Myxomycete Assemblages Recovered from Experimental
Grass and Forb Microhabitats Placed Out and Then
Recollected in the Tallgrass Prairie Preserve, OK
Adam W. Rollins and Steven L. Stephenson
Southeastern Naturalist, Volume 15, Issue 4 (2016): 681–688
Full-text pdf (Accessible only to subscribers.To subscribe click here.)
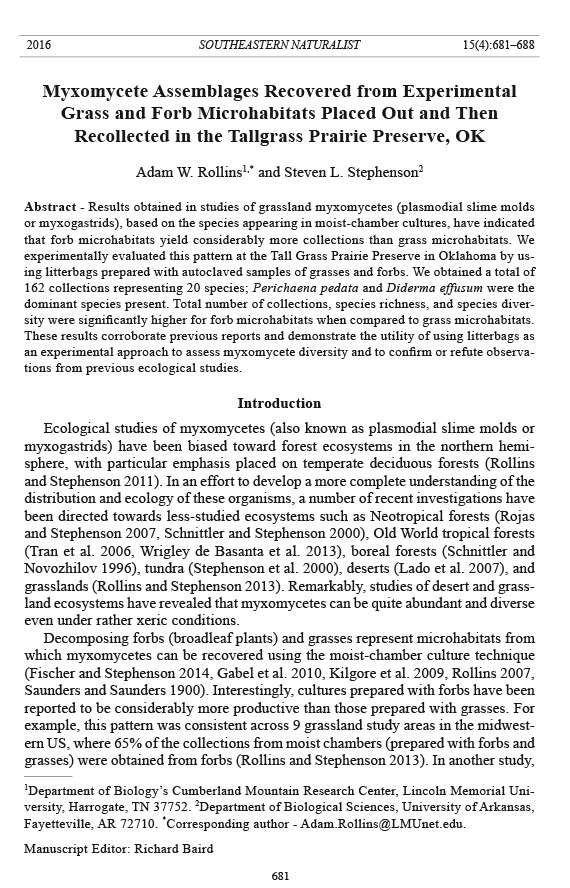
Southeastern Naturalist
681
A.W. Rollins and S.L. Stephenson
22001166 SOUTHEASTERN NATURALIST 1V5o(4l.) :1658,1 N–6o8. 84
Myxomycete Assemblages Recovered from Experimental
Grass and Forb Microhabitats Placed Out and Then
Recollected in the Tallgrass Prairie Preserve, OK
Adam W. Rollins1,* and Steven L. Stephenson2
Abstract - Results obtained in studies of grassland myxomycetes (plasmodial slime molds
or myxogastrids), based on the species appearing in moist-chamber cultures, have indicated
that forb microhabitats yield considerably more collections than grass microhabitats. We
experimentally evaluated this pattern at the Tall Grass Prairie Preserve in Oklahoma by using
litterbags prepared with autoclaved samples of grasses and forbs. We obtained a total of
162 collections representing 20 species; Perichaena pedata and Diderma effusum were the
dominant species present. Total number of collections, species richness, and species diversity
were significantly higher for forb microhabitats when compared to grass microhabitats.
These results corroborate previous reports and demonstrate the utility of using litterbags as
an experimental approach to assess myxomycete diversity and to confirm or refute observations
from previous ecological studies.
Introduction
Ecological studies of myxomycetes (also known as plasmodial slime molds or
myxogastrids) have been biased toward forest ecosystems in the northern hemisphere,
with particular emphasis placed on temperate deciduous forests (Rollins
and Stephenson 2011). In an effort to develop a more complete understanding of the
distribution and ecology of these organisms, a number of recent investigations have
been directed towards less-studied ecosystems such as Neotropical forests (Rojas
and Stephenson 2007, Schnittler and Stephenson 2000), Old World tropical forests
(Tran et al. 2006, Wrigley de Basanta et al. 2013), boreal forests (Schnittler and
Novozhilov 1996), tundra (Stephenson et al. 2000), deserts (Lado et al. 2007), and
grasslands (Rollins and Stephenson 2013). Remarkably, studies of desert and grassland
ecosystems have revealed that myxomycetes can be quite abundant and diverse
even under rather xeric conditions.
