Camera Trapping of Carnivores: Trap Success Among
Camera Types and Across Species, and Habitat Selection
by Species, on Salt Pond Mountain, Giles County, Virginia
Marcella J. Kelly and Erika L. Holub
Northeastern Naturalist, Volume 15, Issue 2 (2008): 249–262
Full-text pdf (Accessible only to subscribers.To subscribe click here.)
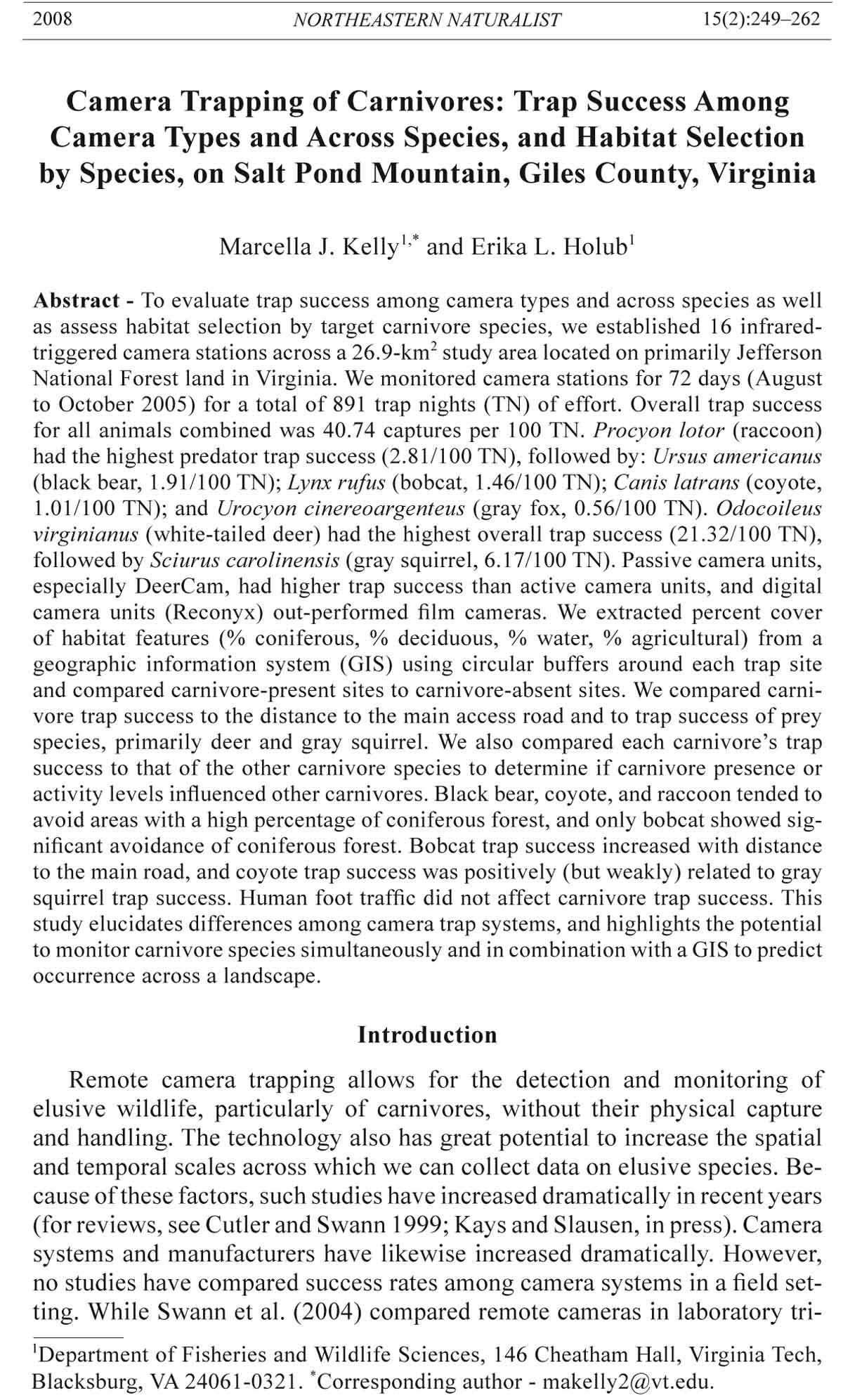
Access Journal Content
Open access browsing of table of contents and abstract pages. Full text pdfs available for download for subscribers.
Current Issue: Vol. 30 (3)
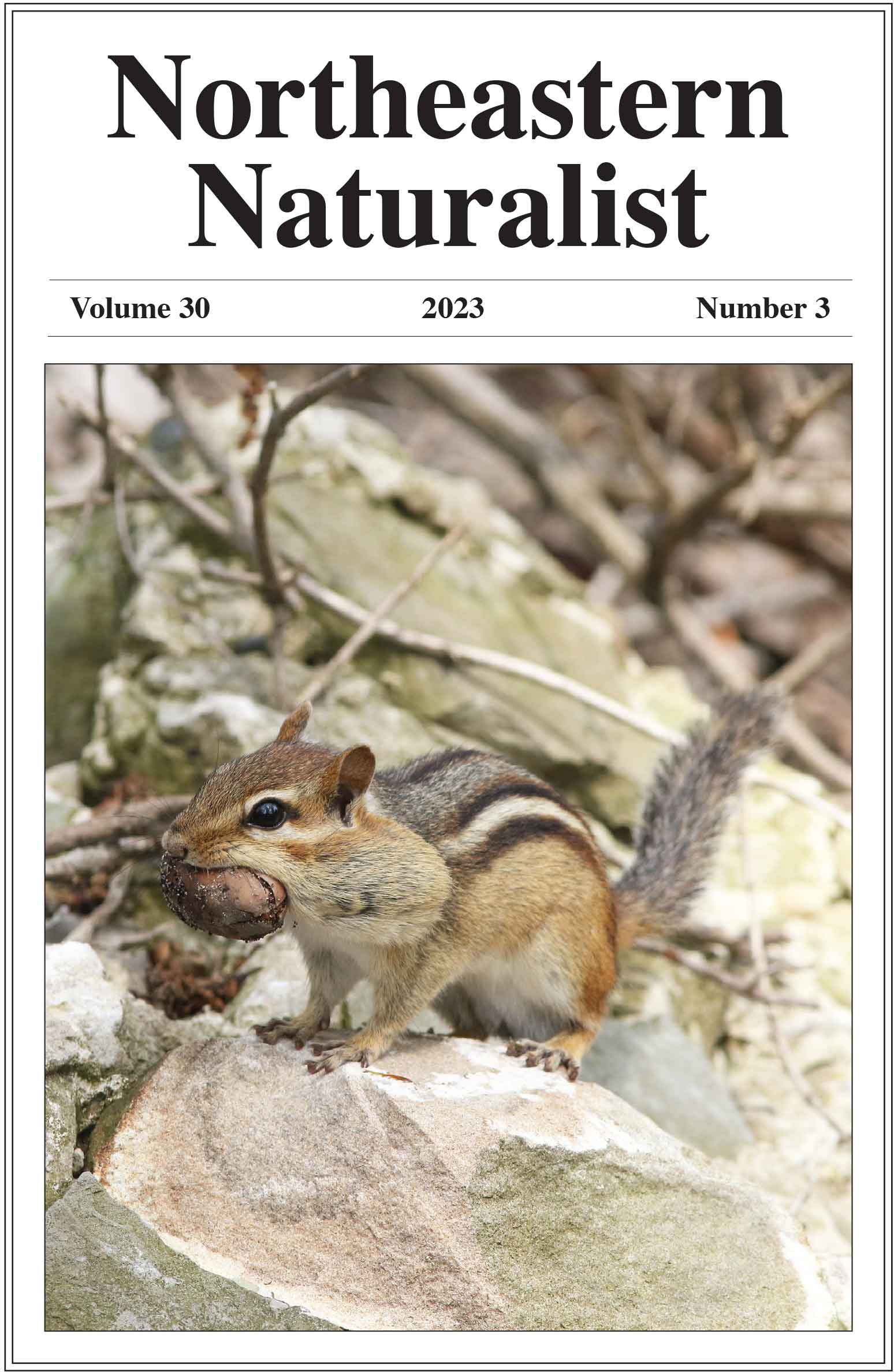
Check out NENA's latest Monograph:
Monograph 22
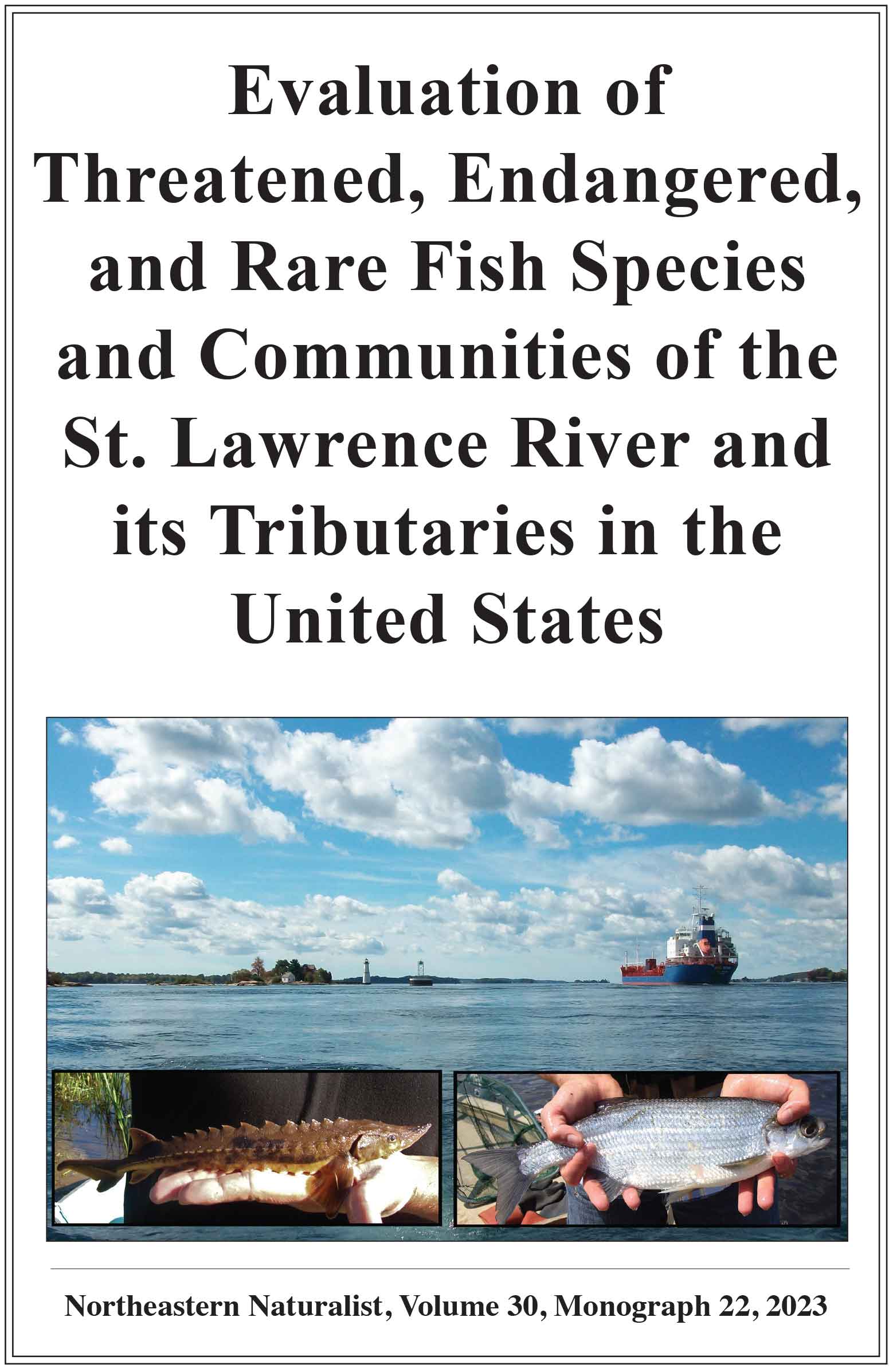
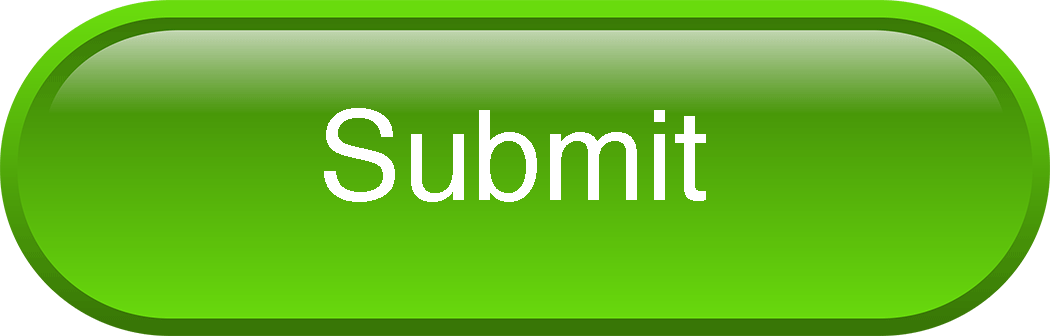
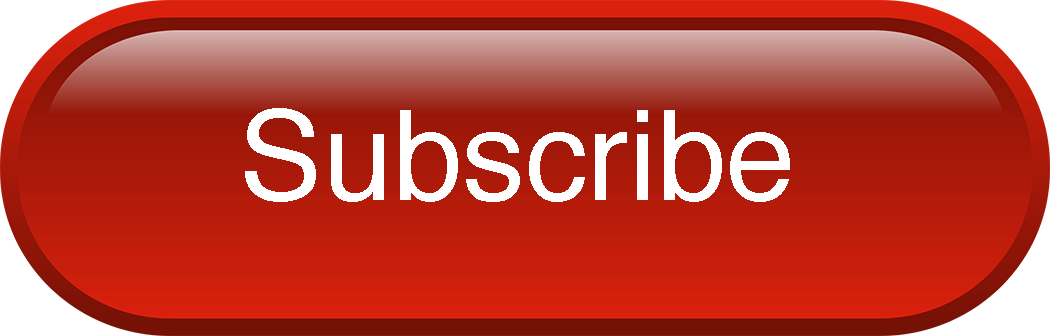
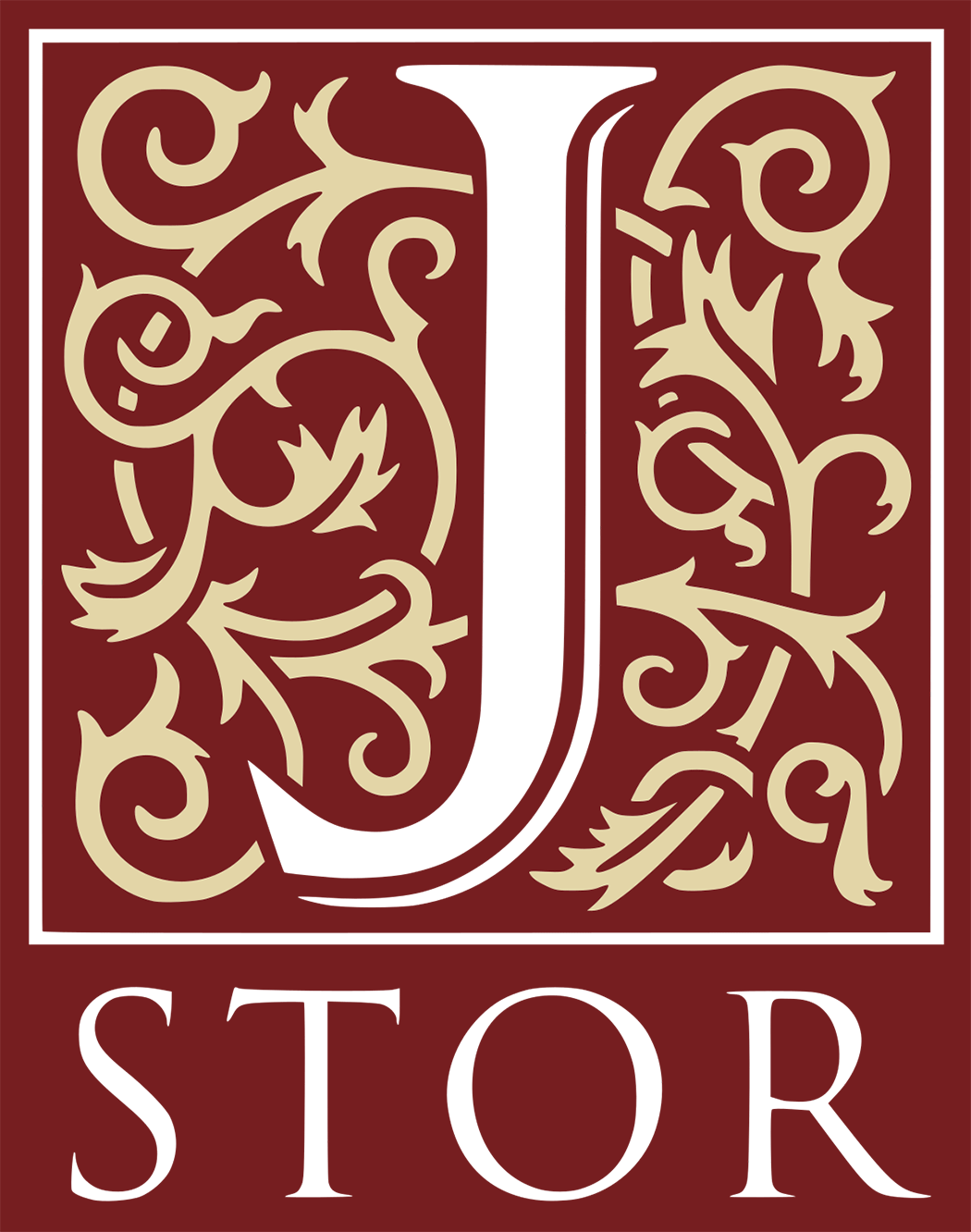


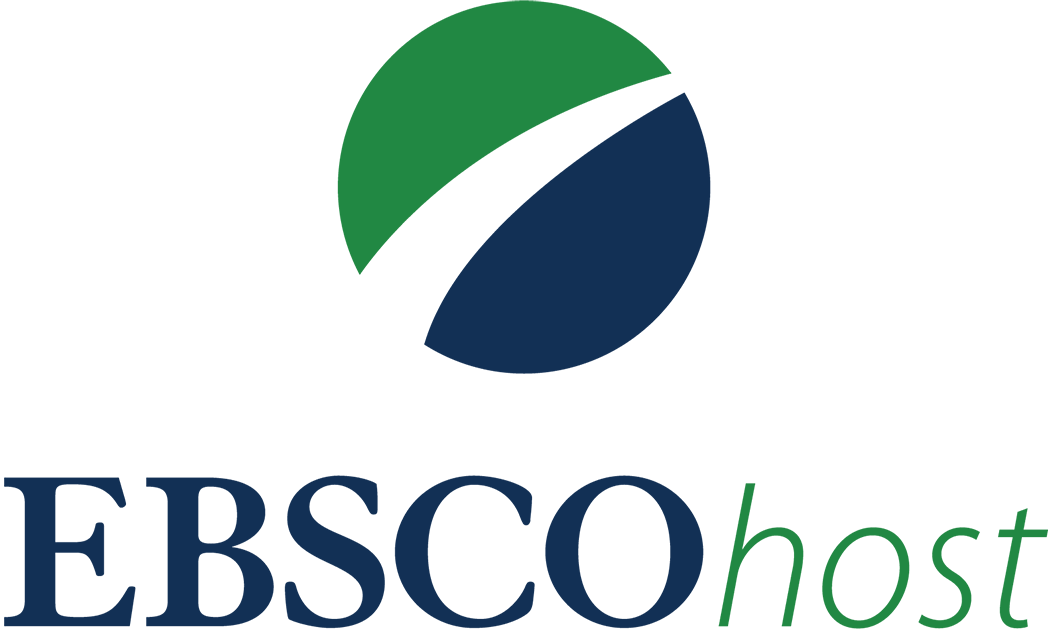

2008 NORTHEASTERN NATURALIST 15(2):249–262
Camera Trapping of Carnivores: Trap Success Among
Camera Types and Across Species, and Habitat Selection
by Species, on Salt Pond Mountain, Giles County, Virginia
Marcella J. Kelly1,* and Erika L. Holub1
