Effects of Growth Rate and Temperature on Metamorphosis
in Eurycea wilderae (Caudata, Plethodontidae,
Hemidactyliinae, Spelerpini; Blue Ridge Two-lined
Salamander)
Christopher K. Beachy
Southeastern Naturalist, Volume 17, Issue 3 (2018): 423–432
Full-text pdf (Accessible only to subscribers.To subscribe click here.)
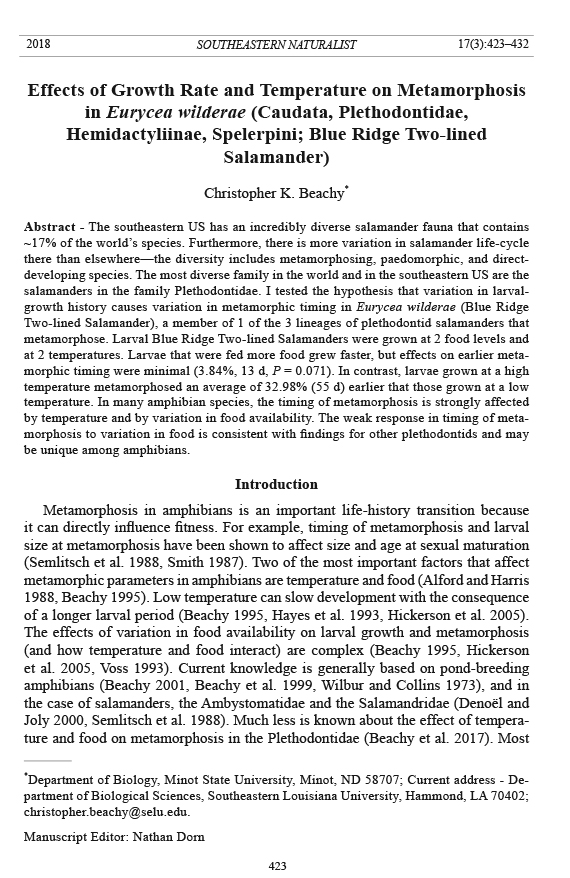
Southeastern Naturalist
423
C.K. Beachy
22001188 SOUTHEASTERN NATURALIST 1V7o(3l.) :1472,3 N–4o3. 23
Effects of Growth Rate and Temperature on Metamorphosis
in Eurycea wilderae (Caudata, Plethodontidae,
Hemidactyliinae, Spelerpini; Blue Ridge Two-lined
Salamander)
Christopher K. Beachy*
Abstract - The southeastern US has an incredibly diverse salamander fauna that contains
~17% of the world’s species. Furthermore, there is more variation in salamander life-cycle
there than elsewhere—the diversity includes metamorphosing, paedomorphic, and directdeveloping
species. The most diverse family in the world and in the southeastern US are the
salamanders in the family Plethodontidae. I tested the hypothesis that variation in larvalgrowth
history causes variation in metamorphic timing in Eurycea wilderae (Blue Ridge
Two-lined Salamander), a member of 1 of the 3 lineages of plethodontid salamanders that
metamorphose. Larval Blue Ridge Two-lined Salamanders were grown at 2 food levels and
at 2 temperatures. Larvae that were fed more food grew faster, but effects on earlier metamorphic
timing were minimal (3.84%, 13 d, P = 0.071). In contrast, larvae grown at a high
temperature metamorphosed an average of 32.98% (55 d) earlier that those grown at a low
temperature. In many amphibian species, the timing of metamorphosis is strongly affected
by temperature and by variation in food availability. The weak response in timing of metamorphosis
to variation in food is consistent with findings for other plethodontids and may
be unique among amphibians.
