Ecosystem Study: A record of sampling
protocols and analytical procedures. US Forest Service, Newtown Square,
PA. USDA-FS NE-275.
Cole, M.B., D.M. Price, and B.R. Fransen. 2006. Change in the upper extent of fish
distribution in eastern Washington streams between 2001 and 2002. Transactions
of the American Fisheries Society 135:634–642.
Dietrich, D., and C. Schlatter. 1989. Aluminum toxicity to Rainbow Trout at low pH.
Aquatic Toxicology 15:197–212.
Driscoll, C.T., G.B. Lawrence, A.J. Bulger, T.J. Butler, C.S. Cronan, C. Eagar, K.F.
Lambert, G.E. Likens, J.L. Stoddard, and K.C. Weathers. 2001. Acidic deposition
in the northeastern United States: Sources and inputs, ecosystem effects, and
management strategies. Bioscience 51:180–198.
Fahey, T.J., T.G. Siccama, C.T. Driscoll, G.E. Likens, J. Campbell, C.E. Johnson,
J.J. Battles, J.D. Aber, J.J. Cole, M.C. Fisk, P.M. Groffman, S.P. Hamburg, R.T.
Holmes, P.A. Schwarz, and R.D. Yanai. 2005. The biogeochemistry of carbon at
Hubbard Brook. Biogeochemistry 75:109–176.
Flebbe, P.A., L.D. Roghair, and J.L. Bruggink. 2006. Spatial modeling to project
southern Appalachian trout distribution in a warmer climate. Transactions of the
American Fisheries Society 135:1371–1382.
2008 D.R. Warren, G.E. Likens, D.C. Buso, and C.E. Kraft 389
Gagen, C.J., W.E. Sharpe, and R.F. Carline. 1993. Mortality of Brook Trout, Mottled
Sculpins, and Slimy Sculpins during acidic episodes. Transactions of the American
Fisheries Society 122:616–628.
Gagen, C.J., W.E. Sharpe, and R.F. Carline. 1994. Downstream movement and
mortality of Brook Trout (Salvelinus fontinalis) exposed to acidic episodes in
streams. Canadian Journal of Fisheries and Aquatic Sciences 51:1620–1628.
Gowan, C., and K.D. Fausch. 1996. Long-term demographic responses of trout populations
to habitat manipulation in six Colorado streams. Ecological Applications
6:931–946.
Hayhoe, K., C. Wake, T.G. Huntington, L. Luo, M.D. Schwartz, J. Sheffield, E.
Wood, B. Anderson, J. Bradbury, A. DeGaetano, T.J. Troy, and D. Wolfe. 2007.
Past and future changes in climate and hydrological indicators in the US Northeast.
Climate Dynamics 28:381–407.
Hoover, E.E. 1938. Fish populations of primitive Brook Trout streams of northern
New Hampshire. Pp. 485–496, In Transactions of the Third North American
Wildlife Conference. American Wildlife Institute, Washington, DC.
Kaeser, A.J., and W.E. Sharpe. 2001. The influence of acidic runoff episodes on
Slimy Sculpin reproduction in Stone Run. Transactions of the American Fisheries
Society 130:1106–1115.
Keeton, W.S., C.E. Kraft, and D.R. Warren. 2007. Mature and old-growth riparian
forests: Structure, dynamics, and effects on Adirondack stream habitats. Ecological
Applications 17:852–868.
Kocovsky, P.M., and R.F. Carline. 2005. Stream pH as an abiotic gradient influencing
distributions of trout in Pennsylvania streams. Transactions of the American
Fisheries Society 134:1299–1312.
Kondratieff, M.C., and C.A. Myrick. 2006. How high can Brook Trout jump? A
laboratory evaluation of Brook Trout jumping performance. Transactions of the
American Fisheries Society 135:361–370.
Latterell, J.J., R.J. Naiman, B.R. Fransen, and P.A. Bisson. 2003. Physical constraints
on trout (Oncorhynchus spp.) distribution in the Cascade Mountains: A
comparison of logged and unlogged streams. Canadian Journal of Fisheries and
Aquatic Sciences 60:1007–1017.
Lawrence, G.B. 2002. Persistent episodic acidification of streams linked to acid rain
effects on soil. Atmospheric Environment 36:1589–1598.
Likens, G.E. 2004. Some perspectives on long-term biogeochemical research from
the Hubbard Brook ecosystem study. Ecology 85:2355–2362.
Likens, G. E., and R. E. Bilby. 1982. Development maintenance and role of organic
debris dams in New England streams. Pp. 122–128, In F.J. Swanson, R.J. Janda,
T. Dunne, and D.N. Swanston (Eds.). Sediment Budgets and Routing in Forested
Drainage Basins. US Forest Service, Pacific Northwest, Forest and Range Experiment
Station, Portland, OR. General Technical Report PNW-141. 165 pp.