Abstract - To evaluate trap success among camera types and across species as well
as assess habitat selection by target carnivore species, we established 16 infraredtriggered
camera stations across a 26.9-km2 study area located on primarily Jefferson
National Forest land in Virginia. We monitored camera stations for 72 days (August
to October 2005) for a total of 891 trap nights (TN) of effort. Overall trap success
for all animals combined was 40.74 captures per 100 TN. Procyon lotor (raccoon)
had the highest predator trap success (2.81/100 TN), followed by: Ursus americanus
(black bear, 1.91/100 TN); Lynx rufus (bobcat, 1.46/100 TN); Canis latrans (coyote,
1.01/100 TN); and Urocyon cinereoargenteus (gray fox, 0.56/100 TN). Odocoileus
virginianus (white-tailed deer) had the highest overall trap success (21.32/100 TN),
followed by Sciurus carolinensis (gray squirrel, 6.17/100 TN). Passive camera units,
especially DeerCam, had higher trap success than active camera units, and digital
camera units (Reconyx) out-performed film cameras. We extracted percent cover
of habitat features (% coniferous, % deciduous, % water, % agricultural) from a
geographic information system (GIS) using circular buffers around each trap site
and compared carnivore-present sites to carnivore-absent sites. We compared carnivore
trap success to the distance to the main access road and to trap success of prey
species, primarily deer and gray squirrel. We also compared each carnivore’s trap
success to that of the other carnivore species to determine if carnivore presence or
activity levels influenced other carnivores. Black bear, coyote, and raccoon tended to
avoid areas with a high percentage of coniferous forest, and only bobcat showed significant avoidance of coniferous forest. Bobcat trap success increased with distance
to the main road, and coyote trap success was positively (but weakly) related to gray
squirrel trap success. Human foot traffic did not affect carnivore trap success. This
study elucidates differences among camera trap systems, and highlights the potential
to monitor carnivore species simultaneously and in combination with a GIS to predict
occurrence across a landscape.
