Use of Box-beam Bridges as Day Roosts by Mexican
Free-tailed Bats (Tadarida brasiliensis) in Texas
Melissa B. Meierhofer, Hsiao-Hsuan Wang, William E. Grant, John H. Young Jr., Lauren H. Johnston, Lilianna K. Wolf, Jonah W. Evans, Brian L. Pierce, Joseph M. Szewczak, and Michael L. Morrison
Southeastern Naturalist, Volume 17, Issue 4 (2018): 605–615
Full-text pdf (Accessible only to subscribers.To subscribe click here.)
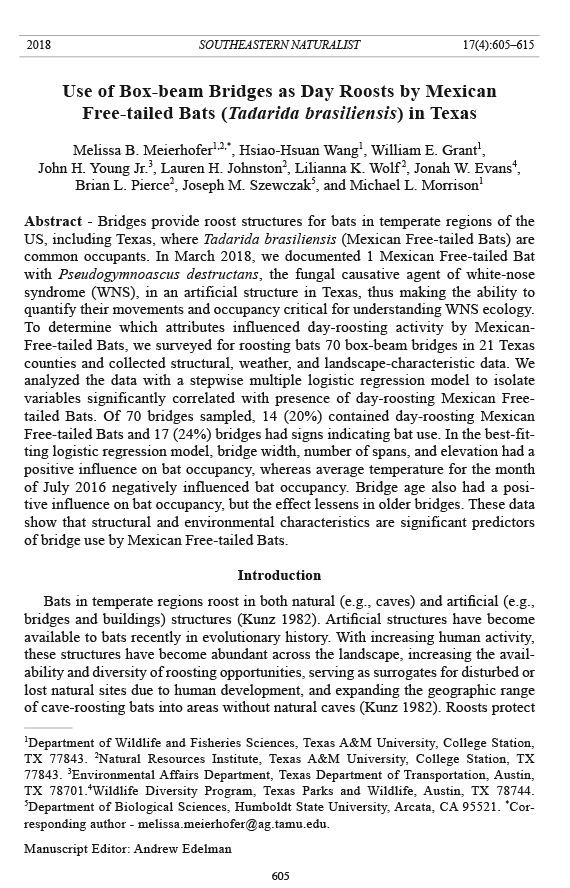
Southeastern Naturalist
605
M.B. Meierhofer et al.
22001188 SOUTHEASTERN NATURALIST 1V7o(4l.) :1670,5 N–6o1. 54
Use of Box-beam Bridges as Day Roosts by Mexican
Free-tailed Bats (Tadarida brasiliensis) in Texas
Melissa B. Meierhofer1,2,*, Hsiao-Hsuan Wang1, William E. Grant1,
John H. Young Jr.3, Lauren H. Johnston2, Lilianna K. Wolf
2, Jonah W. Evans4,
Brian L. Pierce2, Joseph M. Szewczak5, and Michael L. Morrison1
Abstract - Bridges provide roost structures for bats in temperate regions of the
US, including Texas, where Tadarida brasiliensis (Mexican Free-tailed Bats) are
common occupants. In March 2018, we documented 1 Mexican Free-tailed Bat
with Pseudogymnoascus destructans, the fungal causative agent of white-nose
syndrome (WNS), in an artificial structure in Texas, thus making the ability to
quantify their movements and occupancy critical for understanding WNS ecology.
To determine which attributes influenced day-roosting activity by Mexican-
Free-tailed Bats, we surveyed for roosting bats 70 box-beam bridges in 21 Texas
counties and collected structural, weather, and landscape-characteristic data. We
analyzed the data with a stepwise multiple logistic regression model to isolate
variables significantly correlated with presence of day-roosting Mexican Freetailed
Bats. Of 70 bridges sampled, 14 (20%) contained day-roosting Mexican
Free-tailed Bats and 17 (24%) bridges had signs indicating bat use. In the best-fitting
logistic regression model, bridge width, number of spans, and elevation had a
positive influence on bat occupancy, whereas average temperature for the month
of July 2016 negatively influenced bat occupancy. Bridge age also had a positive
influence on bat occupancy, but the effect lessens in older bridges. These data
show that structural and environmental characteristics are significant predictors
of bridge use by Mexican Free-tailed Bats.