Introduction
Metamorphosis in amphibians is an important life-history transition because
it can directly influence fitness. For example, timing of metamorphosis and larval
size at metamorphosis have been shown to affect size and age at sexual maturation
(Semlitsch et al. 1988, Smith 1987). Two of the most important factors that affect
metamorphic parameters in amphibians are temperature and food (Alford and Harris
1988, Beachy 1995). Low temperature can slow development with the consequence
of a longer larval period (Beachy 1995, Hayes et al. 1993, Hickerson et al. 2005).
The effects of variation in food availability on larval growth and metamorphosis
(and how temperature and food interact) are complex (Beachy 1995, Hickerson
et al. 2005, Voss 1993). Current knowledge is generally based on pond-breeding
amphibians (Beachy 2001, Beachy et al. 1999, Wilbur and Collins 1973), and in
the case of salamanders, the Ambystomatidae and the Salamandridae (Denoël and
Joly 2000, Semlitsch et al. 1988). Much less is known about the effect of temperature
and food on metamorphosis in the Plethodontidae (Beachy et al. 2017). Most
*Department of Biology, Minot State University, Minot, ND 58707; Current address - Department
of Biological Sciences, Southeastern Louisiana University, Hammond, LA 70402;
christopher.beachy@selu.edu.
Manuscript Editor: Nathan Dorn
Southeastern Naturalist
C.K. Beachy
2018 Vol. 17, No. 3
424
plethodontid species that metamorphose use streams as larval habitat (Wake 1966).
Given that this family is, by far, the most diverse family of salamanders, it seems
essential to understand how food level and temperature influence metamorphosis.
The plethodontid salamander genus Eurycea contains 33 species and includes
taxa that have either a metamorphic life cycle or larval-form paedomorphosis
(Bonett et al. 2014, Ryan and Bruce 1998). In the species that metamorphose, there
can be within- and among-population variation in larval life-history characteristics,
e.g., duration of the larval period and size at metamorphosis (Bruce 1982, Duellman
and Wood 1954, Voss 1993). Intraspecific geographic variation in metamorphic
timing and size in species of Eurycea has been attributed to several factors, e.g.,
temperature, stream order and productivity of the larval habitat (Semlitsch 1980,
Voss 1993). Nearly all work on the larval life history in species of Eurycea has
been restricted to field surveys (e.g., Bruce 1988, Ryan 1998) that established
metamorphic timing and size (but see Aran et al. 2014, Bonnett and Chippindale
2006, McEntire et al. 2014). There are no laboratory growth experiments on larval
Eurycea testing for effects of ecological factors on metamorphic timing or size.
Using experimental manipulations, I examined the effects of temperature and
food on metamorphic timing and size in Eurycea wilderae Dunn (Blue Ridge Twolined
Salamander). The Plethodontidae has 3 lineages with a life cycle that includes
a postembryonic metamorphosis: Hemidactylium scutatum Temminck & Schlegel
in Von Siebold (Four-toed Salamander), the desmognathans, and the spelerpines
(Bonnett 2016). No plethodontid salamander studied in larval-growth experiments
has shown variation in metamorphic timing despite being grown at different rates
via food treatments. These studies have included Desmognathus ocoee Nicholls
(Ocoee Salamander; Beachy 1995), Desmognathus quadramaculatus (Holbrook)
(Blackbelly Salamder; Hickerson et al. 2005), and Four-toed Salamander
(O’Laughlin and Harris 2000). The spelerpine plethodontids (Eurycea, Pseudotriton,
Stereochilus, Gyrinophilus) have not been examined. I tested the hypothesis
that variation in growth history would result in variation in metamorphic timing in
the spelerpine plethodontids by growing larval Blue Ridge Two-lined Salamanders
in the laboratory under simulated ecological conditions. I manipulated food and
temperature and assayed time and size at metamorphosis. This kind of laboratory
growth experiment has been informative in understanding the proximate ecological
conditions responsible for influencing life-history parameters in larval amphibians
(e.g., Alford and Harris 1988, Beachy et al. 1999, Ryan and Semlitsch 2003).