Likens, G.E., and H.F. Bormann. 1995. Biogeochemistry of a Forested Ecosystem.
Second Edition. Springer-Verlag, New York, NY. 198 pp.
Likens, G.E., and D.C. Buso. 2006. Variation in streamwater chemistry throughout
the Hubbard Brook Valley. Biogeochemistry 78:1–30.
Likens, G.E., F.H. Bormann, N.M. Johnson, D.W. Fisher, and R.S. Pierce. 1970. Effects
of forest cutting and herbicide treatment on nutrient budgets in the Hubbard
Brook watershed ecosystem. Ecological Monographs 40:23–47.
Likens, G.E., C.T. Driscoll, D.C. Buso, T.G. Siccama, C.E. Johnson, G.M. Lovett,
T.J. Fahey, W.A. Reiners, D.F. Ryan, C.W. Martin, and S.W. Bailey. 1998. The
biogeochemistry of calcium at Hubbard Brook. Biogeochemistry 41:89–173.
390 Northeastern Naturalist Vol. 15, No. 3
Likens, G.E., C.T. Driscoll, D.C. Buso, M.J. Mitchell, G.M. Lovett, S.W. Bailey,
T.G. Siccama, W.A. Reiners, and C. Alewell. 2002. The biogeochemistry of sulfur
at Hubbard Brook. Biogeochemistry 60:235–316.
Likens, G.E., D.C. Buso, B.K. Dresser, E.S. Bernhardt, R.O. Hall, K.H. Macneale,
and S.W. Bailey. 2004. Buffering an acidic stream in New Hampshire with a
silicate mineral. Restoration Ecology 12:419–428.
Lowe, W.H., and D.T. Bolger. 2002. Local and landscape-scale predictors of salamander
abundance in New Hampshire headwater streams. Conservation Biology
16:183–193.
Lowe, W.H., K.H. Nislow, and D.T. Bolger. 2004. Stage-specific and interactive effects
of sedimentation and trout on a headwater stream salamander. Ecological
Applications 14:164–172.
McIntosh, A.R., and B.L. Peckarsky. 1996. Differential behavioural responses of
mayflies from streams with and without fish to trout odor. Freshwater Biology
35:141–148.
McIntosh, A.R., B.L. Peckarsky and B.W. Taylor. 2002. The influence of predatory
fish on mayfly drift: Extrapolating from experiments to nature. Freshwater Biology
47:1497–1513.
McIntosh, A.R., B.L. Peckarsky, and B.W. Taylor. 2004. Predator-induced resource
heterogeneity in a stream food web. Ecology 85:2279–2290.
Nislow, K.H., and W.H. Lowe. 2006. Influences of logging history and riparian forest
characteristics on macroinvertebrates and Brook Trout (Salvelinus fontinalis) in
headwater streams (New Hampshire, USA). Freshwater Biology 51:388–397.
Palmer, S. M., C.T. Driscoll, and C.E. Johnson. 2004. Long-term trends in soil solution
and stream water chemistry at the Hubbard Brook Experimental Forest:
Relationship with landscape position. Biogeochemistry 68:51–70.
Peterson, D.P., and K.D. Fausch. 2003. Upstream movement by nonnative Brook
Trout (Salvelinus fontinalis) promotes invasion of native Cutthroat Trout (Oncorhynchus
clarki) habitat. Canadian Journal of Fisheries and Aquatic Sciences
60:1502–1516.
Roghair, C.N., C.A. Dolloff, and M.K. Underwood. 2002. Response of a Brook Trout
population and instream habitat to a catastrophic flood and debris flow. Transactions
of the American Fisheries Society 131:718–730.
Seamans, R.G. 1959a. Trout stream management investigations of the Swift River
watershed in Albany, New Hampshire, Survey Report No. 7. New Hampshire
Fish and Game Department, Concord, NH. pp. 40
Seamans, R.G. 1959b. Trout stream management investigations of the Saco River
watershed, Survey Report No. 9. New Hampshire Fish and Game Department,
Concord, NH. pp. 71
VanSickle, J., J.P. Baker, H.A. Simonin, B.P. Baldigo, W.A. Kretser, and W.E.
Sharpe. 1996. Episodic acidification of small streams in the northeastern United
States: Fish mortality in field bioassays. Ecological Applications 6:408–421.
Warren, D.R., E.S. Bernhardt, R.O.J. Hall, and G.E. Likens. 2007. Forest age, wood,
and nutrient dynamics in headwater streams of the Hubbard Brook Experimental
Forest, NH. Earth Surface Processes and Landforms 32:1154–1163.
Yan, N.D., B. Leung, W. Keller, S.E. Arnott, J.M. Gunn, and G.G. Raddum. 2003.
Developing conceptual frameworks for the recovery of aquatic biota from acidification. Ambio 32:165–169.