Tick Burdens on Peromyscus leucopus Rafinesque and
Infection of Ticks by Borrelia spp. in Virginia
Cheryl L. Tanner, Frank K. Ammer, Ronald E. Barry,
and Ellen Y. Stromdahl
Southeastern Naturalist, Volume 9, Issue 3 (2010): 529–546
Full-text pdf (Accessible only to subscribers.To subscribe click here.)
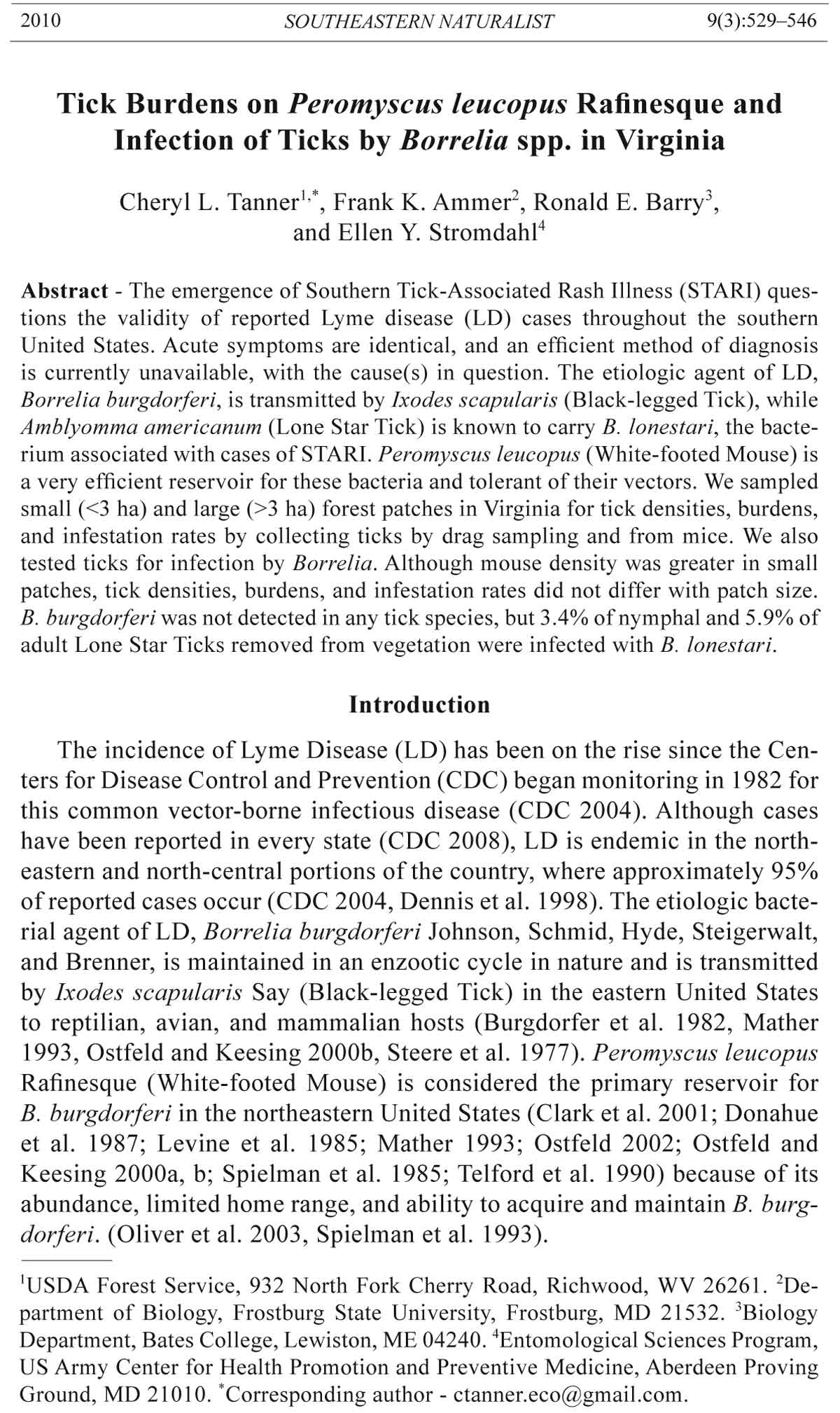
2010 SOUTHEASTERN NATURALIST 9(3):529–546
Tick Burdens on Peromyscus leucopus Rafinesque and
Infection of Ticks by Borrelia spp. in Virginia
Cheryl L. Tanner1,*, Frank K. Ammer2, Ronald E. Barry3,
and Ellen Y. Stromdahl4
Abstract - The emergence of Southern Tick-Associated Rash Illness (STARI) questions
the validity of reported Lyme disease (LD) cases throughout the southern
United States. Acute symptoms are identical, and an efficient method of diagnosis
is currently unavailable, with the cause(s) in question. The etiologic agent of LD,
Borrelia burgdorferi, is transmitted by Ixodes scapularis (Black-legged Tick), while
Amblyomma americanum (Lone Star Tick) is known to carry B. lonestari, the bacterium
associated with cases of STARI. Peromyscus leucopus (White-footed Mouse) is
a very efficient reservoir for these bacteria and tolerant of their vectors. We sampled
small (<3 ha) and large (>3 ha) forest patches in Virginia for tick densities, burdens,
and infestation rates by collecting ticks by drag sampling and from mice. We also
tested ticks for infection by Borrelia. Although mouse density was greater in small
patches, tick densities, burdens, and infestation rates did not differ with patch size.
B. burgdorferi was not detected in any tick species, but 3.4% of nymphal and 5.9% of
adult Lone Star Ticks removed from vegetation were infected with B. lonestari.
Introduction
The incidence of Lyme Disease (LD) has been on the rise since the Centers
for Disease Control and Prevention (CDC) began monitoring in 1982 for
this common vector-borne infectious disease (CDC 2004). Although cases
have been reported in every state (CDC 2008), LD is endemic in the northeastern
and north-central portions of the country, where approximately 95%
of reported cases occur (CDC 2004, Dennis et al. 1998). The etiologic bacterial
agent of LD, Borrelia burgdorferi Johnson, Schmid, Hyde, Steigerwalt,
and Brenner, is maintained in an enzootic cycle in nature and is transmitted
by Ixodes scapularis Say (Black-legged Tick) in the eastern United States
to reptilian, avian, and mammalian hosts (Burgdorfer et al. 1982, Mather
1993, Ostfeld and Keesing 2000b, Steere et al. 1977). Peromyscus leucopus
Rafinesque (White-footed Mouse) is considered the primary reservoir for
B. burgdorferi in the northeastern United States (Clark et al. 2001; Donahue
et al. 1987; Levine et al. 1985; Mather 1993; Ostfeld 2002; Ostfeld and
Keesing 2000a, b; Spielman et al. 1985; Telford et al. 1990) because of its
abundance, limited home range, and ability to acquire and maintain B. burgdorferi.
(Oliver et al. 2003, Spielman et al. 1993).
1USDA Forest Service, 932 North Fork Cherry Road, Richwood, WV 26261. 2Department
of Biology, Frostburg State University, Frostburg, MD 21532. 3Biology
Department, Bates College, Lewiston, ME 04240. 4Entomological Sciences Program,
US Army Center for Health Promotion and Preventive Medicine, Aberdeen Proving
Ground, MD 21010. *Corresponding author - ctanner.eco@gmail.com.
530 Southeastern Naturalist Vol. 9, No. 3
The Black-legged Tick, its major hosts, and B. burgdorferi thrive in deciduous
forests, but controversy persists over the effect of forest patch size
on the abundance of Black-legged Ticks and prevalence of B. burgdorferi.
Allan et al. (2002) concluded that the risk of contracting LD is higher in forest
patches of ≤2 ha compared to larger patches, but the results of Wilder and
Meikle (2004) suggested no difference to LD risk between small and large
forest patches. Patch area is only one of many factors that contribute to the
local incidence of LD. Habitat and microclimate variables as well as density
of Odocoileus virginianus Zimmerman (White-tailed Deer) contribute
greatly to the distribution of the disease-causing bacteria, their vectors, and
their hosts.