Methods
I grew 37 larval Blue Ridge Two-lined Salamanders (either 9 or 10 per treatment)
in a 2 X 2 experiment in which I manipulated temperature (low and high) and
food level (low and high). I collected larvae on 28 December 2011 from a headwater
seepage in Jackson County, NC (31o15'24''N, 83o09'39''W; datum = WGS84).
Stream temperature during collection was 7 °C. I placed larvae in 2 plastic containers
filled with stream water and leaf litter from the streambed and transported
them in a cooler back to the laboratory in Minot, ND. Upon return to the lab, each
Southeastern Naturalist
425
C.K. Beachy
2018 Vol. 17, No. 3
I placed each larva in an individual plastic box (15 cm x 15 cm x 5 cm) filled with
200 ml of reverse osmosis (RO) water and 25 g of pebbles (placed in a corner of the
box to provide a shelter), and randomly assigned each to 1 of 4 treatment groups.
Larval Blue Ridge Two-lined Salamanders from nearby headwater seeps and 1storder
streams at the collection locality were ~7 months old in December (Bruce
1988, Voss 1993).
Larvae were fed Lumbriculus variegatus (Müller) (California Blackworm).
I obtained the California Blackworms from AquaticFoods.com and stored them
in RO water that was changed daily. I fed larvae different numbers of California
Blackworms. I fed high-food treatment larvae 4 California Blackworms per week
and low-food treatment larvae 1 California Blackworm per week. Larvae in the
low-food treatments always consumed the worm within a 24-h period, whereas
larvae in the high-food treatments often had worms remaining at the next feeding;
in that case, I added only enough worms to bring the total to 4 worms.
I manipulated the temperature by placing larvae in 2 refrigerators (True Company,
O’Fallon, MO) equipped with thermostats (Ranco Company, Itasca, IL) and
glass doors in a room with lighting set to a 12:12 h light:dark cycle. The hightemperature
treatment larvae were kept at 11 °C (± 1 °C ) and the low-temperature
treatment larvae were kept at 7 °C (± 1 °C ). I compensated for spatial variation in 2
ways. First, although the larvae were in separate refrigerators, the maximal distance
between the groups was 1 m. Second, at randomly assigned intervals, I switched the
larvae between refrigerators at the same time that the refrigerator temperature was
reset to the other temperature. By the end of the experiment, all larvae had spent an
approximately equal number of days in each refrigerator.
The experiment was initiated on 4 January 2012, at which time I measured the
snout–vent length (SVL; mm) of all larvae before placing them in the appropriate
temperature treatment and providing their first feeding. The next feeding occurred
on 12 January and every 7 d thereafter. I again measured body size after 49 d and
68 d, and every 14 d thereafter until all animals had completed metamorphosis. On
16 January and every 14 d thereafter, I removed with a net and placed each larva
in a 50-ml beaker of water, rinsed the plastic box and pebbles, refilled the box with
200 ml of fresh RO water that was preconditioned to the appropriate temperature
for each treatment, and replaced the larva into the box and placed it back into the
appropriate temperature treatment.
Beginning on day 54, I raised the refrigerator temperatures 1 °C every 14 d until
temperatures were 16 °C and 12 °C (day 110) in the high- and low-temperature
treatments, respectively. These increases mimicked rising spring temperatures at
the collection locality. I chose those temperatures because they encompassed the
range of natural-temperature variation in headwater streams across the range of
Blue Ridge Two-lined Salamander (e.g., Voss 1993). I doubled the food (i.e., 8
worms and 2 worms at each weekly feeding, respectively) when temperature levels
reached 16 °C and 12 °C.
To measure body size, I placed larvae in a circular glass dish containing a
20-mm ruler and mounted this dish on a pedestal that allowed for the larva to be
photographed from underneath. I downloaded these images to a personal computer
Southeastern Naturalist
C.K. Beachy
2018 Vol. 17, No. 3
426
and employed ImageJ software to determine SVL. As each larva completed metamorphosis
(defined as closure of gill slits and resorption of labial folds), I recorded
the number of days since the initiation of the experiment and metamorphic size
(SVL). Animals were not euthanized at the end of the experiment so that they could
be used in a continuing growth experiment.