Introduction
Bats in temperate regions roost in both natural (e.g., caves) and artificial (e.g.,
bridges and buildings) structures (Kunz 1982). Artificial structures have become
available to bats recently in evolutionary history. With increasing human activity,
these structures have become abundant across the landscape, increasing the availability
and diversity of roosting opportunities, serving as surrogates for disturbed or
lost natural sites due to human development, and expanding the geographic range
of cave-roosting bats into areas without natural caves (Kunz 1982). Roosts protect
1Department of Wildlife and Fisheries Sciences, Texas A&M University, College Station,
TX 77843. 2Natural Resources Institute, Texas A&M University, College Station, TX
77843. 3Environmental Affairs Department, Texas Department of Transportation, Austin,
TX 78701.4Wildlife Diversity Program, Texas Parks and Wildlife, Austin, TX 78744.
5Department of Biological Sciences, Humboldt State University, Arcata, CA 95521. *Corresponding
author - melissa.meierhofer@ag.tamu.edu.
Manuscript Editor: Andrew Edelman
Southeastern Naturalist
M.B. Meierhofer et al.
2018 Vol. 17, No. 4
606
bats during more than half of each day when bats are off the wing and vulnerable
to predation (Altringham 1996, Ferrara and Leberg 2005, Kunz 1982, Kunz and
Lumsden 2003,Tidemann and Flavel 1987). Favorable roosts provide a thermally
stable environment by retaining heat or by preventing the heat loss bats gain by
clustering to conserve energy (Kurta 1985, 1986; Trune and Slobodchikoff 1976),
and where bats receive protection from adverse weather (Vaughan 1987, Vaughan
and Vaughan 1986). Predator avoidance can be accomplished by roosting in narrow,
high spaces (Hutchinson and Lacki 2000, Riskin and Pybus 1998), and darker
locations that make bats less visible (Riskin and Pybus 1998). Furthermore, some
artificial and natural roosts offer conditions that are favorable for mating and rearing
of young, with colonial species requiring that roosts hold numerous individuals
(Kunz 1982).
Bats use concrete bridges as maternity roosts (Perlmeter 1996), night roosts
(Adam and Hayes 2000, Hirschfeld et al. 1977), and day roosts (Bennett et al.
2008, Davis and Cockrum 1963, Felts and Webster 2003, Ferrara and Leberg 2005,
Keeley and Tuttle 1999, Lance et al. 2001). The large thermal mass, relative permanence,
and capacity to shelter large numbers of bats are potential advantages of
bridges as roosts (Trousdale and Beckett 2004). Twenty-four of 45 US bat species
have been documented using bridge structures as day and night roosts, yet only an
estimated 1% of US highway structures provide ideal day-roost conditions (Keeley
and Tuttle 1999). Box-beam bridges with open expansion-joints are used more
than any other kind of bridge as day-roosts as they provide numerous crevices of
suitable width (Adam and Hayes 2000, Davis and Cockrum 1963, Feldhamer et al.
2003, Keeley and Tuttle 1999). Thus, these bridges can also aid in the spread of
Pseudogymnoascus destructans, the causative agent of the bat disease, white-nose
syndrome (WNS) (Lorch et al. 2011). This threat is greatest along the leading edge
of the WNS spread, as these bridges may allow for colony sites to exist in areas that
otherwise cannot sustain colony sites in caves.
Tadarida brasiliensis (I. Geoffroy) (Mexican Free-tailed Bat) is one of the
most abundant insectivorous bats in the Western Hemisphere (McCracken et al.
1994, Wilkins 1989), and the most common bat species in Texas (Ammerman et
al. 2012). They are common bridge-dwellers across the southern US (Keeley and
Tuttle 1999, Krutzsch 1955, Scales and Wilkins 2007). In Texas, large colonies
of Mexican Free-tailed Bats use expansion joints and other crevice features of
highway bridges for their daytime roosts (Keeley and Keeley 2004, Keeley and
Tuttle 1999). Although surveys of bridge use by bats has been conducted in Texas
(Keeley and Keeley 2004, Keeley and Tuttle 1999, Krutzsch 1955), those studies
did not focus on characteristics of day-roost selection of Mexican Free-tailed Bats.
Furthermore, with the recent discovery of P. destructans on a Mexican Free-tailed
Bat in Texas (TPWD 2018) and the rapid expansion of the Mexican Free-tailed Bat
range into areas infected with WNS (McCracken et al. 2018), it is crucial to understand
how these bats are utilizing bridge structures. Therefore, to learn about the
use of box-beam bridges as day roosts, we compared selected attributes of bridges
and environmental characteristics of both occupied and unoccupied sites to identify
Southeastern Naturalist
607
M.B. Meierhofer et al.