Decomposing forbs (broadleaf plants) and grasses represent microhabitats from
which myxomycetes can be recovered using the moist-chamber culture technique
(Fischer and Stephenson 2014, Gabel et al. 2010, Kilgore et al. 2009, Rollins 2007,
Saunders and Saunders 1900). Interestingly, cultures prepared with forbs have been
reported to be considerably more productive than those prepared with grasses. For
example, this pattern was consistent across 9 grassland study areas in the midwestern
US, where 65% of the collections from moist chambers (prepared with forbs and
grasses) were obtained from forbs (Rollins and Stephenson 2013). In another study,
1Department of Biology’s Cumberland Mountain Research Center, Lincoln Memorial University,
Harrogate, TN 37752. 2Department of Biological Sciences, University of Arkansas,
Fayetteville, AR 72710. *Corresponding author - Adam.Rollins@LMUnet.edu.
Manuscript Editor: Richard Baird
Southeastern Naturalist
A.W. Rollins and S.L. Stephenson
2016 Vol. 15, No. 4
682
which used a combination of field surveys and moist-chamber cultures, Gabel et al.
(2010) recovered only 9% of their collections from grass litter, further suggesting
that grass microhabitats represent a poor substrate for many myxomycetes.
It seems that fundamental ecological differences exist between decomposing
grasses and forbs that affect their suitability as substrates for myxomycetes. These
differences may be related to a wide range of variables such as the respective rates
of decomposition for forbs and grasses, quantitative differences in the nutrient
pools associated with each microhabitat, or differences in the types and/or abundances
of the microbes myxomycetes utilize as a food resource.
Moore and Spiegel (1995, 2000) examined the distribution patterns of protosteloid
amoebae (often referred to as protostelids) by placing autoclaved sections
of wheat straw into a series of study areas. After a period of time, the straws were
collected, placed in laboratory culture, and monitored for the occurrence of protosteloid
amoebae. The results obtained through the use of this technique were
comparable to results obtained by culturing naturally occurring substrates. The
objective of the present study was to assess the differences in the assemblages of
myxomycetes associated with the 2 microhabitats represented by the sterilized litter
of forbs and grasses placed in nylon-mesh bags and subjected to field conditions in
the Tallgrass Prairie Preserve in Oklahoma.
Study Site
The study was carried out in the 15,432-ha Tallgrass Prairie Preserve
(36°51'40.17"N, 96°25'15.93"W), located in the Osage Plains section of the Central
Lowland physiographic province of Oklahoma. The underlying geology is characterized
by chert-bearing limestone and shale, with rocky soils that have prevented
extensive farming, making this area the largest remaining tract of undisturbed tall
grassland in North America. The study site has an elevation of 322 m and receives
an average of 1118 mm annual precipitation. The dominant vegetation consists of
Andropogon gerardi Vitman (Big Bluestem), Sorghastrum nutans (L.) Nash (Indian
Grass), Panicum virgatum L. (Switchgrass), and Schizachyrium scoparium
(Michx.) Nash var. scoparium (Little Bluestem) (Coppedge and Shaw 1998). The
preserve is grazed by Bos taurus L. (Cattle) and Bison bison Hamilton Smith (American
Bison), and prescribed burning is utilized at various scales and frequencies.
Methods
In May 2007, we collected samples of the dead and decomposing litter of forbs
and grasses from the Tallgrass Prairie Preserve study site. We placed the samples
in brown paper bags and transported them to the laboratory at the University of
Arkansas, where we autoclaved all samples and stored them in plastic freezer-bags.
We constructed from a nylon-mesh hardware cloth (1-mm2 openings) fifty-four 12
cm x 15 cm litterbags, similar in design to those used in litter-decomposition studies
(e.g., Bradford et al. 2002). We filled 27 bags each with grass litter or forb litter.
We closed the bags with a glue sealant and numbered each with fabric paint and a
Southeastern Naturalist
683
A.W. Rollins and S.L. Stephenson
2016 Vol. 15, No. 4
permanent black marker, autoclave-sterilized, and stored them in plastic freezerbags
for transport to the field-study site.