Introduction
Remote camera trapping allows for the detection and monitoring of
elusive wildlife, particularly of carnivores, without their physical capture
and handling. The technology also has great potential to increase the spatial
and temporal scales across which we can collect data on elusive species. Because
of these factors, such studies have increased dramatically in recent years
(for reviews, see Cutler and Swann 1999; Kays and Slausen, in press). Camera
systems and manufacturers have likewise increased dramatically. However,
no studies have compared success rates among camera systems in a field setting.
While Swann et al. (2004) compared remote cameras in laboratory tri-
1Department of Fisheries and Wildlife Sciences, 146 Cheatham Hall, Virginia Tech,
Blacksburg, VA 24061-0321. *Corresponding author - makelly2@vt.edu.
250 Northeastern Naturalist Vol. 15, No.2
als, camera performance must also be evaluated under the often difficult and
variable field conditions of rain, humidity, wind, and snow. We evaluated four
commonly available camera types.
Most remote camera studies focus on one or two target species and yet
often photographs are obtained of multiple species. In fact, the information
on non-targets often far outweighs the information obtained on the target species.
Careful camera placement can further increase the information obtained
on non-target species while still capturing target species. Because of the large
number of photographs obtained in remote camera studies, this “extra” photographic
information often is filed away and not recorded or analyzed. Simple
metrics such as trap success for each species, however, may prove extremely
valuable for wildlife monitoring generally or in relationship to the target species.
While debate continues over whether trap success can be used as an index
of abundance (Anderson 2001, 2003; Carbone et al. 2001, 2002; Engeman
2003; Jennelle et al. 2002; O’Brien et al. 2003), trap-success data across species
can, at the least, lead to hypotheses on species occurrence in relation to
habitat variables and/or other species. Recent advances in occupancy modeling
also can allow us to use presence-absence data obtained from sequential,
repeated, remote camera surveys to generate detection probabilities and
produce more reliable estimates of species’ relative abundance or areas occupied
(MacKenzie and Royle 2005, MacKenzie et al. 2003, Royle and Nichols
2003). Therefore, the value of remote camera data will only increase through
time, and effort should be expended at the onset to enter non-target species
data into a usable format for future analyses.
We targeted all medium- to large-sized carnivores believed to occur in the
study site: Ursus americanus (black bear); Lynx rufus (bobcat); Canis latrans
(coyote); Vulpes vulpes (red fox); Urocyon cinereoargenteus (gray fox); Procyon
lotor (raccoon); and Mephitis mephitis Schreber (skunk). We calculated
trap success for all species, including the non-carnivores, and present this
information as a comparative guide for other researchers on the amount of
effort needed to capture each. We combined our information on trap success
and presence-absence with a geographic information system (GIS) to evaluate
habitat selection by the carnivores. We also examined whether carnivore trap
success was influenced by trap success of other species.
Methods
The study area is located around Mountain Lake Biological Station
(MLBS) on Salt Pond Mountain, Giles County, VA (37.375556ºN,
80.522222ºW) and is primarily within the Jefferson National Forest, with
some on privately owned land (Fig. 1). MLBS is surrounded by deciduous
hardwood forest with stands of Pinus strobus L. (white pine), and Tsuga
canadensis L. (Carr.) (eastern hemlock), at an elevation of 1160 m (3806
ft). Other habitats include mountain streams, successional meadows, a large
natural lake, ponds, rocky ridges, sphagnum bogs, and stands of Picea rubens
Sarg. (red spruce).
2008 M.J. Kelly and E.L. Holub 251
We established 15 camera stations, of which one was later moved, and the
new location became site 16 in Figure 1. Trap sites were systematically spaced
1 to 1.5 km apart across the study site and placed on existing hiking trails,
game trails, and forest service roads. We cleared understory growth at sites to
minimize false triggering and allow for unobstructed photographs. We chose
sites with natural “funnels” to force animals in front of cameras at a distance of
approximately 3–4 m from the lens. We used no lures. Cameras were mounted
30–40 cm above the ground, and care was taken to ensure that each camera
would trigger for an animal as small as a bobcat or raccoon, as well as for a
deer or bear. When possible, we locked cameras to trees with braided steel
cables and padlocks to prevent theft.
We used both active and passive infrared-triggered cameras to survey and
evaluate trap success for all animals captured, as well as to determine the effectiveness
of different camera types. Active systems consisted of two units: a
transmitting unit that sends an infrared beam, and a receiving unit which is set
across the target area. A picture is taken when the infrared beam is broken. Passive
systems are single units that use heat and motion detectors to trigger the
camera. We used the widely available film camera systems: the TrailMaster
active (TM1550) system, and the passive systems DeerCam200 and CamTrakker;
and one passive digital system, Reconyx (Recreational).