I visually inspected growth profiles (Fig. 1) during the experiment to verify that the
food treatments had desired effects, i.e., that larvae grew at different rates. In addition,
I evaluated the effects of temperature and food treatments on growth by analyzing
larval sizes in a 2 x 2 full-factorial MANOVA and ANOVA that analyzed the effects
of temperature and food on the vector consisting of sizes at days 0, 49, 68, and 82. The
analysis did not include body size after day 82 because animals had begun to metamorphose
at day 93. The MANOVA tested the hypothesis that slopes of the growth
profiles were similar (Simms and Burdick 1988), and the series of ANOVAs tested the
hypothesis that body sizes were similar at each measurement date.
I analyzed metamorphic size (SVL) and metamorphic timing (days since initiation
of experiment) in a 2 x 2 full-factorial MANOVA and ANOVA that examined
the effect of food level and temperature on the vector consisting of metamorphic
size and metamorphic timing (MANOVA) and univariate analyses on each variable
(ANOVA). Data on metamorphic timing were inverse-transformed (Alford
and Harris 1988) and data on metamorphic size were log10-transformed (Sokal and
Rohlf 1995).
I conducted all analyses in SPSS version 13. Wilks’ λ was selected as the multivariate
test statistic and type III sums-of-squares were used to generate univariate
mean squares. Significance was assigned using α = 0.05.
Results
Larvae in different treatments were not different in size at the initiation of the
experiment (Table 1, Fig. 1). As predicated by the experiment, larvae fed fewer
worms grew more slowly (Fig. 1). Growth profiles began diverging as soon as food
treatments were initiated and the slope of the growth profiles continued to be different
throughout the experiment (Fig. 1). While food treatment caused larval sizes
to be significantly different by day 82, temperature did not result in significant effects
on larval body size (Table 1). This result suggests that Blue Ridge Two-lined
Table 1. Summary of 2-way analyses on log-transformed larval size prior to initiation of metamorphosis
(i.e., day 93). Multivariate analysis df = 4,30 and univariate df = 1,33 analyses. Values for
analyses are F-statistics. P-values are in parentheses. For the univariate analyses, error mean squares
are 0.003 (day 0), 0.002 (day 49), 0.002 (day 68), and 0.002 (day 82). An asterisk (*) indicates significant
F-tests (P < 0.05).
ANOVA
Source MANOVA Day 0 Day 49 Day 68 Day 82
Temperature 2.45 (0.068) 0.01 (0.924) 0.50 (0.485) 1.40 (0.246) 0.20 (0.661)
Food 9.33 (less than 0.001)* 0.02 (0.892) 1.66 (0.207) 3.22 (0.082) 7.34 (0.011)*
Temperature x Food 0.84 (0.511) 0.10 (0.753) 0.01 (0.920) 0.06 (0.809) 0.41 (0.527)
Southeastern Naturalist
427
C.K. Beachy
2018 Vol. 17, No. 3
Salamander larval growth is more affected by variation in food levels than by temperature
variation.
Larval Blue Ridge Two-lined Salamanders grown at the lower temperature
metamorphosed later (Table 2, Fig. 2). The first metamorph (day 93) was from
Figure 1. Larval
growth profiles of
Blue Ridge Twolined
Salamander in
(A) cold treatment
and (B) warm treatments.
Each point
is mean ± 1 SD.
Lines terminate at
last weighing prior
to initiation of metamorphosis.
Squares
and circles are highfood
treatments and
diamonds and triangles
are low-food
treaments.