In June 2007, we placed the bags in a grid pattern with alternating rows of grass
litter and forb litter at the Tallgrass Prairie Preserve study site. Each row contained
9 bags, with each bag separated, in all directions by 1 m. We secured every bag
to the ground with a metal landscaping staple (Fig. 1). The litterbags represented
experimental implants of the grass-ground and forb-ground microhabitats. At 3, 6,
and 9 months, we relocated and collected a randomly predetermined subset consisting
of 12 litterbags (6 forb and 6 grass). We took the bags to the laboratory at the
University of Arkansas and allowed them to air dry for ~1 week. We prepared 3
moist-chamber cultures from each litterbag as described by Stephenson and Stempen
(1994); thus, 18 forb-litter and 18-grass litter cultures were prepared during
each collection period, resulting in an overall total of 108 cultures prepared from
the implanted litterbags. As a control, we prepared an additional 20 cultures (10
forb and 10 grass) with sterilized substrate material that had never been placed out
in the field.
We ranked species of myxomycetes detected in the moist-chamber cultures as A
= abundant (>3% of total collections), C = common (>1.5%–3%), O = occasional
(>0.5%–1.5%), and R = rare (≤0.5%) as described by Stephenson et al. (1993). We
pooled values obtained from each triplicate set for subsequent analysis. We calculated
relative abundance, diversity indices, and percent similarity as described in
Rollins et al. (2010). We employed the nonparametric Mann-Whitney U test and the
parametric unpaired 2-sample t-test to evaluate the statistical significance between
Figure 1. (A) The general aspect of the Tallgrass Prairie Preserve study site, and (B) a litterbag
secured to the ground by a metal landscaping staple.
Southeastern Naturalist
A.W. Rollins and S.L. Stephenson
2016 Vol. 15, No. 4
684
treatments at each time interval (i.e., 3, 6, 9 months). The data often failed to approximate
a normal distribution and the sample size for each pooled treatment was
small (n = 6) (i.e., 3, 6, 9 months); thus, statistical significance was recognized only
when both tests indicated this outcome. We applied diversity t-tests according to
Poole (1974) and conducted all data analyses using the Past 3.06 statistical software
package (Hammer et al. 2001). Myxomycete nomenclature follows the morphospecies
concepts of Lado (2016).
Results
The 20 control cultures did not produce a single plasmodium or fruiting body.
We obtained 162 collections representing 20 species from the moist-chamber
cultures prepared with material from the recovered litterbags (Table 1). The
forb microhabitat was the most productive—116 collections assigned to 18 species
representing 72% of the total number of collections. As assessed by percent
similarity, the myxomycete assemblages recorded from the 2 microhabitats were
rather dissimilar (Table 1). Perichaena pedata and Diderma effusum were the most
frequently recovered species, accounting for 54% of the total collections obtained
in the study (Table 2). These 2 species were common to both microhabitats, but
represented 84% of the collections obtained from grasses, where no other species
had a relative abundance of ≥5% .
Forb microhabitats were characterized by a significantly greater (a) number of
collections, (b) species richness, and (c) species diversity when compared to grass
microhabitats (Fig. 2). Species richness and diversity did not vary appreciably
between the 3- and 6-month samples, but there was a noticeable decrease for the
9-month samples. The recovery of Arcyria afroalpina and an unidentified Lepidoderma
(both from forbs) was particularly noteworthy.
Discussion
The results of the present study support previous reports that forb microhabitats
are considerably more productive for myxomycetes than are grass microhabitats
(e.g., Rollins and Stephenson 2013). The mechanism(s) resulting in this consistent
and recurring pattern are currently unknown. However, the impacts of differential
decomposition rates, nutrient quality and availability, and the types and/or abundances
of food organisms available represent areas that warrant further study.
Table 1. Summary data for each microhabitat sampled in each collection interval and pooled data for
the entire study.