Complicating the diagnosis of the disease in the southern United States
is the emerging illness, Southern Tick-Associated Rash Illness (STARI), the
symptoms of which are indistinguishable from those of LD, except for the lack
of chronic manifestations in STARI. STARI is associated with the bite of
Amblyomma americanum L. (Lone Star Tick) and, though still under investigation,
may be caused by the bacterium B. lonestari Barbour, Maupin, Teltow,
Carter, and Piesman. The Lone Star Tick has a variety of hosts (Bishopp and
Trembley 1945, Kollars et al. 2000, Sonenshine 1979). It currently ranges
from central Texas northward to Missouri and eastward in a broad swath
across the southern United States continuing northward in a thin band up the
coast to Maine (CDC 2005, Keirans and Lacombe 1998), including areas of
sympatry with the Black-legged Tick. This overlap raises questions about the
diagnoses of LD patients in the South, given similar acute symptoms, the lack
of a reliable standard clinical test for either disease, and physician-related factors
(James et al. 2001, Kirkland et al. 1997).
Investigations into vectors for B. lonestari have almost exclusively focused
on the Lone Star Tick because this is the predominant species found
in areas of STARI cases. However, Taft et al. (2005) detected B. lonestari in
a Black-legged Tick from New York and in another from Massachusetts, but
they did not detect B. lonestari in Dermacentor variabilis Say (American
Dog Tick). Due to borreliacidal activity in its saliva, the American Dog Tick
is capable of acquiring but not maintaining B burgdorferi (Johns et al. 2000,
Piesman and Happ 1997, Piesman and Sinsky 1988). Perhaps the same borreliacidal
factor prevents the maintenance of B. lonestari. On the other hand,
because the Black-legged Tick is able to maintain B. burgdorferi (Lane et al.
1991, Piesman and Happ 1997, Piesman and Sinsky 1988), we believe it may
also maintain infection with B. lonestari, as evidenced by Taft et al. (2005).
At the time of this study, only 4 cases of LD had been reported across 3
of the 4 Virginia counties in which the study area is located (VDH 2001), and
laboratory studies have shown only 4% of Black-legged Ticks in the state
to be infected with B. burgdorferi (VDH 1997), as compared to 20–100% in
focal portions of the country. Factors that contribute to the lower prevalence
of B. burgdorferi in I. scapularis in the South include differences in feeding
behavior of the vector, host diversity, and reservoir competencies of the
2010 C.L. Tanner, F.K. Ammer, R.E. Barry, and E.Y. Stromdahl 531
hosts upon which the vector feeds (Lane et al. 1991; Oliver 1996; Ostfelt
and Keesing 2000a, b). The average infection rate of B. lonestari in Lone
Star Ticks throughout its range is approximately 2% (Armstrong et al. 2001;
Bacon et al. 2003, 2005; Barbour et al. 1996; Clark 2004; Stromdahl et al.
2003; Varela et al. 2004), and PCR analyses of Lone Star Ticks in Fort A.P.
Hill, Caroline County, VA, revealed infection rates of 4.3% (13/299) in adult
ticks and 2.8% (6/211) in nymphs (Stromdahl et al. 2003).
The main objectives of this study were to 1) examine the relationship of
tick densities to forest patch size and season; 2) examine the relationship between
forest patch size and Black-legged Tick, Lone Star Tick, and American
Dog Tick burdens on small mammals, particularly the White-footed Mouse;
3) compare infection rates of B. burgdorferi and B. lonestari between small
(<3 ha) and large (>3 ha) forest patches; and 4) investigate the presence of
B. lonestari in the Lone Star Tick, Black-legged Tick, and American Dog
Tick. We hypothesized that 1) tick burdens on White-footed Mice would be
greater in small patches because of lower mammalian diversity and higher
densities of mice in the absence of competitors and predators; 2) if Blacklegged
Tick and Lone Star Tick burdens on White-footed Mice were greater
in smaller patches, minimum infection rates (MIRs) of B. burgdorferi and
B. lonestari would be greater in those ticks; and 3) because the Black-legged
Tick and American Dog Tick have been shown to acquire B. burgdorferi,
these species also would acquire the closely related B. lonestari.
Materials and Methods
Study area
Fredericksburg and Spotsylvania County Memorial National Military
Park (FRSP) is located in both the Appalachian Piedmont and Atlantic
Coastal Plain physiographic provinces of Virginia (FRSP 2005, Linzey
1998), in Caroline, Orange, Spotsylvania, and Stafford counties. The majority
of forests are mixed deciduous with canopy species comprised of
Quercus spp. (oaks), Carya spp. (hickories), Acer spp. (maples), Liquidamber
styraciflua L. (Sweet Gum), and Liriodendron tulipifera L. (Tulip
Poplar) and understories of Vaccinium angustifolium Aiton (Late Lowbush
Blueberry), Toxicodendron radicans L. (Poison Ivy), Kalmia latifolia L.
(Mountain Laurel), Prunus spp. (cherries), and Cornus spp. (dogwoods)
(Petrides 1986, Tanner 2007).
Study sites
Forest patches were chosen according to size and habitat type using
aerial photos and subsequently ground-truthed. We attempted to locate forest
patches similar in habitat, but avoidance of the interannual impact of masting
events was not possible because oaks are abundant in FRSP (Barry et
al. 2008, NPS 2006). However, we proceeded under the assumption that the
close proximity of all sites is sufficient to assume negligible local variation.
Three small (<3-ha) and three large (>3-ha) forest patches were chosen for
532 Southeastern Naturalist Vol. 9, No. 3
replication, with a patch being defined as a forest fragment surrounded on
≥ 3 sides by nonforested habitat and, if on only 3 sides, bordered on the 4th
side by a hard edge such as a road or stream. The habitat variables of canopy
cover, ground cover, leaf-litter depth, and coarse woody debris (CWD)
volume were quantified and averaged seasonally (Tanner 2007). The microclimate
variables of ambient temperature, relative humidity (RH), soil pH,
and soil moisture were recorded daily (Tanner 2007). Pellet-group surveys
adapted from Mayle et al. (2000) and Wemmer et al. (1996) were conducted
seasonally to estimate White-tailed Deer usage at each site.
Tick collection
Ticks were collected from late April through October daily from small
mammals and monthly by drag sampling. Small-mammal trapping is considered
the most accurate sampling technique for estimating Black-legged Tick
density in areas of low prevalence like Virginia (Lane et al. 1991, Solberg
et al. 2003). All juvenile ticks were removed with forceps from each rodent
and counted to determine tick burdens. All ticks were stored in 70% ethanol
(Clark et al. 2001, Falco and Fish 1992, Ostfeld et al. 1996b) for later identification (Pratt 1961; Ward’s Natural Science n.d.) and lab determination of
Borrelia spp. infection.
Tick densities within patches were determined through drag sampling
with checks every 20 m (Daniels et al. 2000, Falco and Fish 1992, Schulze
and Jordan 2001). Ticks were counted, collected, and stored in 70% ethanol
(Clark et al. 2001, Falco and Fish 1992, Ostfeld et al. 1996b) for later identification and lab determination of Borrelia spp. infection.
Mammal sampling
Sherman live traps (7.6 x 8.9 x 22.9 cm) were arrayed in a grid configuration at each site to sample the nymphal and larval stages of ticks on
mice from late April through mid-October 2005. The number of traps used
depended on the size of the patch being sampled. Small patches contained
49 traps at 10-m intervals in 7 x 7 grids for an effective trapping area of approximately
1.12 ha. The boundary strip method (half the mean maximum
distance moved) was used to determine the effective area of the grid (Krebs
1999, Otis et al. 1978, Parmenter et al. 2003, Wilson and Anderson 1985).
Trapping effort in large patches was 100 traps at 10-m intervals in 10 x 10
grids to account for increased area, yielding an effective trapping area of
approximate 3.28 ha. In larger patches, 1 side of the grid was located along
an edge of the forest patch, and the rest of the grid extended into the interior
of the patch to incorporate both edge and interior habitats. Although small
patches inherently contain more edge than large patches because of the
increased edge-to-interior ratio, traps in small patches were arrayed in the
same configuration for consistency.