2018 Vol. 17, No. 4
variables that may facilitate bat occupancy. This research will provide insight into
conservation efforts of bat colonies occupying bridges, and site selection and design
choices of bridges for future planning and management.
Study Area
We selected 21 eastern and southeastern Texas counties (Bee, Brazos, Burleson,
Dallas, Ellis, Freestone, Goliad, Hardin, Jasper, Jefferson, Kaufman, Kenedy, Liberty,
Live Oak, Madison, Milam, Navarro, Newton, Robertson, San Patricio, Tyler;
Fig. 1) to study use of box-beam bridges by Mexican Free-tailed Bats because they
contained concentrations of bridges with the box-beam design (Fig. 2). Our study
Figure 1. Map depicting the location of the 21 counties in Texas (shaded gray) where we
surveyed box-beam bridges between 2 June 2016 and 5 August 2016 for roosting Mexican
Free-tailed Bats.
Southeastern Naturalist
M.B. Meierhofer et al.
2018 Vol. 17, No. 4
608
area in east and southeast Texas spanned 12 level-II land-use types, which included
commercial and services; cropland and pasture; deciduous forest land; evergreen
forest land; herbaceous rangeland; industrial; mixed-forest land; mixed rangeland;
mixed urban or built-up; other urban or built-up; residential; and transportation,
communications, and utilities (Anderson et al. 1976).
Materials and Methods
We obtained bridge-locality information from the Texas Department of Transportation
(TxDOT) bridge database and identified bridge designs likely to have
attributes, such as expansion joints, suitable for roosting bats (Adam and Hayes
2000, Davis and Cockrum 1963). From the subset of available bridges (n = 2398),
we randomly selected and surveyed 70 concrete, standard box-beam bridges from
2 June 2016 through 5 August 2016.
At each bridge location, we conducted visual surveys between 08:00 and 18:00
for Mexican Free-tailed Bats. A bridge survey consisted of 1 or more investigators
visually scanning the underside of the bridge for bats and/or signs of urine staining
and guano (Keeley and Tuttle 1999). Depending on the height of the bridge, we
used a Black Diamond Icon Headlamp (200 Lumen) or Supernight LED flashlight
(17200 Lumen) to illuminate the crevices to locate bats. Mexican Free-tailed Bats
typically roost in large colonies; thus, we were able to positively identify bridges
where bats were present (Keeley and Tuttle 1999), but we could not accurately
estimate or count the number of bats occupying each bridge surveyed due to the
variation in bridge size and the depth of crevices. Of the bats documented, we could
only identify Mexican Free-tailed Bats with confidence because, to reduce disturbance,
we did not remove bats from bridges.
We gathered structural characteristics (i.e., year of bridge construction, length and
width of bridge, number of spans, construction material) from the TxDOT bridge database.
We considered crevices ideal for roosting bats if they were 0.25–3.0 cm wide
and ≥30-cm deep (Keeley and Tuttle 1999). We documented any obstruction (e.g.,
vegetation) to bat flyways and percentage (%) of the bridge obstructed within 3 m
of the bridge (Keeley and Tuttle 1999). We used bridge localities and US Geological
Survey (USGS) GIS layers to gather additional information on level II land-use (Anderson
et al. 1976) and elevation (meters above sea level) for each site (Table 1). We
also obtained average monthly temperature data for June, July, and August from the
PRISM Climate Group dataset (http://www.prism.oregonstate.edu/). We determined
the distance to water source (e.g., creek, river, stream) at each bridge when water was
Figure 2. Transverse section of a standard box-beam bridge. Examples of a box beam and a
shear-key connection are identified. Figure adapted from Texas Department of Transportation
(TxDOT) blueprint of pre-stressed concrete box-beam spans.
Southeastern Naturalist
609
M.B. Meierhofer et al.
2018 Vol. 17, No. 4
Table 1. Descriptions, values or units of measure, and means or frequencies of landscape features,
bridge characteristics, and climatic conditions evaluated as potential determinants of Mexican Freetailed
Bat occupancy of bridges in Texas. We modeled bridge age as a quadratic variable (bridge age
x bridge age) because of its nonlinearity. We deleted 2 potential determinants (vegetation near bridge
and bridge design) which exhibited no variation among the bridges sampled. All vegetation near
bridges provided open flight paths and design of all bridges was box beam.