3 Months 6 Months 9 Months Forbs Grasses All
Forb Grass Forb Grass Forb Grass pooled pooled pooled
Number of collections 42 24 50 19 24 3 116 46 162
% of collections 63.6 36.4 72.5 27.5 88.9 11.1 71.6 28.4 100
Species richness 11 5 13 5 8 2 18 8 20
Percent similarity 57.1 38.5 37.5 52.2 -
Southeastern Naturalist
685
A.W. Rollins and S.L. Stephenson
2016 Vol. 15, No. 4
Prior to carrying out the study, we hypothesized that species diversity and
richness would increase with time for each of the 2 types of microhabitats. The
forb-litter samples displayed a slight increase in species richness and diversity
between the 3- and 6-month time intervals, whereas the values recorded for the
grass-litter samples remained relatively unchanged. However, both species richness
and diversity decreased for both sets of samples at the 9-month interval.
Although this result was unexpected, it is noteworthy that during the week prior to
the 9-month collection interval, the study area experienced torrential downpours
and flooding. It seems possible that these conditions could have washed away or
otherwise depressed the numbers of propagules (i.e., myxamoebae and microcysts)
present at the time we collected the samples. These data might provide some insight
into the short-term effects of extreme rain events on myxomycete dynamics at a
given locality. This possibility is particularly interesting because it was proposed
(Alexopoulos 1970) that the tropics might be characterized by a lower richness and
diversity of myxomycetes due to the impact of rainfall as a disturbance factor.
The assemblages at both substrates were most similar at the 3-month sampling
period (PS = 57.1). We obtained this result because the 2 most-common species,
Table 2. The overall relative abundance (RA) and ranking of the species of myxomycetes obtained
from the experimentally implanted forb and grass microhabitats at the Tallgrass Prairie Preserve.
Perichaena pedata and Diderma effusum were the dominant species, accounting for 54% of all collections
obtained in the entire study. Ranks: A = abundant (>3% of total collections), C = common
(>1.5%-3%), O = occasional (>0.5%-1.5%), and R = rare (≤0.5%) as described by Stephenson et al.
1993. A dash indicates that the species was not present.
Forb Grass Substrates Rank
Species Forb RA Rank Grass RA Rank pooled pooled
Perichaena pedata (Lister & G. Lister) G. 25.9 A 54.3 A 34.0 A
Lister
Diderma effusum (Schwein.) Morgan 16.4 A 30.4 A 20.4 A
Arcyria cinerea (Bull.) Pers. 16.4 A 0.0 - 11.7 A
Lamproderma scintillans (Berk. & Broome) 14.7 A 4.3 A 11.7 A
Morgan
Didymium difforme (Pers.) S.F. Gray 4.3 A 2.2 C 3.7 A
Perichaena depressa Libert 5.2 A 0.0 - 3.7 A
Didymium ochroideum G. Lister 1.7 C 2.2 C 1.9 C
Perichaena chrysosperma (Currey) Lister 1.7 C 2.2 C 1.9 C
Physarum cinereum (Batsch) Pers. 2.6 C 0.0 - 1.9 C
Arcyria afroalpina Rammeloo 1.7 C 0.0 - 1.2 O
Hemitrichia pardina (Minakata) B. Ing 1.7 C 0.0 - 1.2 O
Lepidoderma sp. A 1.7 C 0.0 - 1.2 O
Perichaena corticalis (Batsch) Rostaf. 1.7 C 0.0 - 1.2 O
Didymium anellus Morgan 0.0 - 2.2 C 0.6 O
Didymium sp. A 0.0 - 2.2 C 0.6 O
Licea operculata (Wingate) G.W. Martin 0.9 O 0.0 - 0.6 O
Perichaena liceoides Rostaf. 0.9 O 0.0 - 0.6 O
Perichaena vermicularis (Schwein.) Rostaf. 0.9 O 0.0 - 0.6 O
Physarum notabile T. Macbr. 0.9 O 0.0 - 0.6 O
Trichia contorta (Ditmar) Rostaf. 0.9 O 0.0 - 0.6 O
Total 100.0 100.0 100.0
Southeastern Naturalist
A.W. Rollins and S.L. Stephenson
2016 Vol. 15, No. 4
686
Diderma effusum and Perichaena pedata, colonized both substrates at a relatively
equal frequency. However, after 6 months, the frequency of recovery of these 2
species decreased substantially for grasses, while they remained consistent on
forbs, and forbs also continued to gain myxomycete species. It seems that the mostcommon
species in the area quickly colonized the sampled substrates, but only
persisted on the most suitable substrate represented by forbs. Throughout the course
of the study, the assemblages of species associated with forb litter remained fairly
similar, while the assemblages associated with grass litter were rather dissimilar.