Table 2. Summary of multivariate and univariate analyses on log-transformed metamorphic size and
inverse-transformed days to metamorphosis in Blue Ridge Two-lined Salamander. For the analysis of
responses by Blue Ridge Two-lined Salamander, df = 2,32 for the multivariate analysis and df = 1,33
for the univariate analyses. Value for univariate analyses are F-statistics. P-values are in parentheses.
Error mean squares are 0.001 for metamorphic mass and 9.91 x 10-7 for days to metamorphosis. An
asterisk (*) indicates significant F-tests (P < 0.05).
Multivariate statistics Univariate statistics
Source Wilks’ λ F Metamorphic mass Days to metamorphosis
Temperature 0.24 49.70 (less than 0.001)* 6.20 (0.018)* 66.15 (less than 0.001)*
Food 0.67 7.91 (0.002)* 16.18 ( less than 0.001)* 3.49 (0.071)
Temperature x Food 0.99 0.18 (0.840) 0.37 (0.548) 0.08 (0.782)
Southeastern Naturalist
C.K. Beachy
2018 Vol. 17, No. 3
428
the high-temperature–high-food treatment, and all high-temperature larvae had
metamorphosed by day 138. Only 1 larva from the low-temperature treatment
metamorphosed before day 138 (at day 109), and the rest metamorphosed by day
254. Larvae grown at low temperature delayed metamorphosis, they grew for
a longer period of time and thus attained larger metamorphic size (7.2%) than
larvae at high temperature (Table 2, Fig. 2). In contrast, the food treatment (i.e.,
growth variation) only strongly affected size at metamorphosis (Table 2, Fig. 2)
but had a relatively weak, and non-significant (P = 0.071) effect on timing of
metamorphosis. Larvae in the high-food treatments were 12.6% larger at the time
of metamorphosis compared to those in the low-food treatments (Fig. 2). The interaction
term was non-significant for both responses (Table 2).
Discussion
The Plethodontidae is, by far, the most diverse family of salamanders, and this
family exhibits a wide variety of life cycles: metamorphosis, paedomorphosis,
and direct development (Dunn 1926, Wake 1966). The diversity of life histories
in the plethodontids and, in particular, the Spelerpini, has been influenced by
shifts in developmental timing (Bonett 2016, Bonett et al. 2014, Ryan and Bruce
1998). Indeed, Bonnet (2016) has suggested that species diversity in Eurycea
is a consequence of life-history diversity, especially in the evolution between
paedomorphic and metamorphic life cycles. Phylogenetic evidence suggests that
ancestral plethodontids were direct-developers and that subsequent shifts to a
Figure 2. Summary of metamorphic responses of Blue Ridge Two-lined Salamander to
temperature and food treatments. (A) Size and metamorphosis in mm SVL. (B) Days from
initation of experiment to metamorphosis. Bars are means ± 1 SD. Statistical analysis is
summarized in Table 1.
Southeastern Naturalist
429
C.K. Beachy
2018 Vol. 17, No. 3
metamorphic life cycle were cases of metamorphic deceleration (Bonnet 2016,
Bonnet et al. 2014). Thus, understanding the proximate causes of shifts in metamorphic
timing in the Spelerpini seem important in addressing the fundamental
question of why this family is so diverse when compared to the rest of salamander
families (Beachy et al. 2017).
The effect of temperature is clearly important in affecting metamorphic timing
in amphibians. Previous work has shown that growing larval amphibians at low
temperature reliably slows development and delays metamorphosis (Beachy 1995,
Clarkson and Beachy 2015, Hayes et al. 1993, Hickerson et al. 2005, Leips and
Travis 1994, Uhlenhuth 1919) and this was the case in my study. There is a clear
connection between these lab studies and natural variation in larval life-histories:
cold habitats support larvae that metamorphose later (and often larger) than warmer
habitats (Berven and Gill 1983, Smith-Gill and Berven 1979, Voss 1993). In some
cases, low temperature appears to be the proximate cause in inducing paedomorphosis
(Eagleson 1976).