Traps were baited with a mixture of peanut butter, bird seed, and oats
and were checked once per day. To create blocking effects, 1 small and 1
large grid were sampled simultaneously for 2 or 3 consecutive days (the
2010 C.L. Tanner, F.K. Ammer, R.E. Barry, and E.Y. Stromdahl 533
schedule was adjusted from 3 days in the beginning to 2 for the remainder
of the study) approximately every 2 weeks. Captured mice were examined
for larval and nymphal ticks, which usually attach to the pinnae (Ostfeld et
al. 1993). Species, age, sex, reproductive status, and weight of each mouse
were recorded, and each individual was marked using the toe-clipping
method (Rudran 1996) for individual identification. Sampling and handling
procedures conformed to Frostburg State University IACUC requirements
and guidelines approved by the American Society of Mammalogists (Animal
Care and Use Committee 1998).
Prevalence of Borrelia spp.
Ticks were refrigerated at 4 ºC in 70% ethanol until they were processed for
isolation of their DNA. Most nymphs and larvae were pooled for extractions according
to location, species, developmental stage, and feeding status. However,
all adult ticks, Black-legged Tick nymphs, and any ticks that were lone captures
of their species and life stage from a drag session or host were processed individually.
Each individual and pool was dissected with a sterile disposable
18-gauge NoKor needle on a sterile glass surface. Extractions were performed
using both an IsoQuick Nucleic Acid Extraction Kit (ORCA Research Inc.,
Bothell, Washington) and a Qiagen DNeasy Tissue Kit (Qiagen Inc., Valencia,
CA) according to manufacturer protocols. DNA isolates were stored at 4 ºC until
amplification by polymerase chain reaction (PCR).
PCR reactions were carried out using puReTaq Ready-To-Go PCR Beads
(GE Healthcare, Piscataway, NJ) following the manufacturer’s protocol.
Each 25-μl reaction contained 1 puReTaq™ Ready-To-Go™ PCR Bead,
purified water, 1 μM of each primer, and approximately 50 ng/μl and 0.682–
1.429 ng/μl of template DNA for tick and Borrelia spp., respectively. All
reactions contained a sample of verified Borrelia spp. DNA and a sample of
purified water as positive and negative controls, respectively. PCR products
were electrophoresed on 2% agarose gels stained with ethidium bromide and
visualized with an ultraviolet (UV) transilluminator.
To verify extraction of DNA, an approximate 300-bp portion of the
mitochondrial 16S rRNA tick gene was amplified using primers 16S+2 and
16S-1 (Black and Piesman 1994), with a band at approximately 300 bp being
accepted as presence of tick DNA. Each PCR run contained a sample of
purified water as a negative control (Stromdahl et al. 2001).
After tick DNA was verified, DNA of Borrelia spp. was amplified by nested
PCR using flagellin gene primers described by Barbour et al. (1996), with
bands at approximately 240 bp from internal reactions being considered positive
for Borrelia spp. infection (Stromdahl et al. 2003). PCR samples positive
for Borrelia spp. were further analyzed by nested PCR using primers specific
for each bacterium. Samples were amplified using the p66 gene primers a, a′, f,
and f′ described by Rosa et al. (1991) specific for B. burgdorferi, with bands at
approximately 240 bp being considered positive for B. burgdorferi infection
(Rosa et al. 1991, Stromdahl et al. 2001). Samples were also amplified using
nested flagellin gene primers modified from Barbour et al. (1996) by P.C.
534 Southeastern Naturalist Vol. 9, No. 3
Williamson (University of North Texas Health Science Center, Forth Worth,
TX, unpubl. data) (Table 1) to target B. lonestari, with bands at approximately
240 bp considered positive for B. lonestari infection (Barbour et al. 1996,
Stromdahl et al. 2003).
All PCR reactions were prepared in a UV hood and run on a PTC 200
DNA Engine (MJ Research, Inc., Waltham, MA). Minimum infection rates
(MIRs) were calculated by assuming only 1 tick per positive pool as infected.
All samples positive for Borrelia spp. were purified using a Quantum Prep
Gel Slice Kit (Bio-Rad Laboratories, Hercules, CA) and sent to SeqWright
DNA Technology Services (Houston, TX) for sequencing. Sample sequences
were then compared to known sequences in the GenBank database using the
Basic Local Alignment Search Tool (BLAST) accessed through the National
Center for Biotechnology Information (NCBI; Bethesda, MD) webpage
(NCBI 2006).
Statistical analyses
Densities (D = N/A) of White-footed Mice were calculated using relative
abundance (individuals per 100 trap nights) as N and effective area (A) of the
grid (Krebs 1999, Otis et al. 1978, Parmenter et al. 2003, Wilson and Anderson
1985). Relative abundance was chosen to represent N to incorporate trapping
effort because captures in 2 of 3 large sites were consistently very low compared
to small sites due to persistent trap disturbance by Procyon lotor (L.)
(Raccoon). One-way ANOVA was used to test for significant differences in
mouse densities between patch sizes. Mean densities of ticks obtained from
drag sampling were expressed per 1000 m2 and tested by repeated measures
(rm) ANOVA for interaction and statistical significance between patch sizes
and seasons.
Because relatively few nymphs were found on mice (17 of 200 ticks removed),
larvae and nymphs were combined into the category of “juveniles”
for analysis of tick burdens. Hereafter the term “tick burden(s)” refers to the
combination of juvenile life stages on mice. Mean tick burdens were calculated
by dividing the total number of ticks found on mice by the total number
of mice captured (i.e., number of ticks per mouse). Too few mouse captures
in certain seasons at some sites prevented seasonal analysis of tick burdens.
Therefore, tick burdens were combined into site means to examine the effect
of only patch size by 1-way ANOVA. Only the 1st mouse capture per individual
per 2- or 3-day session was included in tick burden analyses to allow
reinfestation and an equal opportunity for infestation of all mice at each
Table 1. Borrelia lonestari primer sequences modified from Barbour et. al. (1996) by P.C. Williamson.
Primer Sequence
Bl-Fla 522 F 5'-GGTACATATTCAGATGCAGACAGAGGG
Bl-Fla 1182 R 5'-GCACTTGATTTGCTTGTGCAATCATAGCC
Bl-Fla 667 F 5'-CTGAAGAGCTTGGAATGCAACCTGC
Bl-Fla 907 R 5'-GAGCTAATCCCACCTTGAGCTGG
2010 C.L. Tanner, F.K. Ammer, R.E. Barry, and E.Y. Stromdahl 535
sampling event. Because sampling was repeated on the same experimental
units, rmANOVA (Zar 1999) was used to test for significant difference in
tick burdens between mouse 1) ages, 2) sexes, and 3) weights after these
variables were categorized.
Mean infestation burdens were calculated by dividing the total number
of ticks found on infested mice by the number of mice that were infested
(i.e., number of ticks per infested mouse). Infestation rates (percentages of
mice infested) were calculated by dividing the number of infested mice by
the total number of mice captured and multiplying by 100. Mean infestation
burdens and infestation rates were analyzed for treatment effects of patch
size by 1-way ANOVA.
Simple linear regression (Zar 1999) was used to test for functional dependence
of mean tick burdens on mouse densities and the relationship between
tick densities and mouse densities. The relationship between tick burdens on
mice and tick densities determined from drags could not be tested because
of species- and life stage-specific behavior resulting in insufficient data. Certain
species and life stages of ticks were found on either drags or rodents but
never both. Infection rates of B. lonestari could not be compared with tick
burdens because the bacterium was detected only in Lone Star Ticks, which
were found only on drags and not on mice.
Data in percent format (infestation rates) were arcsine square-root
transformed before analyses. All data were tested for compliance with the
assumptions of normality and homoscedasticity using Kolmogorov-Smirnov
and Levene’s tests, respectively. Most normality and almost all equal
variance assumptions were met, but ANOVA is powerful and robust, can
tolerate minor violations of assumptions (Zar 1999), and therefore was used
to analyze mouse densities, tick densities, tick burdens, infestation burdens,
and infestation rates. All samples were random, with sites chosen based on
their sizes, but samples within taken randomly. Samples that could not be
considered independent because data were recorded repeatedly from the
same 6 sites were analyzed with rmANOVA (Zar 1999). All ANOVA, regression,
normality, and homoscedasticity tests were performed using MINITAB
Release 14 statistical software (Minitab Inc., State College, Pennsylvania).