A total of nine TrailMasters, 15 DeerCams, two CamTrakkers and two Reconyx
were dispersed among the trap sites. We began with six CamTrakkers,
but four malfunctioned and required continual troubleshooting in the field and
hence were replaced with DeerCams. At 13 locations we placed two different
camera systems on opposite sides of the trail to capture target animals from
both sides of their body. This also allowed us to compare the effectiveness of
different systems. All systems were programmed with 30-second intervals
Figure 1. Location of the study site and camera traps, indicated by numbered dots.
Camera stations were spaced systematically at 1–1.5 km apart. Buffers of 1 km surrounding
each camera station resulted in an effective survey size of 26.91 km2.
252 Northeastern Naturalist Vol. 15, No.2
between each camera trip, and cameras were active 24 hours. The Reconyx
were set to take three photographs every time the system was triggered.
Cameras operated from August 11 to October 22, 2005, for a 72-day
trapping period, which is consistent with other studies that use 60–90 days
(Karanth and Nichols 1998, Silver et al. 2004). In addition, this time of year
ensured a wide range of environmental conditions, with average monthly
temperatures ranging from -2.9 to 26.8 °C and average relative humidity at
85–90%. No snow accumulated during this time. Monthly rainfall accumulation
ranged from 22 mm to 111 mm, and average wind speed was 1.3–1.8 m/
sec. Because we could only reach half of the stations in one day of hiking, each
station was visited for maintenance at least once every 14 days. To determine
the approximate survey area, we created a 1-km buffer around each trap station
and dissolved buffers using ArcView software to calculate survey area
(Fig. 1).
We summarized the total number of photographs taken, identifiable animals
in photographs (events), and trap-nights of effort after subtracting days
where cameras malfunctioned or ran out of film or batteries. We calculated
trap success as the number of trap events per 100 trap-nights. Camera malfunctions
were usually easy to discern upon developing film and consisted of
either a camera that did not take any photos, or one that triggered randomly in
quick succession until all the film was exposed (usually within a single day).
Animals that could not be individually distinguished and were captured within
30 minutes of each other at the same station were considered to be the same
event. After 30 minutes, they were arbitrarily considered to be a new photographic
event, which is consistent with protocols of Kelly (2003) and Silver
et al. (2004). Care was taken not to double-count animals as two separate
events when two cameras on opposite sides of a trail triggered simultaneously.
The date and time stamp on each photograph, as well as animal position or
individual marks, facilitated in separating events and in eliminating double
counting. We then compared trap success among stations and camera types for
each species. We used analysis of variance (ANOVA) and Student’s t-tests to
analyze differences in trap success among camera types. Rigid head-to-head
comparisons of camera systems were not possible because camera malfunctions
were commonplace, and we had to remove cameras and replace them on
a weekly basis with other cameras that were not always the same system as the
one removed.
We created circular buffers with radii of 100, 250, 500, and 750 m surrounding
each camera trap. The smaller radii addressed habitat selection at a
scale relevant to the smaller carnivores. We did not use radii larger than 750 m
due to the autocorrelation that would occur because of the distances between
camera traps. For each buffer area, we extracted the percent cover of deciduous
forest, coniferous forest, row crops, agricultural land, and water from the
1992 National Land Cover Dataset (NLCD). We also calculated the distance
of each station to the main access road, a high-use dirt road (Rt. 613). For each
of the buffer radii, we used Student’s t-test to determine whether locations
capturing target species had a different percent cover of habitat types than
locations without target species. Due to the small sample sizes for t-tests, we
2008 M.J. Kelly and E.L. Holub 253
used an alpha value of 0.10 as our significance level. We further examined
habitat associations across camera stations using linear regression to determine
if carnivore trap success was influenced by cover type or distance to the
main access road. We calculated trap success for humans, and used linear regression
(alpha of 0.05 for significance) to determine if human use influenced
animal trap success. We also used linear regression to compare trap success of
prey animals (deer and gray squirrel) for which we had adequate data to that
of the carnivores, and to compare carnivores’ trap success to other carnivores
to determine if they avoided each other across the landscape.
Results
We recorded 363 trap events with a total of 499 animal photographs in
the 72-day trapping period (Table 1). After subtracting those days for malfunctioning
cameras, the total number of trap nights was 891 and the overall
trap success for animals captured was 40.74 per 100 trap nights (TN). Of the
363 trap events, the majority were Odocoileus virginianus (white-tailed deer;
52.34%), and Sciurus carolinensis (gray squirrel; 15.15%), followed by raccoon
(6.89%), black bear (4.68%), bobcat (3.58%), and coyote (2.48%).
Trap success varied significantly among animals (ANOVA: F = 9.439;
p < 0.001) (Fig. 2). White-tailed deer had the highest trap success (21.32/100
TN), and was photographed at all but one station (Fig. 2). Gray squirrel was
second highest (6.17/100 TN), but this was largely driven by high trap success
of it by the Reconyx system. Trap success per 100 TN for target carnivores
was: raccoon (2.81), black bear (1.91), bobcat (1.46), coyote (1.01), and gray
fox (0.56). Cameras also captured Sylvilagus floridanus (eastern cottontail),
Accipiter striatus (Sharp-shinned Hawk), Bonasa umbellus (Ruffed Grouse),
Table 1. Trap events, number of photographs of animals, and trap success for the 72-day trapping
period.
Total number Total number of
Species (common name) of trap events animal photographs
Odocoileus virginianus Zimmerman (white-tailed deer) 190 286
Sciurus carolinensis Gmelin (gray squirrel) 55 59
Procyon lotor Storr (raccoon) 25 30
Usus americanus Pallas (black bear) 17 42
Lynx rufus Schreber (bobcat) 13 14
Tamias striatus Linneaus (eastern chipmunk) 13 13
Meleagris gallopavo Linnaeus (Wild Turkey) 10 13
Canis latrans Say (coyote) 9 9
Urocyon cinereoargenteus Schreber (gray fox) 5 6
Didelphis virginiana Kerr (opossum) 4 4
Sylvilagus floridanus Allen (eastern cottontail) 1 2
Bonasa umbellus Linneaus (Ruffed Grouse) 1 1
Accipiter striatus Vieillot (Sharp-shinned Hawk) 1 1
Unknown 19 19
Grand Total 363 499
Total number of trap nights 891
Total trap success (trap events/trap nights * 100) 40.74
254 Northeastern Naturalist Vol. 15, No.2
and Meleagris gallopavo (Wild Turkey) but in low frequency (Fig. 2). No red
fox or skunk were captured, and other animals, labeled as “unknown” due to
poor quality photographs, made up a small percentage (5.2%) of events.
There was high variability in trap success among the individual trap
sites, which ranged from 0.0/100 TN (station 10) to nearly 134.7/100
TN (station 15) (Fig. 3). Trap success varied significantly among camera
systems (ANOVA: F = 8.441, p = 0.0005), ranging from 8.9/100 TN for
TrailMaster to 96.4/100 TN for Reconyx (Fig. 4). Trap success for DeerCam
was significantly higher than for TrailMaster (n = 15/100TN vs. 9/100 TN,
respectively; mean = 32.43 vs. 8.93; t = 2.07, p-value = 0.017). We did not
compare Reconyx and Camtrakker statistically because of the small sample
sizes. We note that one of the two Camtrakkers failed completely, while the
other had a 45.0/100 TN trap success.