Variable Definition; value or unit of measure Mean (min–max) or frequency
Landscape features
Land use Land use for level-2 habitat type;
1—commercial and services 2
2—cropland and pasture 30
3—deciduous forest land 8
4—evergreen forest land 6
5—herbaceous rangeland 2
6—industrial 1
7—mixed forest land 9
8—mixed rangeland 4
9—mixed urban or built-up 1
10—other urban or built-up 2
11—residential 3
12—transportation, communications, and utilities 2
Elevation Elevation; m 89.14 (2–165)
Distance to water Distance to water from the bridge; m 154.01 (0–1457)
Flowing water If water was present at the bridge and flowing;
Yes 39
No 31
Standing water If water was present at the bridge and standing;
Yes 5
No 65
Bridge characteristics
Bridge width Width of bridge; m 20.80 (7.92–32.31)
Bridge length Length of bridge; m 62.33 (12.19–708.66)
Number of spans Number of spans 3.47 (1–47)
Bridge age Years 21.53 (3–87)
Concrete Was the bridge mainly concrete;
Yes 69
No 1
% obstructed Obstructed percentage on either side of bridge by 1.71 (0.00–40.00)
vegetation; %
Obstruction type If the obstruction type was vegetation;
Yes 5
No 65
Ideal crevices If crevices fell within these measurements: 0.25–3 cm
wide, ≥ 30 cm deep;
Yes 63
No 7
Climatic conditions
June 2016 temp. Average temperature for the month of June 2016; C° 27.42 (26.17–28.16)
July 2016 temp. Average temperature for the month of July 2016; C° 29.70 (28.47–30.63)
August 2016 temp. Average temperature for the month of August 2016; C° 28.61 (27.45–29.53)
Southeastern Naturalist
M.B. Meierhofer et al.
2018 Vol. 17, No. 4
610
visibly present. If we could not determine a water source on site, we used GIS layers
containing water features (e.g., rivers, streams, lakes) to obtain distance from the
bridge to the nearest water source. We considered only major water features that we
were confident retained water year-round.
Potential predictors of bridge occupancy
We used landscape features, bridge characteristics, and climatic conditions described
in Table 1 as potential explanatory variables promoting bat occupancy under
bridges during summer months (June, July, and August) in Texas. We evaluated
landscape, weather, and bridge characteristics as determinants of bat occupancy via
stepwise multiple logistic regression (Hosmer et al. 2013). As in ordinary regression,
collinearity among the explanatory variables can cause problems with parameter
estimates and standard errors in multiple logistic regression. To avoid possible collinearity,
we used multiple logistic regression to remove most insignificant terms
with the highest P-value and a large standard error (Gan et al. 2009; Table 1). We then
re-estimated the model until the Akaike information criterion score (AIC) (Akaike
1998) and Bayesian information criterion score (BIC) (Schwarz 1978) could not be
lowered further. For each of the remaining variables, we calculated the estimated
odds ratio which indicated the change in the probability of bat occupancy under
bridges that would result from a 1-unit change in the value of the indicated variable.
We used Hosmer and Lemeshow’s test to check for the model’s overall goodnessof-
fit. We evaluated the reliability and validity of our models as fair (0.50 < AUC ≤
0.75), good (0.75 < AUC ≤ 0.92), very good (0.92 < AUC ≤ 0.97), or excellent (0.97 less than
AUC ≤ 1.00) based on the value of AUC (Hosmer et al. 2013). We conducted all statistical
analyses in SAS 9.4 (SAS Institute Inc. 2013).
Results
We surveyed 70 bridges: 24 in June, 24 in July, and 22 in August 2016. Of the 70
randomly selected box-beam bridges surveyed for presence of Mexican Free-tailed
Bats, 14 (20%) were occupied at the time of survey and 17 (24%) showed signs of
use by bats. We documented 13 occupied bridges in June, 1 occupied bridge in July,
and no occupied bridges in August.