The criterion for statistical significance for all tests was P < 0.05.
Results
Mammals captured and White-footed Mouse demography
Seven species of small mammals were captured. P. leucopus comprised
74% of small mammals captured and 84% of total captures. Mean (± SE)
density of white-footed mice was significantly greater in small forest
patches (x̅small = 4.2 ± 2.0 mice/ha, x̅large = 1.0 ± 0.3 mice/ha; F = 8.01; d.f. =
1, 4; P < 0.05).
Tick density
Drags yielded no American Dog Tick larvae or nymphs or Black-legged
Tick larvae or adults (Table 2). American Dog Tick juveniles quest at the
536 Southeastern Naturalist Vol. 9, No. 3
base of vegetation and are rarely caught on drags (Sonenshine 1993), and
perhaps the timing of our sampling from late April through October did not
include the period of peak abundance of Black-legged Tick adults (early and
late fall). We found no single-factor effect of forest patch size (F = 0.00;
d.f. = 1, 12; P > 0.05), season (F = 2.53; d.f. = 2, 12; P > 0.05), or patch size
x season interaction (F = 0.02; d.f. = 2, 12; P > 0.05) on density of adult
American Dog Ticks (Table 3). No single-factor effect of patch size (F =
0.11; d.f. = 1, 12; P > 0.05), season (F = 3.53; d.f. = 2, 12; P > 0.05), or
patch size x season interaction (F = 0.07; d.f. = 2, 12; P > 0.05) on density
of nymphal Black-legged Ticks was detected. No patch size x season interaction
(F = 0.50; d.f. = 2, 12; P > 0.05), or single-factor effect by patch size
(F = 0.71; d.f. = 1, 12; P > 0.05) or season (F = 2.11; d.f. = 2, 12; P > 0.05),
on density of adult Lone Star Ticks was found. Density of nymphal Lone
Star Ticks (Table 3) did not differ significantly by patch size (F = 0.64; d.f. =
1, 12; P > 0.05), season (F = 2.10; d.f. = 2, 12; P > 0.05), or interaction of
patch size x season (F = 0.36; d.f. = 2, 12; P > 0.05). Because of a significant
patch size x season interaction (F = 4.65; d.f. = 2, 12; P < 0.05), main effects
of patch size and season on density of larval Lone Star Ticks could not be
analyzed. However, regression analysis revealed no functional dependence
of density of Lone Star Tick larvae on density of mice (F = 3.29; d.f. = 1, 4;
P > 0.05). Similarly, there was no functional dependence of density of Lone
Star Tick nymphs (F = 0.24; d.f. = 1, 4; P > 0.05), Lone Star Tick adults (F =
0.32; d.f. = 1, 4; P > 0.05), Black-legged Tick nymphs (F = 0.04; d.f. = 1, 4;
P > 0.05), or American Dog Tick adults (F = 0.87; d.f. = 1, 4; P > 0.05) on
density of mice. We found no single-factor effect of forest patch size (F =
0.18; d.f. = 1, 12; P > 0.05), season (F = 0.66; d.f. = 2, 12; P > 0.05), or
Table 2. Sources and total numbers of ticks tested for, and minimum infection rates (MIRs) of
ticks by, Borrelia spp.
B. burgdorferi B. lonestari
Source of ticks % of Sample MIR Sample MIR
Tick species/life stage Drags Rodents* Total sample size (%) size (%)
Amblyomma americanum
Larvae 72 0 72 13.1 72 0 72 0.0
Nymphs 237 0 237 43.2 237 0 237 3.4
Adults 17 0 7 3.1 17 0 17 5.9
Dermacentor variabilis
Larvae 0 129 129 23.5 129 0 129 0.0
Nymphs 0 15 15 2.7 15 0 15 0.0
Adults 7 0 7 1.3 7 0 7 0.0
Ixodes scapularis
Larvae 0 49 49 8.9 49 0 49 0.0
Nymphs 20 2 22 4.0 22 0 22 0.0
Adults 0 0 0 0.0 0 0 0 0.0
Totals 353 195 548 100
*All rodents are Peromyscus leucopus except for 1 Mus musculus from which 2 D. variabilis
larvae were removed.
2010 C.L. Tanner, F.K. Ammer, R.E. Barry, and E.Y. Stromdahl 537
patch size x season interaction (F = 0.02; d.f. = 2, 12; P > 0.05) on density
of White-tailed Deer as reflected by usage indices.
Tick burdens
All but 2 ticks (of any species) were encountered on White-footed Mice;
2 larval American Dog Ticks were found on a single Mus musculus L. (House
Mouse). No Lone Star Ticks of any life stage or adult ticks of any species
were documented on mice (Table 2). Black-legged Tick (F = 3.27; d.f. = 1, 4;
P > 0.05) and American Dog Tick (F = 1.20; d.f. = 1, 4; P > 0.05) burdens
on newly captured mice did not differ between forest patch sizes (Table 4).
Similarly, when recaptures were included in the model, Black-legged Tick
(F = 1.23; d.f. = 1, 4; P > 0.05) and American Dog Tick (F = 1.57; d.f. = 1, 4;
P > 0.05) burdens did not differ by patch size (Table 4). Mean tick burdens of
infested mice (Table 4) did not differ with patch size for either the American
Dog Tick (F = 0.00; d.f. = 1, 4; P > 0.05) or Black-legged Tick (F = 0.41;
d.f. = 1, 4; P > 0.05).
Infestation rates by the American Dog Tick (F = 2.78; d.f. = 1, 4; P > 0.05)
and Black-legged Tick (F = 2.70; d.f. = 1, 4; P > 0.05) did not differ with patch
Table 3. Mean (± SE) overall and seasonal tick densities (per 1000 m2) in small (<3 ha; S) and
large (>3 ha; L) deciduous forest patches.
Season
Species Patch size Overall Spring Summer Fall
Dermacentor variabilis adults S 1.1 (1.1) 3.2 (3.2) 0.0 (0.0) 0.0 (0.0)
L 1.1 (0.7) 3.0 (2.0) 0.4 (0.4) 0.0 (0.0)
Ixodes scapularis nymphs S 2.1 (2.1) 6.4 (6.4) 0.0 (0.0) 0.0 (0.0)
L 2.8 (1.1) 6.7 (1.3) 1.9 (0.4) 0.0 (0.0)
Amblyomma americanum adults S 1.3 (1.1) 3.2 (3.2) 0.8 (0.8) 0.0 (0.0)
L 3.5 (2.4) 8.9 (6.8) 1.1 (0.6) 0.4 (0.4)
A. americanum nymphs S 18.3 (10.8) 23.8 (19.2) 31.0 (27.5) 0.0 (0.0)
L 32.0 (14.0) 57.8 (33.2) 37.0 (20.5) 1.1 (0.6)
Table 4. Mean (± SE) overall tick burdens and infestation rates of newly captured mice by 2
species of ticks in small (<3 ha; S) and large (>3 ha; L) deciduous forest patches.
Patch size
Variable S L
Burdens on mice (ticks/mouse)*
Dermacentor variabilis 1.2 (0.4) 0.6 (0.3)
Ixodes scapularis 0.1 (0.1) 0.3 (0.2)
Burdens on infested mice (ticks/mice)*
D. variabilis 2.5 (0.4) 2.5 (0.4)
I. scapularis 1.6 (0.3) 2.3 (0.4)
Infestation rates (%)*
D. variabilis 41.9 15.1
I. scapularis 5.8 17.4
*Includes recaptures (see text).