Although the effect of temperature on amphibian development is clear, the relationship
of growth to metamorphic timing is still not well understood. Studies that
directly manipulate larval growth by using food treatments are most informative
when individuals are isolated from one another, thus avoiding confounding effects
of interacting larvae (Alford and Harris 1988). Surprisingly, there are still only ~30
studies across all species in which individual larvae were fed different amounts
of food, and metamorphic timing was analyzed (Beachy et al. 2017). It is always
desirable to begin growth experiments with embryos and hatchlings, but this is not
always possible, e.g., not all species have egg clutches that are easy to find. In any
case, and in contrast to predictions of fixed-rate models (e.g., Travis 1984), these
studies show that larvae fed different amounts of food metamorphose at different
dates, even when the variation in feeding is imposed relatively late in development,
i.e., as much as 50–67% of the days needed to complete larval development (Alford
and Harris 1988, Beachy et al. 1999, Beachy 2001). Although it is conceivable that
beginning this experiment with larvae that are 7 months old (i.e., at 47–58% of the
larval period; Bruce 1982, 1988; Voss 1993) limits the interpretation of these results,
every experiment (except for those on larval plethodontids) that shifted food
availability within this period resulted in variation in metamorphic timing (Beachy
et al. 2017).
In studies where growth was directly manipulated using individual larvae,
the result of food treatment was generally that larvae grown rapidly metamorphosed
earlier (e.g., Hensley 1993, Leips and Travis 1994, Vaissi and Sharifi
2016), although there are cases in the frog genus Spea where rapidly growing
larvae metamorphose later than larvae that are growing slowly (Denver et
al. 1998, Newman 1994). In all lab studies (exclusive of those on plethodontid
larvae) where food treatments were imposed, timing of metamorphosis was affected
(e.g., Alford and Harris 1988, Beachy 2001, Hensley 1993, Leips and
Travis 1994). These studies include insects, crustaceans, frogs, and salamanders
(Beachy et al. 2017), and the effects of growth-rate variation were increases or
Southeastern Naturalist
C.K. Beachy
2018 Vol. 17, No. 3
430
decreases in mean metamorphic timing that varied from 13% to 66%. In contrast,
even if the P-value (0.071; Table 2) for the effect of food on metamorphic timing
on metamorphosis in Blue Ridge Two-lined Salamander were considered significant,
the differences between mean metamorphic timing were only 5.48% (at
low temperature) and 2.19% (at high temperature). In the 4 studies that manipulated
food levels in larval plethodontids—Beachy (1995), Hickerson et al. (2005),
O’Laughlin and Harris (2000), and this study)—changes in mean metamorphic
timing varied from less than 0.1% to 5.48% and were not statistically significant.
It is remarkable that growth-induced plasticity in metamorphic timing is weak
(or non-existent) in the plethodontid salamanders, given that it is such a strong
determinant of variation in metamorphic timing in other groups of amphibians. It
is tenable that the phenotypic plasticity so often inferred to have adaptive value in
high-productivity and ephemeral habitats may entail costs that are not economical
in the permanent, low-productivity larval habitats experienced by most plethodontid
larvae (Beachy et al. 2017). Perhaps this loss of plasticity is important in the
evolution of both direct-development and paedomorphosis in the Plethodontidae
(Beachy et al. 2017). In contrast, if the ancestral life-cycle of plethodontids is a
direct-developing one (Bonett et. 2014), then this lack of plasticity in metamorphic
timing is likely a developmental constraint inherited from direct-developing ancestors
where plasticity in metamorphosis was disadvantageous.
Acknowledgments
This project was supported by grants from the National Center for Research Resources
(5P20RR016471-12) and the National Institute of General Medical Sciences (8 P20
GM103442-12) from the National Institutes of Health. The study was approved by the
Minot State University Institutional Animal Care and Use Committee (protocol 2012-01).
Thanks to W. Beachy and H. Beachy for assistance in collection and to F. Coyle for permission
to collect larvae from his creek and for providing creek-temperature data.