In the final model (L), both AIC and BIC reached their respective minimums
(AIC = 26.20, BIC = 40.09; Table 2) after 11 variables had been removed. Hosmer
and Lemeshow’s goodness-of-fit test indicated no significant difference (P = 0.43)
between observed and model-predicted occupancy values based on the final model
(L). The AUC score of the model was 0.98, which indicated an excellent ability to
discriminate between presence and absence of bats. The overall model classified
91% of the bridges correctly regarding presence and absence of bats.
Results of logistic regression indicated the probability of bat occupancy was
correlated (estimated odds ratios in parentheses) positively with bridge width
(2.4), number of spans (3.3), elevation (1.1), and bridge age x bridge age (1.0)
with gradually weaker influence on older bridges, and correlated negatively
with and average temperature for the month of July (less than 0.001) (Table 3). For
Southeastern Naturalist
611
M.B. Meierhofer et al.
2018 Vol. 17, No. 4
example, a 2-m increase in bridge width would make occupancy 6.6 times more
likely, whereas a 2-y increase in bridge age would make occupancy only 0.776
(1 – 0.112 – 0.112) as likely, after controlling for the other variables.
Discussion
Mexican Free-tailed Bats were more likely to occupy wider box-beam
bridges with a greater number of spans. Bats might select roosting sites at larger
bridges because these structures provide greater protection from predators and increase
roosting potential with more roosting crevices (i.e., availability of crevices
and rough substrate) (Ferrara and Leberg 2005, Keeley and Tuttle 1999, Tidemann
and Flavel 1987), thus increasing the potential for bats that roost in large
congregations, such as the Mexican Free-tailed Bat, to use the bridge as a roost.
Table 2. Results of multiple logistic regression modeling to evaluate landscape and environmental
variables as potential determinants of Mexican Free-tailed Bat occupancy of bridges in Texas. Variables
are listed in the order they were removed; once removed from the model, we did not return
that variable to the model. The minimum value of the Akaike information criterion score (AIC) and
Bayesian Information Criterion score (BIC) is indicated by an asterisk (*).
Variable removed AIC BIC
None 350.35 420.05
Land use 45.35 81.32
June temperature 2016 43.37 77.09
Concrete 41.37 72.85
Standing water 39.37 68.60
% obstructed 37.37 64.35
Ideal vrevices 35.38 60.12
Obstruction type 33.39 55.87
Distance to water 31.52 51.75
Flowing water 29.57 47.55
August temperature 2016 27.60 43.34
Bridge length 26.60* 40.09*
Number of spans 31.24 40.45
Elevation 34.52 41.35
Table 3. Potential determinants of Mexican Free-tailed Bat occupancy of bridges in Texas as indicated
by results of stepwise multiple logistic regression. The estimated odds ratio indicates the change in
the probability of bat occupation under bridges that would result from a 1-unit change in the value of
the indicated variable. For example, a 1-unit increase in the number of spans signifies that occupation
is 3.3 times more likely than before, after controlling for the other variables.
Odds ratio 95% CI
Variable Coefficient SE Odds ratio Lower Upper
Elevation 0.0895 0.0639 1.094 0.965 1.240
Bridge width 0.8854 0.5999 2.424 0.748 7.855
Number of spans 1.1901 0.9647 3.287 0.496 21.778
Bridge age × bridge age 0.0014 0.0010 1.001 0.999 1.003
July temperature 2016 −8.7281 5.2891 less than 0.001 less than 0.001 5.148
Southeastern Naturalist
M.B. Meierhofer et al.
2018 Vol. 17, No. 4
612
Furthermore, larger bridges sustain a diversity of microclimates that provide bats
with thermoregulatory benefits (Ferrara and Leberg 2005). By design, box-beam
bridges have 1.5-in longitudinal gaps between spans (TxDOT 2006). These gaps,
which can be from 2.54–12.7 cm (1.0 to 5.0 in) in length (TxDOT 2006), are crevices
in which bats are found roosting (Keeley and Tuttle 1999).
Our results suggest that higher elevation is a positive predictor of bridge use
by Mexican Free-tailed Bats. Although we surveyed bridges across a breadth of
geography, our elevation range was limited (2–165 m; Table 1) because of our survey-
site selection. According to the TxDOT bridge database, the box-beam bridge
design is used predominantly in eastern Texas where the elevation does not exceed
200 m; across Texas, elevation can range from 0 m (sea level) to 2667 m. This wide
range in elevation may differentially affect the use of box-beam structures across
the landscape. Therefore, our results suggesting a correlation between elevation and
bridge use likely varies by region and may or may not play an important factor in
roost occupancy across the state.