538 Southeastern Naturalist Vol. 9, No. 3
size (Table 4). Neither Black-legged Tick (F = 2.40; d.f. = 1, 8; P > 0.05) nor
American Dog Tick (F = 0.55; d.f. = 1, 8; P > 0.05) burdens differed signifi-
cantly by mouse age. No significant difference by mouse sex was detected in
Black-legged Tick (F = 0.01; d.f. = 1, 8; P > 0.05) or American Dog Tick (F =
0.29; d.f. = 1, 8; P > 0.05) burdens. Black-legged Tick (F = 0.84; d.f. = 2, 12;
P > 0.05) and American Dog Tick (F = 0.61; d.f. = 2, 12; P > 0.05) burdens did
not differ significantly by mouse weight. Neither juvenile Black-legged Tick
(F = 1.66; d.f. = 1, 4; P > 0.05) nor American Dog Tick (F = 0.00; d.f. = 1, 4;
P > 0.05) burdens were functionally dependent on mouse density.
Borrelia spp. minimum infection rates
All 188 tick individuals and pools yielded tick DNA, with further analysis
confirming 9 samples positive for B. lonestari infection. No Lone Star
Tick larvae, Black-legged Ticks, or American Dog Ticks were infected
(Table 2). All ticks species were negative for B. burgdorferi infection; i.e.,
an MIR of 0% regardless of life stage. All positive samples for B. lonestari
originated from Lone Star Ticks, with an MIR of 5.9% (1 of 17) for adults
and 3.4% (8 of 237) for nymphs. MIRs could not be analyzed between forest
patch sizes because of inadequate tick sample sizes within each site.
All Borrelia-positive nucleotide sequences aligned with known B. lonestari
sequences in the Genbank database for further confirmation of sample
results. Most but not all samples contained some unidentified nucleotides
on either side of the target loci. However, all target sequences aligned 100%
with known sequences. Sample sequence lengths ranging from 177–206 nucleotides
aligned perfectly (100% with no mismatches or gaps) with several
known sequences originated from 13 states (including Virginia) throughout
the range of the Lone Star Tick.
Discussion
Competitive and predatory pressures on White-footed Mice should be
reduced in smaller forest patches, allowing one of the most competent reservoirs
for B. burgdorferi to flourish (Nupp and Swihart 1996, 1998). While
our research supports this assertion with greater mouse density in small forest
patches, tick densities in small and large patches did not differ, and the
percentage of mice infested with ticks also did not differ with patch size,
perhaps due to sample size. Densities of Lone Star Tick larvae, nymphs, or
adults, American Dog Tick adults, or Black-legged Tick nymphs removed
from vegetation were not dependent on mouse density. We expected greater
tick burdens on mice where there was a higher mouse density, but Blacklegged
Tick and American Dog Tick burdens on mice showed no dependence
on mouse density. Neither Black-legged Ticks nor American Dog Ticks demonstrated
selection for mouse age, sex, or weight in our investigation (Tanner
2007). Collectively, these observations suggest that, in some locations, factors
other than the density of competent reservoirs for B. burgdorferi may be
as important as patch size in LD ecology.
2010 C.L. Tanner, F.K. Ammer, R.E. Barry, and E.Y. Stromdahl 539
Mast production is a major determinant of deer activity and abundance
of White-footed Mice (McShea and Schwede 1993; Ostfeld 1997, 2002;
Ostfeld et al. 1996a), but many other habitat and microclimate variables
share in determining tick survival, densities, and burdens once eggs are
laid (Lane et al. 1991, Ostfeld 1997, Ostfeld et al. 1996a). Leaf-litter depth,
canopy cover, ambient temperature, relative humidity, soil moisture, and
soil pH differed seasonally in our investigation (Tanner 2007) and may have
determined tick activity and survival, but such variation between late April
and October when sampling occurred had no apparent role in determination
of tick densities in our study. Canopy cover, ground cover, and soil moisture
differed between small and large patches and may have affected mouse density
(Tanner 2007). However, differences in these habitat variables did not
effect differences in tick densities in patches differing in size. Variables that
affect deer movement, such as masting activity (Koenig and Knops 2002,
McShea and Rappole 1992, McShea and Schwede 1993), have a greater effect
on tick densities because their eggs will be laid where they detach from
the deer. However, deer usage showed no difference with patch size in our
study, which was expected with a lack of difference in tick densities and
burdens. That tick densities and burdens were low compared to other studies
(Allan et al. 2002, Armstrong et al. 2001, Clark et al. 2001, Kollars et
al. 2000), regardless of patch size, suggests that conditions in FRSP are not
optimal for tick promulgation and/or survival. Seasonal occurrence of the 3
tick species at this Virginia site (Table 2) was similar to that reported from
other Mid-Atlantic sites (Schulze et al. 1986).
Stromdahl et al. (2008) detected a low prevalence (4/1320 = 0.3% MIR)
of B. lonestari in Lone Star Tick larvae. Failure to detect B. lonestari in larvae
in this study was probably due to a comparatively small sample size and the
low expectation of transovarial transmission. The MIRs of Lone Star Ticks by
B. lonestari in this study (5.9% adult and 3.4% nymphal) are within the range
(1–5%) reported by numerous researchers throughout the South (Armstrong
et al. 2001; Bacon et al. 2003, 3005; Barbour et al. 1996; Clark 2004; Varela et
al. 2004) and in Caroline County, VA (4.3% adult and 2.8% nymphal; Stromdahl
et al. 2003), further supporting its distribution and incidence. B. lonestari
nucleotide sequences from Lone Star Ticks matched 100% with those reported
from 11 southern states—Arkansas, Florida, Georgia, Kansas, Kentucky,
Maryland, Missouri, North Carolina, South Carolina, Tennessee, and Virginia—
and New Jersey and New York. These findings do not support our hypothesis
of higher bacterial prevalence accompanying higher mouse densities in
smaller patches, but sample sizes were relatively small.
Infection rates of ticks by B. lonestari may be relatively low in the South
(Armstrong et al. 2001; Bacon et al. 2003, 2005; Barbour et al. 1996; Clark
2004; Varela et al. 2004), but infection rates by B. burgdorferi appear even
lower. Approximately 95% of LD cases occur in the northeastern and northcentral
portions of the US, but cases are scant and scattered in the South,
where the vector is also established (CDC 2004, Dennis et al. 1998). In
540 Southeastern Naturalist Vol. 9, No. 3
the South, the Lone Star Tick is more abundant than the Black-legged Tick
(Armstrong et al. 2001, Bishopp and Trembley 1945, Childs and Paddock
2003, James et al. 2001, Varela 2004). This finding is consistent with the
results of our tick sampling in which Lone Star Ticks comprised almost 60%
of all ticks collected (drags and burdens), and Black-legged Ticks only accounted
for approximately 13%. Our sample size for Lone Star Ticks was
much greater than that for Black-legged Ticks, which could have led to
greater detection ability of B. lonestari compared to B. burgdorferi.
The American Dog Tick was negative for both B. lonestari and
B. burgdorferi, suggesting that the species is a poor vector for the former,
in contrast to what we hypothesized. However, sample size was relatively
low, with larvae represented disproportionately. Infection with B. burgdorferi
activates an antimicrobial defense in American Dog Ticks that rapidly
kills the bacterium (Johns et al. 2000), so that even if the tick acquires the
pathogen it does not maintain it. Perhaps the same is true of its infection
with B. lonestari. That American Dog Ticks in this study did not acquire
B. lonestari does not mean that the ability is absent. Perhaps its efficiency of
acquiring B. lonestari is similar to that of acquiring B. burgdorferi. Mukolwe
et al. (1992), Piesman and Happ (1997), and Piesman and Sinsky (1988)
have documented low acquisition levels of B. burgdorferi in American Dog
Ticks, whereas we and Taft et al. (2005) documented none. Our sample sizes
may have been too small to detect Borrelia spp. in American Dog Ticks.
Contrary to our hypothesis, we found no Black-legged Ticks infected
with B. lonestari. However, Taft et al. (2005) documented 2 Black-legged
Ticks infected with B. lonestari in the northeastern portion of the country.
Few tests have been conducted to determine other possible vectors of
B. lonestari other than its principal vector, the Lone Star Tick. Coinfections
with pathogens in different genera also have been documented for the Blacklegged
Tick (Schauber et al. 1998, Stromdahl et al. 2008), so it is possible
that this species can transmit both B. burgdorferi and B. lonestari. Further
testing with larger sample sizes is necessary to determine other possible vectors
for B. lonestari, and the investigation into the bacterium’s role in STARI
is ongoing.