These results suggest that the assemblages of myxomycetes associated with forbs
may be more stable, whereas those associated with grasses may be more transient.
The possible stability associated with a particular microhabitat warrants further investigation.
As mentioned earlier, this situation may be related to the fundamental
biotic and abiotic differences that exist between the 2 microhabitats.
We obtained what appears to represent an undescribed species of Lepidoderma
from the forb samples in the current study as well as from the samples processed
during a previous study carried out in the Sheyenne National Grasslands (Rollins
Figure 2. Comparisons of (A) mean number of collections per sample, (B) mean species
richness per sample, (C) Shannon diversity index, and (D) Simpson’s index of diversity
for forb microhabitats (shaded bars) and grass microhabitats (open bars) at each collection
interval and all intervals pooled. Forb microhabitats had significantly greater values for all
comparisons with the exception of the 9-month Simpson index of diversity (D). Error bars
represent standard error of the mean (A and B) and standard deviation (C and D). We set
an α value of 0.05 for all tests. * indicates a significant difference between microhabitats.
Southeastern Naturalist
687
A.W. Rollins and S.L. Stephenson
2016 Vol. 15, No. 4
and Stephenson 2013). These collections require further study in order to confirm
their taxonomic status. It is also noteworthy that the 2 collections of Arcyria afroalpina
represent a rare species typically recorded only from tropical regions (Lado
and Wrigley de Basanta 2008, Rojas et al. 2010).
Numerous descriptive studies have focused on myxomycete occurrence patterns
across various biomes and microhabitats. However, very little work has been done
that utilizes experimental manipulation to evaluate fundamental ecological hypotheses.
Myxomycetes are abundant in virtually every terrestrial microhabitat and
produce macroscopic fruiting bodies that are easily collected and cultured; thus, they
represent excellent model organisms to study microbial ecology. Although myxomycete
studies have been biased toward forested ecosystems, grassland ecosystems
represent a simpler system in which to utilize myxomycetes to address broad ecological
questions. Grasslands hold great potential to study the role of microorganisms
in ecosystem function, biogeochemical processes, and functional traits.
The results presented herein are drawn from 54 independent samples placed
across a single location covering an area less than 54 m2 and provide a limited
ability to make large-scale inferences across the grassland biome. The ultimate
significance of our study is that it demonstrated the utility of using litterbags as
an experimental approach for assessing myxomycete diversity. This technique has
great promise as a tool to support or refute observations made as part of previous
ecological studies. Because myxomycetes are relatively easy to isolate from samples
of dead plant material under laboratory conditions, a similar approach could
be employed to investigate a number of other aspects of the distribution and ecology
of this group of organisms. For example, observational studies have resulted
in (a) the classification of myxomycetes into ecological groups such as lignicolous,
corticolous, litter-inhabiting, and coprophilous; and (b) reported changes in species
assemblages with changes in elevation. Our approach could be utilized to evaluate
these hypotheses and many others that have been based on correlations from observational
studies.
Acknowledgments
The research described herein was supported by grants from the National Science
Foundation (DEB-0316284) and Prairie Biotic Research, Inc. We thank George Ndiritu and
Bryon Jones for help with collecting some of the samples.
Literature Cited
Alexopoulos, C.J. 1970. Rainforest myxomycetes. Pp. F21–F23, In H.T. Odum (Ed). A
Tropical Rain Forest. Atomic Energy Commission, Washington, DC. 1600 pp.
Bradford, M.A., G.M. Tordoff, T. Eggers, T. Hefin Jones, and J.E. Newington. 2002. Microbiota,
fauna, and mesh-size interactions in litter decomposition. Oikos 99:317–323.
Coppedge, B.R., and J.H. Shaw. 1998. Bison grazing patterns on seasonally burned tallgrass
prairie. Journal of Range Management 51:258–264.
Fischer, H.E., and S.L. Stephenson. 2014. Myxomycetes associated with two types of
grassland in northwest Arkansas. Österreichische Zeitschrift für Pilzkunde 23:125–129.