We found a positive relationship between bridge age and bat presence, with
the effect lessening in older bridges. This finding is similar to previous literature
whereby bridges occupied by bats in Florida were significantly older than bridges
without bats (Gore and Studenroth 2005). Reasons for this may include dissimilarity
in roosting opportunities within the adjacent landscape (Trousdale et al. 2008) as
landscape connectivity may influence animal distributions and movements (Henry
et al. 2007). However, Hendricks et al. 2005 reported use of bridges for roosting
was generally unrelated to the landscape within 3 km of the structure. Structurally,
newer bridges may have less roosting opportunities, as older bridges experience
more weathering or heaving vibrations leading to grouting between stones falling
out, increasing overall roosting potential.
We found that monthly temperature for July was a negative predictor of bat
occupancy. Previous research has suggested that day-roosting bats choose roosts
with reduced temperatures, especially during warm weather (Hutchinson and
Lacki 2001, Kerth et al. 2001, Riskin and Pybus 1998, Vonhof and Barclay 1997).
July had the hottest documented ambient temperatures of any month in our study
(Table 1); thus, heat retention may have been greater for those bridges surveyed as
well as natural roosts. We conducted surveys once at each site over 3 months. We
visited each site only once in July (n = 24); bats may have been absent at the time of
our surveys due to other factors (e.g., elevation, geographic location). We suggest
further bridge surveys be conducted with repeat visits to understand how ambient
temperature may influence bridge use seasonally.
Pressure on natural roost sites for bats may increase as the human population
in Texas increases. More infrastructure projects are planned to support this
growth, which could increase the use of bridges by bats as roosting structures
into areas where bats were not previously documented. Furthermore, the use
of bridges by bats is likely helping to expand the distribution of Mexican Freetailed
Bats into areas with natural caves and other bats that are susceptible to
WNS (McCracken et al. 2018). The movement of Mexican Free-tailed Bats into
Southeastern Naturalist
613
M.B. Meierhofer et al.
2018 Vol. 17, No. 4
WNS-positive sites could hasten the spread of disease by moving the fungus into
new, previously uninfected areas, such as the western US or Central America. As
a result, additional species may become at risk of disease, or may become carriers
for the fungus. With this in mind, it is crucial that we place importance on
understanding the structural and environmental factors that influence bat bridgeroosting
preferences. This information could contribute to the understanding on
how WNS may spread with the aid of anthropogenic infrastructure. Moreover,
this information could inform the planning of lasting wildlife-management projects
and infrastructure development, which has a positive effect on environmental
systems. As such, future research should focus on identifying species-specific
factors that drive seasonal bat use of infrastructure.
Acknowledgments
We are grateful to TxDOT for access to bridge sites. We thank TxDOT Environmental
Specialist D. Griffith for her assistance in the field and J. Boleware, Transportation Engineer
Bridge Division, for his help in explaining bridge design. We thank the US Fish and Wildlife
Service in part for supporting our overall research efforts. Funding for this project was
provided through the US Fish and Wildlife Service’s State Wildlife Grant Program (CFDA#
15.611) as administered by the Texas Parks and Wildlife Department.
Literature Cited
Adam, M.D., and J.P. Hayes. 2000. Use of bridges as night roosts by bats in the Oregon
Coast Range. Journal of Mammalogy 81:402–407.
Akaike, H., 1998. Information theory and an extension of the maximum likelihood principle.
Pp. 199–213, In E. Parzen, K. Tanabe, and G. Kitagawa (Eds.). Selected Papers of
Hirotugu Akaike. Springer Series in Statistics, Springer, New York, NY. 432 pp.
Altringham, J.D. 1996. Bats: Biology and Behavior. Oxford University Press, New York,
NY. 262 pp.
Ammerman, L.K., C.L. Hice, and D.J. Schmidly. 2012. Bats of Texas. Texas A&M University
Press, College Station, TX. 162 pp.
Anderson, J.R., E.E. Hardy, J.T. Roach, and R.E. Witmer. 1976. A land-use and land-cover
classification system for use with remote-sensor data. US Geological Survey Professional
Paper 964, Washington, DC. 28 pp.
Bennett, F.M., S.C. Loeb, M.S. Bunch, and W.W. Bowerman. 2008. Use and selection of
bridges as day roosts by Rafinesque’s Big-eared Bats. American Midland Naturalist
2:386–399.