Cameras varied in their capabilities to capture different species, with no
clear pattern of highest trap success for any one camera system across all
species (Fig. 5). However, Reconyx appears to have higher trap success for
smaller species such as raccoon, gray fox, Wild Turkey, and, in particular,
gray squirrel, which were the main driver for Reconyx’s overall higher trap
success as illustrated in Figure 3. All camera systems photographed deer in
groups of two or three, while only DeerCam photographed groups of two
bear and two bobcat; both cases were a female and her young. Only Reconyx
photographed groups of turkey, raccoon, gray fox, and gray squirrel. Reconyx’s
high sensitivity and ability to take several photographs in a row with
one triggering event most likely lead to higher trap success for medium and
small species, especially those that travel in groups. For larger or more solitary
animals such as deer, bear, or bobcat, trap-success rate was more similar
across camera systems.
Figure 2.Trap success (and standard errors) for each species averaged across the
camera stations.
2008 M.J. Kelly and E.L. Holub 255
Gray fox was recorded at only two sites, and no rigorous comparison of
habitat preference was possible. Raccoon and black bear were present at 10,
Figure 3. Trap success (number of photographic events of all animals per 100 trap
nights) for camera stations across the study site.
Figure 4. Average trap success (+ SE) among camera types. Stars indicate a signifi-
cant difference between camera types. CamTrakker and Reconyx were not analyzed
statistically due to small sample sizes.
256 Northeastern Naturalist Vol. 15, No.2
bobcat at 8, and coyote at 5 of the 16 trap sites. While there was slightly more
deciduous forest at the sites where raccoon, black bear, and coyote were present,
none of these relationships was significant for any buffer radius surrounding
traps. Bobcat, however, avoided coniferous areas, and was present in areas
with significantly less coniferous than deciduous forest for buffer sizes of 750
m (means = 8.40 vs. 19.34%, respectively; t = 2.15; p = 0.048), 500 m (means
= 10.61 vs, 21.79%, respectively; t = 2.14, p = 0.069), and in areas with marginally
significantly less coniferous for the 250-m (means = 9.10 vs. 22.10%,
respectively; t = 2.14; p = 0.153) buffer radius (Fig 6).
There were no relationships between trap success of carnivores and distance
to the main access road, except for bobcat, which showed a positive relationship
between trap success and distance from the road (Fig. 7).
Hikers/hunters were photographed at nine of the camera sites, and trap
success at sites varied widely, from 5.56/100 TN (station 12) to 436.36/100
TN (station 14) for the heavily used hiking trails. There was no relationship
between extent of human foot traffic and trap success for any of the carnivores.
There were no significant relationships between white-tailed deer trap success
and carnivore trap success. Gray squirrel occurred at eight sites, and we
found a significant (but weak) positive relationship between gray squirrel trap
success and coyote trap success (n = 16; p = 0.0391; y = 0.1849x + 0.4761; r2 =
0.2882). The only relationship between carnivore-carnivore trap success was
a marginally significant, positive relationship between black bear and bobcat
(n = 16; p = 0.0867; y = 0.4159x + 0.6814; r2 = 0.1951).
Discussion
Our study demonstrated that remote cameras can be used to survey
multiple carnivores simultaneously with a non-baited, systematic, camera
Figure 5. Trap success of species captured separated by camera type. Note that due
to small sample size for Camtrakker and Reconyx, a particular animal may not have
passed in front of those two cameras, rather than represent a true trap success of zero.
2008 M.J. Kelly and E.L. Holub 257
site set-up. We photographed 13 species: 10 mammals, five of which were
carnivores, and three birds. With the exception of the ubiquitous white-tailed
Figure 6. Bobcat presence as correlated with the percent coniferous cover among
different sized buffers. Bobcat did not occur in areas with a high percentage of coniferous
forest for buffer radii of 250 m, 500 m, and 750 m.
Figure 7. Bobcat trap success as it related to distance from the road. Across the eight
camera stations where bobcat was present, trap success increased with distance from
the main access road.
258 Northeastern Naturalist Vol. 15, No.2
deer, trap success for the other 12 species was lower than 6.5/100 TN, and
for 10 of these species, it was below 2.0/100 TN. While this trap success may
seem low, it is similar to that reported in other remote camera studies in the
US and abroad (Cuellar et al. 2006, Gompper et al. 2006, Kelly 2003, Moruzzi
et al. 2002, Séquin et al. 2003) and underscores the need to use a large
number of cameras and camera trap days of effort for carnivore studies.
Other studies have indicated that an effort of approximately 1000 trap
nights is needed to be certain that an animal is truly absent from a site (Carbone
et al. 2001). We approached this number at 891 trap nights and captured
all mid-sized to large mammals believed to be present, with the exception
of red fox, indicating either a true absence of it or a need for more effort.
Future surveys at this site should add one to two additional trapping stations
to increase trap nights above 1000 per survey, and consider changing camera
locations or trapping season.
Our study also showed that camera systems vary in their capability to capture
animals. This calls into question the use of different camera systems for a
single survey, due to the difficulties in distinguishing true variation in trap success
among animals versus among cameras. Since we paired different brands
opposite each other at each site, we believe that our study did not suffer from
camera bias. But our study also shows that different studies may require different
camera systems, depending on the species studied and the questions asked.
Therefore, our discussion below may be specific to the goals of this study—to
obtain information on multiple carnivore species across the study site and
to evaluate trap success among camera types.
DeerCam significantly outperformed TrailMaster. Additionally, we found
it more difficult to set up and to program TrailMaster’s active beam system,
leading to more user error. This system also had the most malfunctions
consisting of false triggering until all film was exposed. Also, if not set low
enough to the ground, small animals can move beneath the active infrared
beam and not be detected, as previously noted in laboratory studies (Swann et
al. 2004). TrailMaster also suffered more damage from animals, particularly
from chewing of exposed wiring. Further work is needed to statistically test
Camtrakker against DeerCam, but CamTrakker also experienced more user
error than DeerCam and was more difficult to trouble-shoot in the field. Unlike
the Swann et al. (2004) lab tests, we did not find CamTrakker to have particularly
high detection rates, and CamTrakker seemed to be the most unreliable
under field conditions, especially with high humidity and rainfall, with the
most malfunctions that consisted of no triggering. While sample sizes were
low for CamTrakker, our results are consistent with past CamTrakker experience
in other field projects (M.J. Kelly, unpubl. data; Thompson et al., in
review). Of the film cameras, DeerCam was the easiest to use and to troubleshoot,
experienced the fewest malfunctions, and was the most inexpensive.