The few potential hosts captured (Tanner 2007) that were not Whitefooted
Mice may serve as “dilution” or “rescue” species during times of low
densities of the principal host (Ostfeld and Keesing 2000a, b). Incidental
small-mammal captures included the House Mouse, Blarina brevicauda Say
(Northern Short-tailed Shrew), Tamias striatus L. (Eastern Chipmunk), Didelphis
virginianus Kerr (Virginia Opossum), Sciurus carolinensis Gmelin (Gray
Squirrel), and Rattus norvegicus Berkenhout (Norway Rat), all of which have
demonstrated some level of reservoir competence (Craine et al. 1997, Gage et
al. 1995, Gern et al. 1998, Godsey et al. 1987, Markowski et al. 1998, Mather
1993, Matuschka et al. 1997, McLean et al. 1993, Ostfeld and Keesing 2000b,
Slajchert et al. 1997, Telford et al. 1990). Lizards seen in the study sites were
Eumeces fasciatus L. (Five-lined Skink), E. laticeps Schneider (Broadhead
2010 C.L. Tanner, F.K. Ammer, R.E. Barry, and E.Y. Stromdahl 541
Skink), and Sceloporus undulatus Green (Northern Fence Lizard). Any
of them may serve as “rescue” or “dilution” species (Ostfeld and Keesing
2000b), but the reservoir competencies of these species are unknown.
Based on our research, we do not recommend active management for
LD or STARI at FRSP. Because of the time, cost, and effort required,
management procedures are feasible only in areas with high risk factors,
including high tick burdens and densities, and high prevalence of the tickborne
pathogen. The relatively low density of Black-legged Ticks within the
Park, the low burdens of the species on reservoir mice, and the absence of
B. burgdorferi in all tick species collected suggest only a remote probability
of contracting LD in FRSP. Even though no Lone Star Ticks were found on
reservoir mice, the density of this species was the greatest of the 3 studied,
and B. lonestari was detected. American Dog Ticks yielded the lowest density
but highest burdens, yet no bacteria. These tick densities and burdens,
together with low Borrelia spp. prevalence, suggest a low risk of contracting
STARI, if B. lonestari is shown to be the causative agent, and an even lower
probability of contracting LD in the Fredericksburg region.
Acknowledgments
We thank Drs. Durland Shumway and Lance Revennaugh for statistical expertise
and Drs. Wayne Yoder and Rich Robbins (the latter at the Defense Pest Management
Information Analysis Center/Armed Forces Pest Management Board of the Walter Reed
Army Medical Center, Washington, DC) for assistance with initial tick identification.
Field housing, equipment, and funding were provided by the National Park Service
(NPS) under Cooperative Agreement 1443DCA309701200 with Ronald Barry.
NPS Natural Resource Manager Gregg Kneipp provided GIS maps and frequent
general support. We thank Dr. William Seddon and the Frostburg State University
Department of Biology for providing laboratory supplies. We also thank Mrs. Valerie
Fritz for obtaining both field and laboratory supplies and Ms. Bebe Elrick for her
continuous help and support. C.L. Tanner thanks her family for their unyielding support,
encouragement, and confidence.
Literature Cited
Allan, B.F., F. Keesing, and R.S. Ostfeld. 2002. Effect of forest fragmentation on
Lyme disease risk. Conservation Biology 17:267–272.
Animal Care and Use Committee. 1998. Guidelines for the capture, handling, and
care of mammals as approved by the American Society of Mammalogists. Journal
of Mammalogy 79:1416–1431.
Armstrong, P.M., L.R. Brunet, A. Spielman, and S.R. Telford, III. 2001. Risk of
Lyme disease: Perceptions of residents of a Lone Star Tick-infested community.
Bulletin of the World Health Organization 79:916–925.
Bacon, R.M., R.D. Gilmore, Jr., M. Quintana, J. Piesman, and B.J.B. Johnson. 2003.
DNA evidence of Borrelia lonestari in Amblyomma americanum (Acari: Ixodidae)
in southeast Missouri. Journal of Medical Entomology 40:590–592.
Bacon, R.M., M.A. Pilgard, B.J.B Johnson, J. Piesman, B.J. Biggerstaff, and M.
Quintana. 2005. Rapid detection methods and prevalence estimation for Borrelia
lonestari glpQ in Amblyomma americanum (Acari: Ixodidae) pools of unequal
size. Vector-Borne and Zoonotic Diseases 5:146–156.
542 Southeastern Naturalist Vol. 9, No. 3
Barbour, A.G., G.O. Maupin, G.J. Teltoe, C.J. Carter, and J. Piesman. 1996. Identifi-
cation of an uncultivable Borrelia species in the hard tick Amblyomma americanum:
Possible agent of a Lyme disease-like illness. Journal of Infectious Diseases
173:403–409.
Barry, R.E., C.L. Tanner, and J.M. Mulligan. 2008. Inventory of mammals (excluding
bats) of Fredericksburg and Spotsylvania National Military Park. Technical
Report NPS/NCR/NRTR-2008/121. National Park Service, Philadelphia, PA.
Bishopp, F.C., and H.L. Trembley. 1945. Distribution and hosts of certain North
American ticks. Journal of Parasitology 31:1–51.
Black, W.C., and J. Piesman. 1994. Phylogeny of hard- and soft-tick taxa (Acari: Ixodida)
based on mitochondrial 16S rDNA sequences. Proceedings of the National
Academy of Sciences 91:10034–10038.
Burgdorfer, W., A.G. Barbour, S.F. Hayes, J.L. Benach, E. Grunwaldt, and J.P. Davis.
1982. Lyme disease: A tick-borne spirochetosis? Science 216:1317–1319.
Centers for Disease Control and Prevention (CDC). 2004. Morbidity and Mortality
Weekly Report 53(17):365–369. Atlanta, GA.
CDC. 2005. Southern tick-asociated rash illness. Available online at http://www.cdc.
gov/ncidod/dvbid/stari/index.htm. Accessed August 2006.
CDC. 2008. Reported Lyme Disease Cases by State, 1993–2007. http://www.cdc.
gov/ncidod/dvbid/lyme/ld_rptdLymeCasesbyState.htm. Available online at Accessed
November 2008.
Childs, J.E., and C.D. Paddock. 2003. The ascendancy of Amblyomma americanum
as a vector of pathogens affecting humans in the United States. Annual Review
of Entomology 48:307–337.
Clark, K. 2004. Borrelia species in host-seeking ticks and small mammals in northern
Florida. Journal of Clinical Microbiology 42:5076–5086.
Clark, K.L., J.H. Oliver, Jr., J.M. Grego, A.M. James, L.A. Durden, and C.W. Banks.
2001. Host associations of ticks parasitizing rodents at Borrelia burgdorferi enzootic
sites in South Carolina. Journal of Parasitology 87:1379–1386.
Craine, N.G., P.A. Nuttall, A.C. Marriott, and S.E. Randolph. 1997. Role of Grey
Squirrels and pheasants in the transmission of Borrelia burgdorferi sensu lato,
the Lyme disease spirochete, in the UK. Folia Parasitologica 44:155–160.
Daniels, T.J., R.C. Falco, and D. Fish. 2000. Estimating population size and drag
sampling efficiency for the Black-legged Tick (Acari: Ixodidae). Journal of
Medical Entomology 37:357–363.
Dennis, D.T., T.S. Nekomoto, J.C. Victor, W.S. Paul, and J. Piesman. 1998. Reported
distribution of Ixodes scapularis and Ixodes pacificus (Acari: Ixodidae) in the
United States. Journal of Medical Entomology 35:629–638.
Donahue, J.G., J. Piesman, and A. Spielman. 1987. Reservoir competence of Whitefooted
Mice for Lyme disease spirochetes. American Journal of Tropical Medicine
and Hygiene 36:92–96.
Falco, R.C., and D. Fish. 1992. A comparison of methods for sampling the Deer Tick,
Ixodes dammini, in a Lyme disease endemic area. Experimental and Applied
Acarology 14:165–173.
Fredericksburg and Spotsylvania County Memorial National Military Park (FRSP).