Davis, R., and E.L. Cockrum. 1963. Bridges utilized as day-roosts by bats. Journal of Mammalogy
44:428–430.
Feldhamer, G.A., T.C. Carter, A.T. Morzillo, and E.H. Nicholson. 2003. Use of bridges as
day roosts by bats in southern Illinois. Transactions of the Illinois State Academy of
Science 96:107–112.
Felts, J.W., and W.M.D. Webster. 2003. Use of bridges as daytime roosts by bats in southeastern
North Carolina. Journal of the North Carolina Academy of Science 119:172–178.
Ferrara, F.J., and P.L. Leberg. 2005. Characteristics of positions selected by day-roosting
bats under bridges in Louisiana. Journal of Mammalogy 86:729–735.
Southeastern Naturalist
M.B. Meierhofer et al.
2018 Vol. 17, No. 4
614
Gan, J., J.H. Miller, H. Wang, and J.W. Taylor. 2009. Invasion of Tallow Tree into southern
US forests: Influencing factors and implications for mitigation. Canadian Journal of
Forest Research 39:1346–1356.
Gore, J.A., and K.R. Studenroth Jr. 2005. Status and management of bats roosting in bridges
in Florida. Report to the Florida Department of Transportation. Florida Fish and Wildlife
Conservation Commission, Tallahassee, FL. 64 pp.
Hendricks, P., S. Lenard, C. Currier, and J. Johnson. 2005. Bat use of highway bridges
in south-central Montana. Report to Montana Department of Transportation. Montana
Natural Heritage Program, Helena, MT. 31 pp.
Henry, M., J.-M. Pons, and J.-F. Cosson. 2007. Foraging behavior of a frugivorous bat helps
bridge landscape connectivity and ecological processes in a fragmented rainforest. Journal
of Animal Ecology 76:801–813.
Hirschfeld, J.R., Z.C. Nelson, and W.G. Bradley. 1977. Night-roosting behavior in four species
of desert bats. Southwestern Naturalist 22:427–433.
Hosmer, D.W., Jr., S. Lemeshow, and R.X. Sturdivant. 2013. Applied Logistic Regression.
John Wiley and Sons, New York, NY. 510 pp.
Hutchinson J.T., and M.J. Lacki. 2000. Selection of day roosts by Red Bats in mixed mesophytic
forests. Journal of Wildlife Management 64:87–94.
Hutchinson J.T., and M.J. Lacki. 2001. Possible microclimate benefits of roost-site selection
in the Red Bat, Lasiurus borealis, in mixed mesophytic forests of Kentucky. Canadian
Field-Naturalist 115:205–209.
Keeley, A.T.H., and B.W. Keeley. 2004. The mating system of Tadarida brasiliensis
(Chiroptera: Molossidae) in a large highway bridge colony. Journal of Mammalogy
84:113–119.
Keeley, B.W., and M.D. Tuttle. 1999. Bats in American bridges. Bat Conservation International
Inc., Austin, TX. 41 pp.
Kerth, G., K. Weissmann, and B. Konig. 2001. Day-roost selection in female Bechstein's
Bats (Myotis bechsteinii): A field experiment to determine the influence of roost temperature.
Oecologia 126:1–9.
Krutzsch, P.H. 1955. Observations on the Mexican Free-tailed Bat, Tadarida mexicana.
Journal of Mammalogy 36:236–242.
Kunz, T.H. 1982. Roosting ecology of bats. Pp.1–55, In T.H. Kunz (Ed.). Ecology of Bats.
Plenum Publishing, New York, NY. 427 pp.
Kunz, T.H., and L.F. Lumsden. 2003. Ecology of cavity and foliage-roosting bats. Pp.
3–89, In T.H. Kunz and M.B. Fenton (Eds.). Bat Ecology. University of Chicago Press,
Chicago, IL. 798 pp.
Kurta, T.H. 1985. External insulation available to a non-nesting mammal, the Little Brown
Bat (Myotis lucifugus). Comparative Biochemistry and Physiology 82:413–420.
Kurta, T.H. 1986. Factors affecting the resting and post-flight body temperature of Little
Brown Bats, Myotis lucifugus. Physiological Zoology 59:429–438.