Although sample sizes were small, the Reconyx digital performed remarkably
better than all of the film cameras. The Reconyx appeared to be more heat
and motion sensitive and to have a wider infrared beam, allowing it to record
smaller animals. While not presented in this paper, we also photographed mice
repeatedly with Reconyx. Because we programmed this system to take three
photographs with each triggering, we captured groups of raccoon, gray fox,
2008 M.J. Kelly and E.L. Holub 259
and turkey, and increased our trap success for such species. Of all the camera
systems trialed, only Reconyx had zero malfunctions in this study. This feature,
and its capability to store hundreds of photographs, make it particularly
attractive for future remote camera studies. Unlike other digital remote camera
systems (Thompson et al., in press), Reconyx has no delay between detection
of an animal and camera firing. However, image quality of Reconyx is not as
high as in the film cameras, making identification of individuals by natural
markings more difficult, especially for more subtly marked species such as
bobcat. Furthermore, the price of Reconyx (≈$500–1000 per unit) is high.
While our GIS consisted of relatively simple, clearly defined habitat
layers, our results confirm the utility of combining remote camera survey
techniques with species-specific habitat-use patterns. Other studies have
shown that coyote prefers edge sites around agricultural or disturbed open
areas, while black bear prefers interior areas away from development and
agricultural land (Moruzzi et al. 2002). We did not find this relationship for
coyote and bear, but our study site consisted almost entirely of forestland,
with only few small, open areas present. To gain more information on habitat
use, future surveys should include more habitat types.
Bobcat in our study showed preference for deciduous rather than coniferous
forest at buffer sizes of 500- to 750-m radii (0.79- to 1.77-km2 area) surrounding
each camera trap location. Habitat selection by carnivores does occur at several
spatial scales (Brown and Litvaitis 1995), but to our knowledge, this study is
the first report to show habitat selection for bobcat at such a fine spatial scale.
Other studies have focused on larger landscapes using, for example, 3.3-km
buffer radii (34-km2
area) surrounding bobcat locations (Litvaitis et al. 2006),
home-range size (8.6–60 km2) (Chamberlain et al. 2003, Lovallo and Anderson
1996), or core areas (1.4–3.0 km2) (Chamberlain et al. 2003) in comparison to
entire habitat in the surrounding study sites.
Studies in New Hampshire suggest that bobcats prefer early successional
habitats that support cottontail (Litvaitis et al. 2006), but our study site did
not contain much of this habitat for comparison. In Mississippi, bobcats, especially
males, selected mature pine stands with extensive herbaceous cover
(Chamberlain et al. 2003), while in eastern Maine, they preferred hardwood
understories, and in western Maine, softwood understories (Litvaitis et al.
1986). Bobcats also occur in dense chaparral-type vegetation in Texas (Heilbrun
et al. 2006), and clearly exhibit flexibility across the species’ range.
Bobcats avoided the main access road, indicating greater sensitivity
to vehicle disturbance than the other species. This pattern is consistent
with previous research showing that bobcats occur in areas with fewer
roads (Litvaitis et al. 2006, Lovallo and Anderson 1996), and that bobcat,
especially female, movement is influenced by disturbance from
hunters (Chamberlain et al. 1999). The main access road in our study area
was frequently driven by hunters, and this increased with the onset of the
hunting season in the middle of our survey. However, it appears that bobcats
do not respond similarly to all types of disturbance, since human foot traffic
did not influence bobcat trap success across the study site.
Heilbrun et al. (2006) had high bobcat detection rates in their similarsized
study area (31.5 km2) in Texas, and could identify individual bob260
Northeastern Naturalist Vol. 15, No.2
cats by their distinct coat patterns and estimate density through markrecapture.
Their high detection rate did correspond to high bobcat density
(48 per 100 km2). While we could identify some individual bobcats, we
did not have enough photographs with which to conduct mark-recapture
analysis and can only compare our trap success to Heilbrun et al. (2006).
It should be noted, however, detection rate (i.e., trap success) may be
related to local abundance of target species, but those indices are controversial
(Anderson 2001, 2003; Engeman 2003) because few studies have
calibrated photographic detection rates to independent assessments of
abundance. Photographic detection rates have been calibrated for Panthera
tigris L. (tiger), but even this remains controversial (Carbone et al.
2001, 2002; Jennelle et al. 2002). However, other studies have demonstrated
that photographic capture frequency correlates with abundance of
target animals (O’Brien et al. 2003).
Our trap success for bobcat (1.46/100 TN) was much lower than that
of Heilbrun et al. (2006) in the central Texas coastal plain (6.86/100 TN),
potentially indicating a much lower population density at our study size.
However, our trap success was higher than found by Moruzzi et al. (2002)
in Vermont (less than 1.0/100 TN), who did not photograph enough bobcat to
examine habitat preference. Interestingly, we had higher trap success for
bobcat than coyote, while Moruzzi et al. (2002) found the opposite. This
may indicate that bobcats have not yet been reduced in number by competition
with expanding coyote populations as suspected in Vermont (Moruzzi
et al. 2002), Maine (Litvaitis and Harrison 1989), and New Hampshire
(Litvaitis et al. 2006). We did not find evidence of avoidance of coyotes
by bobcats in this study, but if coyote range is expanding and competitively
excludes bobcats, and if abundance is correlated with detection rate
(i.e., trap success), continued surveys in this area should reveal a decrease
in bobcat trap success and an increase in coyote trap success through
time.
This study highlights the potential of remote camera monitoring over
a large area, on multiple species, and in conjunction with a GIS. The use
of remote photography can decrease survey time and effort, especially
for rare, elusive, or territorial species, and can reduce adverse effects
that may be caused by more invasive methods, such as physical capture
(Heilbrun et al. 2006). For individually identifiable specimens of species
such as bobcat (and potentially coyote; Séquin 2001), larger sample sizes
can be obtained with camera traps than in studies with physical capture,
and these remote captures can be used to obtain robust estimates of abundance
and density. For other species, photographic detection rates can be
analyzed in conjunction with their temporal pattern (e.g., by conducting
repeated surveys in the same locations over time) to calculate more accurate
estimates of relative abundance and proportion of area occupied
(MacKenzie and Royle 2005, MacKenzie et al. 2003, Royle and Nichols
2003). While we plan to increase our effort and continue to monitor on a
yearly basis, we encourage replication of this technique over different areas
and over time to build the data sets necessary for comparative analyses
and for occupancy estimates.
2008 M.J. Kelly and E.L. Holub 261
Acknowledgments
We thank the nine Virginia Tech student volunteers who gave one day of each
weekend for three months to hike to camera stations in all weather to change film
and batteries, trouble-shoot malfunctions, and/or replace cameras: Eddie Owens,
Hunter Stuart, Risa Pesapane, Jacquelyn Zidzik, Michael Biederman, Vicky Flores,
Sarah Thompson, Adam Keene, and Tirzah Breslar. Scott Klopfer provided assistance
with the geographic information system. We thank Rudolf Arndt, Richard
Heilbrun, and Andrew Noss for providing useful and insightful comments as well
as numerous editorial suggestions. Any errors or omissions that might remain in the
manuscript are solely the responsibility of the authors. We thank the Acorn Alcinda
Foundation and Virginia Tech for financial support.