2005. Fredericksburg and Spotsylvania County Memorial National Military Park.
Available online at http://www.nps.gov/frsp/pphtml/nature.html. Accessed June
2005.
Gage, K.L., R.S. Ostfeld, and J.G. Olson. 1995. Nonviral vector-borne zoonoses associated
with mammals in the United States. Journal of Mammalogy 76:695–715.
2010 C.L. Tanner, F.K. Ammer, R.E. Barry, and E.Y. Stromdahl 543
Gern, L., A. Estrada-Peña, F. Frandsen, J.S. Gra, T.G. Jaenson, F. Jongejan, O. Kahl,
E. Korenberg, R. Hehl, and P.A. Nuttall. 1998. European reservoir hosts of Borrelia
burgdorferi sensu lato. Zentralbl Bakteriol 287:196–204.
Godsey, M.S., Jr., T.E. Amundson, E.C. Burgess, W. Schell, J.P. Davis, R. Kaslow,
and R. Edelman. 1987. Lyme disease ecology in Wisconsin: Distribution and host
preferences of Ixodes dammini, and prevalence of antibody to Borrelia burgdorferi
in small mammals. American Journal of Tropical Medicine and Hygiene
37:180–187.
James, A.M., D. Liveris, G.P. Wormser, I. Schwartz, M.A. Montecalvo, and B.J.B.
Johnson. 2001. Borrelia lonestari infection after a bite by an Amblyomma americanum
tick. Journal of Infectious Diseases 183:1810–1814.
Johns, R., D.E. Sonenshine, and W.L. Hynes. 2000. Response of the tick Dermacentor
variabilis (Acari: Ixodidae) to hemocoelic inoculation of Borrelia burgdorferi
(Spirochetales). Journal of Medical Entomology 37:265–270.
Keirans, J.E., and E.H. Lacombe. 1998. First records of Amblyomma americanum,
Ixodes (Ixodes) dentatus, and Ixodes (Ceratixodes) uriae (Acari: Ixodidae) from
Maine. Journal of Parasitology 84:629–631.
Kirkland, K.B., T.B. klimko, R.A. Meriwether, M. Schriefer, M. Levin, J. Levine,
W.R. MacKennzie, and D.T. Dennis. 1997. Erythema migrans-like rash illness at
camp in North Carolina: A new tick-borne disease? Archives of Internal Medicine
157(22):2635–2641.
Koenig, W.D., and J.M.H. Knops. 2002. The behavioral ecology of masting in oaks.
Pp. 129–148, In W.J. McShea and W.M. Healy (Eds.). Oak Forest Ecosystems:
Ecology and Management for Wildlife. Johns Hopkins University Press, Baltimore,
MD.
Kollars, T.M., Jr., J.H. Oliver, Jr., L.A. Durden, and P.G. Kollars. 2000. Host associations
and seasonal activity of Amblyomma americanum (Acari: Ixodidae) in
Missouri. Journal of Parasitology 86:1156–1159.
Krebs, C.J. 1999. Ecological Methodology. 2nd Edition. Addison-Welsey Educational
Publisher, Inc., Menlo Park, CA.
Lane, R.S., J. Piesman, and W. Burgdorfer. 1991. Lyme borreliosis: Relation of its
causative agent to its vectors and hosts in North America and Europe. Annual
Review of Entomology 36:587–609.
Levine, J.F., W.L. Wilson, and A. Spielman. 1985. Mice as reservoirs of the Lyme disease
spirochete. American Journal of Tropical Medicine and Hygiene 34:355–360.
Linzey, D.W. 1998. The Mammals of Virginia. The McDonald and Woodward Publishing
Company, Blacksburg, VA.
Markowski, D., H.S. Ginsberg, K.E. Hyland, and R. Hu. 1998. Reservoir competence
of the Meadow Vole (Rodentia: Cricetidae) for the Lyme disease spirochete Borrelia
burgdorferi. Journal of Medical Entomology 35:804–808.
Mather, T.N. 1993. The dynamics of spirochete transmission between ticks and vertebrates.
Pp. 43–62, In H.S. Ginsberg (Ed.). Ecology and Environmental Management
of Lyme Disease. Rutgers University Press, New Brunswick, NJ.
Matuschku, F.R., S. Endepols, D. Richter, and A. Spielman. 1997. Competence of
urban rats as reservoir hosts for Lyme disease spirochetes. Journal of Medical
Entomology 34:489–493.
Mayle, B.A., R.J. Putman, and I. Wyllie. 2000. The use of trackway counts to establish
an index of deer presence. Mammal Review 30:233–237.
McLean, R.G., S.R. Ubico, and L.M. Cooksey. 1993. Experimental inoculation of the
Eastern Chipmunk (Tamias striatus) with the Lyme disease spirochete (Borrelia
burgdorferi). Journal of Wildlife Diseases 29:527–532.
544 Southeastern Naturalist Vol. 9, No. 3
McShea, W.J., and J.H. Rappole. 1992. White-tailed Deer as a keystone species
within forest habitats of Virginia. Virginia Journal of Science 43:177–186.
McShea, W.J., and G. Schwede. 1993. Variable acorn crops: Responses of Whitetailed
Deer and other mast consumers. Journal of Mammalogy 74:999–1006.
Mukolwe, S.W., A.A. Kocan, R.W. Berker, K.M. Kokan, and G.L. Murphy. 1992. Attempted
transmission of Borrelia burgdorferi (Spirochaetales: Spirochaetaceae)
(JDI strain) by Ixodes scapularis (Acari: Ixodidae), Dermacentor variabilis, and
Amblyomma americanum. Journal of Medical Entomology 29:673–677.
National Center for Biotechnology Information (NCBI). 2006. US National Library
of Medicine. Bethesdsa, MD. Available online at http://www.ncbi.nih.gov/. Accessed
August 2006.
National Park Service (NPS). 2006. NPSpecies. Available online at http://www.science1.
nature.nps.gov/npspecies/. Accessed May 2006.
Nupp, T.E., and R.K. Swihart. 1996. Effect of forest patch area on population attributes
of White-footed Mice (Peromyscus leucopus) in fragmented landscapes.
Canadian Journal of Zoology 74:467–472.
Nupp, T.E., and R.K. Swihart. 1998. Effects of forest fragmentation on population
attributes of White-footed Mice and Eastern Chipmunks. Journal of Mammalogy
79:1234–1243.
Oliver, J.H., Jr. 1996. Lyme borreliosis in the southern United States: A review. Journal
of Parasitology 82:926–935.
Oliver, J.H., Jr., T. Lin, L. Gao, K.L. Clark, C.W. Banks, L.A. Durden, A.M. James,
and F.W. Chandler, Jr. 2003. An enzootic transmission cycle of Lyme borreliosis
spirochetes in the southeastern United States. Proceedings of the National Academy
of Sciences 100:11642–11645.
Ostfeld, R.S. 1997. The ecology of Lyme disease risk. American Scientist 85:338–346.
Ostfeld, R.S. 2002. Ecological webs involving acorns and mice: Basic research and
its management implications. Pp. 196–214, In W.J. McShea and W.M. Healy
(Eds.). Oak Forest Ecosystems: Ecology and Management for Wildlife. Johns
Hopkins University Press, Baltimore, MD.
Ostfeld, R.S., and F. Keesing. 2000a. Biodiversity and disease risk: The case of Lyme
disease. Conservation Biology 14:722–728.
Ostfeld, R.S., and F. Keesing. 2000b. The function of biodiversity in the ecology of
vector–borne zoonotic diseases. Canadian Journal of Zoology 78:2061–2078.
Ostfeld, R.S., M.C. Miller, and J. Schnurr. 1993. Ear tagging increases tick (Ixodes
dammini) infestation rates of White-footed Mice (Peromyscus leucopus). Journal
of Mammalogy 74:651–655.
Ostfeld, R.S., C.G. Jones, and J.O. Wolff. 1996a. Of mice and mast: Ecological connections
in eastern deciduous forests. Bioscience 46:323–330.
Otis, D.L., K.P. Burnham, G.C. White, and D.R. Anderson. 1978. Statistical inference
from capture data on closed animals populations. Wildlife Monographs
62:1–135.