Southeastern Naturalist
A.W. Rollins and S.L. Stephenson
2016 Vol. 15, No. 4
688
Gabel, A., E. Ebbert, M. Gabel, and L. Zierer. 2010. A survey of myxomycetes from the
Black Hills of South Dakota and the Bear Lodge Mountains of Wyoming. Proceedings
of the South Dakota Academy of Science 89:45–67.
Hammer, Ø., D.A.T. Harper, and P.D. Ryan. 2001. PAST: Paleontological statistics software
package for education and data analysis. Palaeontologica Electronica 4:9.
Kilgore, C.M., H.W. Keller, and J.S. Ely. 2009. Aerial reproductive structures of vascular
plants as a microhabitat for myxomycetes. Mycologia 101:305–319.
Lado, C. 2016. An online nomenclatural information system of Eumycetozoa. Available
online at http://www.nomen.eumycetozoa.com. Accessed on 15 March 2016.
Lado, C., and D. Wrigley de Basanta. 2008. A review of Neotropical myxomycetes (1828–
2008). Anales del Jardin Botanico de Madrid 65:211–254.
Lado, C., A. Estrada-Torres, and S.L. Stephenson. 2007. Myxomycetes collected in the first
phase of a north–south transect of Chile. Fungal Diversity 25:81–101.
Moore, D., and F.W. Spiegel. 1995. A new technique for sampling protostelids. Mycologia
87:414–418.
Moore, D.L., and F.W. Spiegel. 2000. Microhabitat distribution of protostelids in temperate
habitats in northwestern Arkansas. Canadian Journal of Botany 78:985–994.
Poole, R.W. 1974. An Introduction to Quantitative Ecology. McGraw-Hill, New York, NY.
532 pp.
Rojas, C., and S.L. Stephenson. 2007. Distribution and ecology of myxomycetes in the
high-elevation oak forests of Cerro Bellavista, Costa Rica. Mycologia 99:534–543.
Rollins, A.W. 2007. The myxomycetes of Thunder Basin National Grassland. Castilleja
26:8–9.
Rollins, A.W., and S.L. Stephenson. 2011. Global distribution and ecology of myxomycetes.
Current Topics in Plant Biology 12:1–14.
Rollins, A.W., and S.L. Stephenson. 2013. Myxomycetes associated with grasslands of the
western central United States. Fungal Diversity 59:147–158.
Rollins, A.W., J.C. Landolt, and S.L. Stephenson. 2010. Dictyostelid cellular slime
molds associated with grasslands of the central and western United States. Mycologia
102:996–1003.
Saunders, J.E., and E. Saunders. 1900. The habitats of the Mycetozoa. Transactions of the
Hertfordshire Natural History Society 10:169–172.
Schnittler, M., and Y.K. Novozhilov. 1996. The myxomycetes of boreal woodlands in Russian
northern Karelia: A preliminary report. Karstenia 36:19–40.
Schnittler, M., and S.L. Stephenson. 2000. Myxomycete biodiversity in four different forest
types in Costa Rica. Mycologia 92:626–637.
Stephenson, S.L., and H. Stempen. 1994. Myxomycetes: A Handbook of Slime Molds.
Timber Press, Portland, OR. 183 pp.
Stephenson, S.L., I. Kalyanasundaram, and T.N. Lakhanpal. 1993. A comparative biogeographical
study of myxomycetes in the mid-Appalachians of eastern North America and
two regions of India. Journal of Biogeography 20:645–657.
Stephenson, S.L., Y.K. Novozhilov, and M. Schnittler. 2000. Distribution and ecology of
myxomycetes in high-latitude regions of the Northern Hemisphere. Journal of Biogeography
27:741–754.
Tran, H.T.M., S.L. Stephenson, K.D. Hyde, and O. Mongkolporn. 2006. Distribution and
occurrence of myxomycetes in tropical forests of northern Thailand. Fungal Diversity
22:227–242.
Wrigley de Basanta, D., C. Lado, A. Estrada-Torres, and S.L. Stephenson. 2013. Biodiversity
studies of myxomycetes in Madagascar. Fungal Diversity 59:55–83.