Literature Cited
Anderson, D.R. 2001. The need to get the basics right in wildlife field studies.
Wildlife Society Bulletin 29:1294–1297.
Anderson, D.R. 2003. Response to Engeman: Index values rarely constitute reliable
information. Wildlife Society Bulletin 31:288–291.
Brown, A.L., and J.A. Litvaitis. 1995. Habitat features associated with predation
of New England cottontails: What scale is appropriate? Canadian Journal of
Zoology 73:1005–1011.
Carbone, C., S. Christie, K. Conforti, T. Coulson, N. Franklin, J.R. Ginsberg, M.
Griffiths, J. Holden, K. Kawanishi, M. Kinnaird, R. Laidlaw, A. Lynam, D.W.
Macdonald, D. Martyr, C. Mcdougal, L. Nath, T. O’Brien, J. Seidensticker,
D.J.L. Smith, M. Sunquist, R. Tilson, and W.N.W. Shahruddin. 2001. The use
of photographic rates to estimate densities of tigers and other cryptic mammals.
Animal Conservation 4:75–79.
Carbone, C., S. Christie, K. Conforti, T. Coulson, N. Franklin, J.R. Ginsberg, M.
Griffiths, J. Holden, M. Kinnaird, R. Laidlaw, A. Lynam, D.W. Macdonald, D.
Martyr, C. Mcdougal, L. Nath, T. O’Brien, J. Seidensticker, D.J.L. Smith, R.
Tilson, and W.N. Wan Shahruddin. 2002. The use of photographic rates to estimate
densities of tigers and other cryptic mammals: Response to Jennelle et
al. Animal Conservation 5:121–123.
Chamberlain, M.J., B.D. Leopold, L.W. Burger, B.W. Plowman, and L.M. Conner.
1999. Survival and cause-specific mortality of adult bobcats in central Mississippi.
Journal of Wildlife Management 63:613–620.
Chamberlain, M.J., B.D. Leopold, and L.M. Conner. 2003. Space use, movements
and habitat selection of adult bobcats (Lynx rufus) in central Mississippi.
American Midland Naturalist 149:395–405.
Cuellar, E., L. Maffei, R. Arispe, and A.J. Noss. 2006. Geoffroy’s cats at the
northern limit of their range: Activity patterns and density estimates from
camera trapping in Bolivian dry forests. Studies on Neotropical Fauna and
Environment 41(3): 169–177.
Cutler, T.L., and D.E. Swann. 1999. Using remote photography in wildlife ecology:
A review. Wildlife Society Bulletin 27:571–581.
Engeman, R.M. 2003. More on the need to get the basics right: Population indices.
Wildlife Society Bulletin 31:286–287.
Gompper, M.E., R.W. Kays, J.C. Ray, S.D. Lapoint, D.A. Bogan, and J.R. Cryan.
2006. A comparison of noninvasive techniques to survey carnivore communities
in northeastern North America. Wildlife Society Bulletin 34:1142–1151.
262 Northeastern Naturalist Vol. 15, No.2
Heilbrun, R.D., N.J. Silvy, M.J. Peterson, and M.E. Tewes. 2006. Estimating bobcat
abundance using automatically triggered cameras. Wildlife Society Bulletin
34:69–73.
Jennelle, C.S., M.C. Runge, and D.I. Mackenzie. 2002. The use of photographic rates to
estimate densities of tigers and other cryptic mammals: A comment on misleading
conclusions. Animal Conservation 5:119–120.
Karanth, K.U., and J.D. Nichols 1998. Estimation of tiger densities in India using photographic
captures and recaptures. Ecology 79: 2852-2862.
Kays, R.W., and K.M. Slausen. In Press. Remote cameras. In R.A. Long, P. McKay, J.C.
Ray, and W.J. Zielinski (Eds.). Noninvasive Survey Methods for North American
Carnivores. Island Press.
Kelly, M.J. 2003. Jaguar monitoring in the Chiquibul forest, Belize. Caribbean Geography
13:19–32.
Litvaitis, J.A., and D.J. Harrison. 1989. Bobcat-coyote niche relationships during a period
of coyote population increase. Canadian Journal of Zoology 67:1180–1188.
Litvaitis, J.A., J.A. Sherburne, and J.A. Bissonette. 1986. Bobcat habitat use and homerange
size in relation to prey density. Journal of Wildlife Management 50:110–117.
Litvaitis, J.A. J.P. Tash, and C.L. Stevens. 2006. The rise and fall of bobcat populations
in New Hampshire: Relevance of historical harvests to understanding current patterns
of abundance and distribution. Biological Conservation 128:517–528.
Lovallo, M.J., and E.M. Anderson. 1996. Bobcat (Lynx rufus) home-range size and habitat
use in northwest Wisconsin. American Midland Naturalist 135:241–252.
MacKenzie, D.I., and J.A. Royle. 2005. Designing occupancy studies: General advice
and allocating survey effort. Journal of Applied Ecology 42:1105–1114.
MacKenzie D.I., J.D. Nichols, J.E. Hines, M.G. Knutson, and A.B. Franklin. 2003. Estimating
site occupancy, colonization, and local extinction when a species is detected
imperfectly. Ecology 84:2200–2207.
Moruzzi, T.L., T.K. Fuller, R.M. Degraaf, R.T. Brooks, and W. Li. 2002. Assessing
remotely triggered cameras for surveying carnivore distribution. Wildlife Society
Bulletin 30:380–386.
O’Brien, T.G., M.F. Kinnaird, and H.T. Wibisono. 2003. Crouching tigers, hidden prey:
Sumatran tiger and prey populations in a tropical forest landscape. Animal Conservation
6:131–139.
Royle, J.A., and J.D. Nichols. 2003. Estimating abundance from repeated presenceabsence
data or point counts. Ecology 84:777–790.
Séquin, E.S. 2001. The influence of social status on coyote vulnerability to
photo-capture. M.Sc. Thesis. University of Nevada, Reno, NV.
Séquin, E.S., M.M. Jaeger, P.F. Brussard, and R.H. Barrett. 2003. Wariness of coyotes to
camera traps relative to social status and territory boundaries. Canadian Journal of
Zoology 81:2015–2025.
Silver, S.C., L.E.T. Ostro, L.K. Marsh, L. Maffei, A.J. Noss, M.J. Kelly, R.B. Wallace,
H. Gomez, and G. Ayala. 2004. The use of camera traps for estimating jaguar abundance
and density using capture/recapture analysis. Oryx 38:148–154.
Swann, D.E., C.C. Hass, D.C. Dalton, and S.A. Wolf. 2004. Infrared-triggered cameras
for detecting wildlife: An evaluation and review. Wildlife Society Bulletin 32:357–
365.
Thompson, S., J. Swenson, B.H. Stuart, and M.J. Kelly. Under Review. Field trials of
film and digital remote cameras. Southeastern Naturalist.