Parmenter, R.R., T.L. Yates, D.R. Anderson, K.P. Burnham, J.L. Dunnum, A.B.
Franklin, M.T. Friggens, B.C. Lubow, M. Miller, G.S. Olson, C.A. Parmenter,
J. Pollard, E. Rexstad, T.M. Shenk, T.R. Stanley, and G.C. White. 2003. Smallmammal
density estimation: A field comparison of grid-based vs. web-based
density estimators. Ecological Monographs 73:1–26.
Petrides, G.A. 1986. A Field Guide to Trees and Shrubs: Northeastern and Northcentral
United States and Southeastern and South-central Canada. 2nd Edition.
Houghton Mifflin Company, New York, NY.
2010 C.L. Tanner, F.K. Ammer, R.E. Barry, and E.Y. Stromdahl 545
Piesman, J., and C.M. Happ. 1997. Ability of the Lyme disease spirochete Borrelia
burgdorferi to infect rodents and three species of human-biting ticks (Blacklegged
Tick, American Dog Tick, Lone Star Tick) (Acari: Ixodidae). Journal of
Medical Entomology 34:451–456.
Piesman, J., and R.J. Sinsky. 1988. Ability of Ixodes scapularis, Dermacentor variabilis,
and Amblyomma americanum (Acari: Ixodidae) to acquire, maintain, and
transmit Lyme disease spirochetes (Borrelia burgdorferi). Journal of Medical
Entomology 25:336–339.
Pratt, H.D. 1961. Ticks: Key to genera in United States. US Department of Health,
Education, and Welfare, Public Health Service, Communicable Disease Center,
Training Branch, Atlanta, GA.
Rosa, P.A., D. Hogan, and T.G. Schwan. 1991. Polymerase chain reaction analyses
identify two classes of Borrelia burgdorferi. Clinical Microbiology 29:524–532.
Rudran, R. 1996. Methods for marking mammals: General marking techniques. Pp.
299–304, In D.E. Wilson, F.R. Cole, J.D. Nichols, R. Rudran, and M.S. Foster.
(Eds.). Measuring and Monitoring Biological Diversity: Standard Methods for
Mammals. Smithsonian Institution Press, Washington, DC.
Schauber, E.M., S.J. Gertz, W.T. Maple, and R.S. Ostfeld. 1998. Coinfection of
Blacklegged Ticks (Acari: Ixodidae) in Dutchess County, New York, with the
agents of Lyme disease and human granulocytic ehrlichiosis. Journal of Medical
Entomology 35:901–903.
Schulze, T.L., and R.A. Jordan. 2001. Effects of habitat structure on the retention of
Ixodes scapularis and Amblyomma americanum (Acari: Ixodidae) adults during
drag sampling surveys. Journal of Medical Entomology 38:606–608.
Schulze, T.L., G.S. Bowen, M.F. Lakat, W.E. Parkin, and J.K. Shisler. 1986. Seasonal
abundance and hosts of Ixodes dammini (Acari: Ixodidae) and other ixodid ticks
from an endemic Lyme disease focus in New Jersey, USA. Journal of Medical
Entomology 23:105–109.
Slajchert, T., U.D. Kitron, C.J. Jones, and A. Mannelli. 1997. Role of the Eastern
Chipmunk (Tamias striatus) in the epizootiology of Lyme borreliosis in northwestern
Illinois, USA. Journal of Wildlife Diseases 33:40–46.
Solberg, V.B., J.A. Moller, T. Hadfield, R. Burge, J.M. Schech, and J.M. Pound.
2003. Control of Ixodes scapularis (Acari: Ixodidae) with topical self-application
of permethrin by White-tailed Deer inhabiting NASA, Beltsville, Maryland.
Journal of Vector Ecology 28:117–134.
Sonenshine, D.E. 1979. Ticks of Virginia (Acari: Metastigmata). The insects of Virginia,
No. 13. Virginia Polytechnic Institute and State University Research Bulletin
139, Research Division, Virginia Polytechnic Institute and State University,
Blacksburg, VA.
Sonenshine, D.E. 1993. Biology of Ticks. Volume 2. Oxford University Press, New
York, NY.
Spielman, A., M.L. Wilson, J.E. Levine, and J. Piesman. 1985. Ecology of Ixodes
dammini-borne human babesiosis and Lyme disease. Annual Review of Entomology
30:439–460.
Spielman, A., S.R. Telford III, and R.J. Pollack. 1993. The origins and course of the
present outbreak of Lyme disease. Pp. 83–98, In H.S. Ginsberg (Ed.). Ecology
and Environmental Management of Lyme Disease. Rutgers University Press,
New Brunswick, NJ.
Steere, A.C., S.E. Malawista, J.A. Hardin, S. Ruddy, P.W. Askenase, and W.A. Andiman.
1977. Erythema chronicum migrans and Lyme arthritis: The enlarging
clinical spectrum. Annals of Internal Medicine 86:685–698.
546 Southeastern Naturalist Vol. 9, No. 3
Stromdahl, E.Y., S.R. Evans, J.J. O’Brien, and A.G. Gutierrez. 2001. Prevalence
of infection in ticks submitted to the Human Tick Test Kit Program of the US
Army Center for Health Promotion and Preventive Medicine. Journal of Medical
Entomology 38:67–74.
Stromdahl, E.Y., P.C. Willimason, T.M. Kollars, Jr., S.R. Evans, R K. Barry, M.A.
Vince, and N.A. Dobbs. 2003. Evidence of Borrelia lonestari DNA in Amblyomma
americanum (Acari: Ixodidae) removed from humans. Journal of Clinical
Microbiology 41:5557–5562.
Stromdahl, E.Y., M.A. Vance, P.M. Billingsley, N.A. Dobbs, and P.C. Williamson.
2008. Rickettsia amblyommii infecting Amblyomma americanum larvae. Vector-
Borne and Zoonotic Diseases 8:15–24.
Taft, S.C, M.K. Miller, and S.M. Wright. 2005. Distribution of Borreliae among ticks
collected from eastern states. Vector-borne and Zoonotic Diseases 5:383–389.
Tanner, C.T. 2007. Tick burdens of Peromyscus leucopus and Borrelia infection rates
in Ixodid ticks in a fragmented Virginia landscape. M.Sc. Thesis, Frostburg State
University, Frostburg, MD. 137 pp.
Telford III, S.R., T.N. Mather, G.H. Alder, and A. Spielman. 1990. Short-tailed
Shrews as reservoirs of the agents of Lyme disease and human babesiosis. Journal
of Parasitology 76:681–683.
Varela, A.S., V.A. Moore, and S.E. Little. 2004. Disease agents in Amblyomma americanum
from northeastern Georgia. Journal of Medical Entomology 41:753–759.
Virginia Department of Health (VDH). 1997. Tick-borne diseases in Virginia. Virginia
Department of Health, Richmond, VA. Virginia Epidemiology Bulletin
97(4):1–6.
VDH. 2001. Virginia Department of Health, Richmond, VA. Reportable Disease
Surveillance in Virginia 2002:66–68.
Ward’s Natural Science. No date. Ward’s tick identification and saftey guide. Rochester,
NY.
Wemmer, C., T.H. Kunz, G. Lundie-Jenkins, and W.J. McShea. 1996. Mammalian
sign. Pp. 157–176, In D.E. Wilson, F.R. Cole, J.D. Nichols, R. Rudran, and M.S.
Foster. (Eds.). Measuring and Monitoring Biological Diversity: Standard Methods
for Mammals. Smithsonian Institution Press, Washington, DC.
Wilder, S.M., and D.B. Meikle. 2004. Prevalence of Deer Ticks (Ixodes scapularis)
on White-footed Mice (Peromyscus leucopus) in forest fragments. Journal of
Mammalogy 85:1015–1018.
Wilson, K.R., and D.R. Anderson. 1985. Evaluation of two density estimators of
small-mammal population size. Journal of Mammalogy 66:13–21.
Zar, J.H. 1999. Biostatistical Analysis. 4th Edition. Prentice Hall, Upper Saddle
River, NJ.