Literature Cited
Alford, R.A., and R.N. Harris. 1988. Effects of larval-growth history on anuran metamorphosis.
American Naturalist 131:91–106.
Aran, R.P., M.A. Steffen, S.D. Martin, O.I. Lopez, and R.M. Bonett. 2014. Reduced effects
of thyroid hormone on gene expression and metamorphosis in a paedomorphic plethodontid
salamander. Journal of Experimental Zoology (Molecular and Developmental
Evolution) 322B:294–303.
Beachy, C.K. 1995. Effects of larval growth history on metamorphosis in a stream-dwelling
salamander (Desmognathus ochrophaeus). Journal of Herpetology 29:375–382.
Beachy, C.K. 2001. Effects of growth history and thyroxine-induced acceleration on metamorphic
timing in the toad Bufo americanus (Amphibia, Anura). Copeia 2001:829–834.
Beachy, C.K., M. Reyes, and T. Surges. 1999. Effects of developmental and growth history
on metamorphosis in the Gray Treefrog, Hyla versicolor (Amphibia, Anura). Journal of
Experimental Zoology 283:522–530.
Beachy, C.K, T.J. Ryan, and R.M. Bonett. 2017. How metamorphosis is different in
plethodontids: Larval life-history perspective on life-cycle evolution. Herpetologica
73:252–258.
Southeastern Naturalist
431
C.K. Beachy
2018 Vol. 17, No. 3
Berven, K.A., and D.E. Gill. 1983. Interpreting geographic variation in life-history traits.
American Zoologist 23:85–97.
Bonett, R.M. 2016. An integrative endocrine model for the evolution of developmental
timing and life history of plethodontids and other salamanders. Copeia 104:209–221.
Bonett, R.M., and P.T. Chippindale. 2006. Streambed microstructure predicts evolution of
development and life-history mode in the plethodontid salamander Eurycea tynerensis.
BMC Biology 4:6.
Bonett, R.M., M.A. Steffen, and G.A. Robinson. 2014. Heterochrony repolarized: A phylogenetic
analysis of developmental timing in plethodontid salamanders. EvoDevo 5:27.
Bruce, R.C. 1982. Larval periods and metamorphosis in two species of salamanders of the
genus Eurycea. Copeia 1982:117–127.
Bruce, R.C. 1988. An ecological life table for the salamander Eurycea wilderae. Copeia
1988:15–26.
Clarkson, P.M, and C.K. Beachy. 2015. Induction of metamorphosis causes differences in
sex-specific allocation patterns in axolotls (Ambystoma mexicanum) that have different
growth histories. Journal of Herpetology 49:621–626.
Denoël, M., and P. Joly. 2000. Neoteny and progenesis as two heterochronic processes
involved in paedomorphosis in Triturus alpestris (Amphibia: Caudata). Proceedings of
the Royal Society of London B 267:1481–1485.
Denver, R.J., N. Mirhadi, and M. Phillips. 1998. Adaptive plasticity in amphibian metamorphosis:
Response of Scaphiopus hammondii tadpoles to habitat desiccation. Ecology
79:1859–1972.
Duellman, W.E., and J.T. Wood. 1954. Size and growth of the Two-lined Salamander, Eurycea
bislineata rivicola. Copeia 1954:92–96.
Dunn, E.R. 1926. The Salamanders of the Family Plethodontidae. Smith College,
Northampton, MA. 446 pp.
Eagleson, G.W. 1976. A comparison of the life histories and growth patterns of populations
of the salamander Ambystoma gracile (Baird) from permanent low-altitude and montane
lakes. Canadian Journal of Zoology 54:2098–2111.
Hayes, T.B., R. Chan, and P. Licht. 1993. Interactions of temperature and steroids on larval
growth, development, and metamorphosis in a toad (Bufo boreas). Journal of Experimental
Zoology 266:206–215.