Lance, R.F., B.T. Hardcastle, A. Talley, and P.L. Legerg. 2001. Day-roost selection by
Rafinesque’s Big-eared Bat (Corynorhinus rafinesquii) in Louisiana forests. Journal of
Mammalogy 82:166–172.
Lorch, J.M., C.U. Meteyer, M.J. Behr, J.G. Boyles, P.M. Cryan, A.C. Hicks, A.E. Ballmann,
J.T. Coleman, D.N. Redell, D.M. Reeder, and D.S. Blehert. 2011. Experimental infection
of bats with Geomyces destructans causes white-nose syndrome. Nature 480:376.
McCracken, G.F., M.K. McCracken, and A.T. Vawter. 1994. Genetic structure in migratory
populations of the bat Tadarida brasiliensis mexicana. Journal of Mammalogy
75:500–514.
Southeastern Naturalist
615
M.B. Meierhofer et al.
2018 Vol. 17, No. 4
McCracken, G.F., R.F. Bernard, G.-R. Melquisidec, R. Wolfe, J.J. Krauel, D.N. Jones, A.L.
Russell, and V.A. Brown. 2018. Rapid range expansion of the Brazilian Free-tailed Bat
in the southeastern United States, 2008–2016. Journal of Mammalogy 99:312–320.
Perlmeter, S.I. 1996. Bats and bridges: Patterns of night-roost activity in the Willamette
National Forest. Pp.132–150, In R.M.R. Barclay and R.M. Brigham (Eds.). Bats and
Forests Symposium. Research Branch, British Columbia Ministry of Forests, Victoria,
BC. 302 pp.
Riskin, D.K., and M.J. Pybus. 1998. The use of exposed diurnal roosts in Alberta by the
Little Brown Bat, Myotis lucifugus. Canadian Journal of Zoology 76:767–770.
SAS Institute Inc. 2013. SAS® 9.4 User’s Guide. SAS Institute Inc. Cary, N.C.
Scales, J.A., and K.T. Wilkins. 2007. Seasonality and fidelity in roost use of the Mexican
Free-tailed Bat, Tadarida brasiliensis, in an urban setting. Western North American
Naturalist 67:402–408.
Schwarz, G. 1978. Estimating the dimension of a model. Annals of Statistics 6:461–464.
Shelford, V.E. 1963. The Ecology of North America. University of Illinois Press, Urbana,
IL. 810 pp.
Texas Department of Transportation (TxDOT), Bridge Division Standard. 2006. Prestressed
concrete box-beam spans (Technical Drawing No. SBBS-B20-24). Available
online at http://ftp.dot.state.tx.us/pub/txdot-info/cmd/cserve/standard/bridge/bbstds19.
pdf. Accessed 1 November 2017.
Texas Parks and Wildlife Department (TPWD). 2018. Fungus causing white-nose syndrome
spreads into central Texas. Available online at https://tpwd.texas.gov/newsmedia/
releases/?req=20180404a. Accessed 14 June 2018.
Tidemann, C.R., and S.C. Flavel. 1987. Factors affecting choice of diurnal-roost site by
tree-hole bats (Microchiroptera) in southeastern Australia. Australian Wildlife Research
14: 459–473.
Trousdale, A.W., and D.C. Beckett 2004. Seasonal use of bridges by Rafinesque’s Bigeared
Bat, Corynorhinus rafinesquii, in southern Mississippi. Southeastern Naturalist
3:103–112.
Trousdale, A.W., D.C. Beckett, and S.L. Hammond 2008. Short-term roost fidelity of
Rafinesque’s Big-eared Bat (Corynorhinus rafinesquii) varies with habitat. Journal of
Mammalogy 89:477–484.
Trune, D.R, and C.N. Slobodchikoff. 1976. Social effects of roosting on the metabolism of
the Pallid Bat (Antrozous pallidus). Journal of Mammalogy 57:656–663.
Vaughan, T.A. 1987. Behavioral thermoregulation in the African Yellow-winged Bat. Journal
of Mammalogy 68:376–378.
Vaughan, T.A., and R.P. Vaughan. 1986. Seasonality and the behavior of the African Yellow-
winged Bat. Journal of Mammalogy 67:91–102.
Vonhof, M.J., and R.M.R. Barclay. 1997. Use of tree stumps as roosts by the Western Longeared
Bat. Journal of Wildlife Management 61:674–684.
Wilkins, K.T. 1989. Tadarida brasiliensis. Mammalian Species 331:1–10.