Hensley, F.R. 1993. Ontogenetic loss of phenotypic plasticity of age at metamorphosis in
tadpoles. Ecology 74:2405–2412.
Hickerson, C.M., E.L. Barker, and C.K. Beachy. 2005. Determinants of metamorphic timing
in the Black-bellied Salamander, Desmognathus quadramaculatus. Southeastern
Naturalist 4:33–50.
Leips, J., and J. Travis. 1994. Metamorphic responses to changing food levels in two species
of hylid frogs. Ecology 75:1345–1356.
McEntire, K.P., T.W. Pierson, and J.C. Maerz. 2014. Eurycea cirrigera (Southern Twolined
Salamander) paedomorphosis. Herpetological Review 45:473.
Newman, R.A. 1994. Effects of changing density and food level on metamorphosis of a
desert amphibian, Scaphiopus couchii. Ecology 75:1085–1096.
O’Laughlin, B.E., and R.N. Harris. 2000. Models of metamorphic timing: An experimental
evaluation with the pond-dwelling salamander Hemidactylium scutatum (Caudata:
Plethodontidae). Oecologia 124:343–350.
Ryan, T.J. 1998. Larval life-history and abundance of a rare salamander, Eurycea junaluska.
Journal of Herpetology 32:10–17.
Southeastern Naturalist
C.K. Beachy
2018 Vol. 17, No. 3
432
Ryan, T.J., and R.C. Bruce. 1998. Life-history evolution and adaptive radiation of hemidactyliine
salamanders. Pp. 303–326, In R.C. Bruce, R.G. Jaeger, and L.D. Houck (Eds.).
The Biology of Plethodontid Salamanders. Plenum Press, New York, NY. 485 pp.
Ryan T.J., and R.D. Semlitsch. 2003. Growth and the expression of alternative life cycles in
the salamander Ambystoma talpoideum (Caudata: Ambystomatidae). Biological Journal
of the Linnaean Society 80:639–646.
Semlitsch, R.D. 1980. Growth and metamorphosis of larval dwarf salamanders (Eurycea
quadridigitata). Herpetologica 36:138–140.
Semlitsch, R.D., D.E. Scott, and J.H.K. Pechmann. 1988. Time and size at metamorphosis
related to adult fitness in the salamander Ambystoma talpoideum. Ecology 69:184–192.
Simms, E.L., and D.S. Burdick. 1988. Profile analysis of variance as a tool for analyzing
correlated responses in experimental ecology. Biometry Journal 30:229–242.
Smith, D.C. 1987. Adult recruitment in chorus frogs: Effects of size and date at metamorphosis.
Ecology 68:344–350.
Smith-Gill, S.J., and K. Berven. 1979. Predicting amphibian metamorphosis. American
Naturalist 113:563–585.
Sokal, R.R., and F.J. Rohlf. 1995. Biometry, 3rd Edition. W.H. Freeman, New York, NY.
887 pp.
Travis, J. 1984. Anuran size at metamorphosis: Experimental test of a model based on intraspecific
competition. Ecology 35:423–432.
Uhlenhuth, E. 1919. Relation between metamorphosis and other developmental phenomena
in amphibians. Journal of General Physiology 1:525–544.
Vaissi, S., and M. Sharifi. 2016. Changes in food availability mediate the effects of temperature
on growth, metamorphosis, and survival in endangered Yellow Spotted Newt:
Implications for captive breeding programs. Biologica 71:441–451.
Voss, S.R. 1993. Relationship between stream order and length of larval period in the salamander
Eurycea wilderae. Copeia 1993:736–742.
Wake, D.B. 1966. Comparative osteology and evolution of the lungless salamanders, family
Plethodontidae. Memoirs of the Southern California Academy of Science 4:1–111.
Wilbur, H.M., and J.P. Collins. 1973. Ecological aspects of amphibian metamorphosis. Science
182:1305–1314.